10. Cellular Senescence
Cellular senescence is a biological phenomenon in which cells lose their ability to divide and function properly due to various factors, including DNA damage, stress, or reaching the maximum number of divisions (replicative senescence). Senescent cells undergo distinct changes in gene expression and morphology, often becoming larger and developing a characteristic senescence-associated secretory phenotype (SASP). This phenotype involves the secretion of various molecules, including inflammatory cytokines, growth factors, and proteases, which can impact neighboring cells and the tissue microenvironment.
Importance in Biological Systems
Cellular senescence plays a dual role in biological systems. On one hand, it serves as a mechanism to prevent damaged or potentially cancerous cells from proliferating, which helps maintain tissue integrity and suppress tumor formation. On the other hand, the accumulation of senescent cells over time contributes to aging and age-related diseases. Senescent cells can promote inflammation, tissue dysfunction, and impair the regenerative capacity of tissues. Cellular senescence has an important role in the developmental processes that shape organismal form and function. During embryonic development, senescence helps regulate tissue morphogenesis by eliminating cells that are not needed or have the potential to disrupt proper development. This process is called developmental senescence. It ensures that organs and tissues form correctly and that unnecessary structures are removed. Senescence also contributes to tissue repair and regeneration during development. For example, during limb development, some cells undergo senescence to guide the precise patterning of fingers or toes.
How does cellular senescence contribute to tissue aging and development?
The effects of cellular senescence can be both beneficial and detrimental, depending on the context and timing. Here's how cellular senescence influences tissue aging and development:
Tissue Aging
Accumulation of Senescent Cells: Over time, senescent cells can accumulate in tissues due to various factors such as exposure to stress, DNA damage, and chronic inflammation. These cells have lost their ability to divide and can persist in tissues, contributing to age-related changes.
Senescence-Associated Secretory Phenotype (SASP): Senescent cells undergo changes in gene expression, leading to the secretion of inflammatory cytokines, growth factors, and other molecules. This SASP can promote chronic inflammation, disrupt tissue homeostasis, and contribute to age-related diseases.
Tissue Dysfunction: The presence of senescent cells and their SASP can impair tissue function by promoting fibrosis (scar tissue formation), altering the extracellular matrix, and interfering with the regenerative capacity of stem cells. This can lead to decreased tissue functionality and an overall decline in organ performance.
Loss of Regenerative Potential: Senescent cells can negatively affect the regenerative capacity of tissues. They can impair the activity of nearby stem cells and hinder tissue repair and renewal, leading to decreased resilience against damage and aging.
Development
Tissue Patterning and Morphogenesis: During embryonic development, cellular senescence plays a role in tissue patterning and morphogenesis. Some cells undergo senescence to help sculpt and refine developing structures. This process ensures proper formation of organs and body parts.
Clearance of Unwanted Cells: Senescence can help eliminate cells that are no longer needed during development. This ensures the removal of structures or cell populations that could interfere with proper tissue formation.
Guidance of Tissue Regeneration: In certain developmental contexts, senescent cells can guide tissue regeneration. For instance, during limb development, senescent cells may contribute to precise finger or toe patterning.
What are the molecular triggers and pathways that lead to cellular senescence?
Cellular senescence is a complex process regulated by various molecular triggers and pathways. Several factors can induce senescence, including DNA damage, telomere attrition, oncogene activation, and oxidative stress.
DNA Damage: DNA damage activates the DNA damage response (DDR) pathway, involving proteins like ATM, ATR, and p53. Activation of p53 can lead to cell cycle arrest and senescence through transcriptional regulation of genes involved in cell cycle control and senescence.
Telomere Attrition: Telomeres, protective caps at the ends of chromosomes, shorten with each cell division. Critically short telomeres trigger a DNA damage response, leading to p53 activation and cell cycle arrest.
Oncogene Activation: Certain oncogenes (genes that promote cell growth) can induce senescence when activated. The RAS oncogene pathway is known to contribute to senescence induction.
Oxidative Stress: High levels of reactive oxygen species (ROS) can cause cellular damage. ROS-induced DNA damage and activation of DDR can lead to senescence.
Epigenetic Changes: Alterations in epigenetic marks, such as DNA methylation and histone modifications, can drive senescence.
Senescence-associated heterochromatin foci (SAHF) form due to epigenetic changes and contribute to gene silencing.
Mitochondrial Dysfunction: Impaired mitochondrial function and increased ROS production can lead to senescence. Mitochondrial dysfunction contributes to the aging process and senescence.
Inflammatory Signaling: The senescence-associated secretory phenotype (SASP) involves the secretion of inflammatory cytokines, chemokines, and growth factors. SASP promotes inflammation and can affect neighboring cells and the tissue microenvironment.
Senescence-Associated Kinase Pathways: Various kinases, such as mTOR (mammalian target of rapamycin) and p38 MAPK (mitogen-activated protein kinase), are implicated in senescence regulation.
Autophagy Deficiency: Impaired autophagy (cellular recycling process) can lead to accumulation of damaged cellular components and senescence.
Non-Coding RNAs: MicroRNAs and long non-coding RNAs can regulate senescence-associated pathways and gene expression. The interplay of these triggers and pathways contributes to the intricate regulation of cellular senescence.
Depending on the context and combination of factors, cells can enter a state of senescence, which can have both protective and detrimental effects on the organism. Senescence serves as a mechanism to prevent damaged cells from proliferating and potentially becoming cancerous, but the accumulation of senescent cells can contribute to aging and age-related diseases.
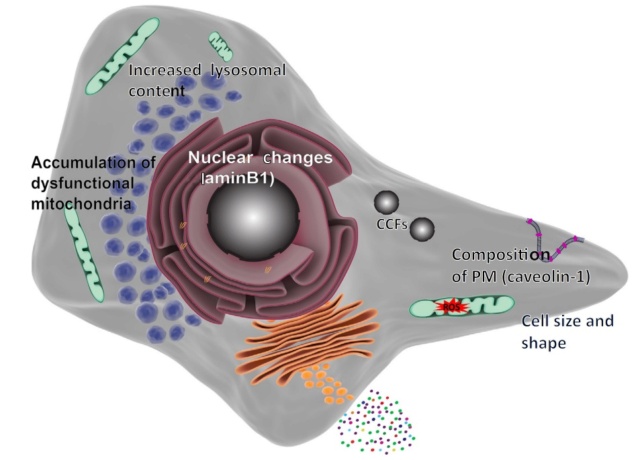
Hallmarks of Senescence Morphological Alterations. The molecular pathways of senescence result in morphological alterations. Senescent cells are enlarged and have an irregular shape; their nuclear integrity is compromised
due to the loss of laminB1, which also leads to the appearance of cytoplasmic chromatin fragments (CCFs); they have an increased lysosomal content, which is often detected as high b-galactosidase activity; they have large but dysfunctional mitochondria that produce high levels of reactive oxygen species (ROS); and their plasma membrane (PM) changes its composition (for instance, upregulating caveolin-1). 1
Appearance of Cellular Senescence in the evolutionary timeline
Cellular senescence has likely emerged relatively early in the evolution of complex multicellular organisms. While the exact timing and origins of cellular senescence are not fully elucidated, evidence suggests that its emergence is intertwined with the evolution of multicellularity and complex life forms. Here's a general overview of how cellular senescence might have appeared in the evolutionary timeline:
Origin of Simple Multicellularity: Around 1 to 2 billion years ago, simple multicellular organisms, such as algae and early colonial organisms, would have started to evolve. As cells came together to form tissues, mechanisms to prevent uncontrolled growth and maintain tissue integrity would have emerged. These mechanisms would be considered rudimentary forms of what we now understand as cellular senescence.
Emergence of More Complex Multicellular Life: About 600 million years ago, more complex multicellular life forms would have began to evolve, including early metazoans (animals). With the development of more specialized cell types and tissues, the need to regulate cell division and prevent runaway growth would have become more critical.
Balancing Senescence and Regeneration: Throughout evolution, organisms would have needed to strike a balance between the protective effects of cellular senescence (preventing cancer and tissue overgrowth) and the necessity for tissue regeneration and repair. Cellular senescence would have evolved as a way to prevent damaged cells from propagating in multicellular organisms, contributing to the overall fitness of the organism.
Variability in Senescence Mechanisms: Different organisms would have developed variations of senescence mechanisms based on their specific needs and environmental pressures. Senescence would have evolved independently in different lineages, leading to diverse regulatory pathways and outcomes.
Evolution of Longevity and Aging: As organisms would have evolved, longer lifespans would have become advantageous for various reasons, such as increased reproductive opportunities and the development of more complex behaviors. Cellular senescence would have become intertwined with aging processes, contributing to age-related changes and the development of age-related diseases.
De Novo Genetic Information necessary to instantiate Cellular Senescence
Creating a hypothetical process for generating new genetic information and introducing it in the correct sequence to establish the mechanisms of cellular senescence involves conceptualizing a scenario where new functional elements are added to an existing genetic system. Here's a simplified outline of what such a process might entail:
DNA Sequences Encoding Senescence Factors: New DNA sequences would need to originate that encode for specific proteins and regulatory elements involved in cellular senescence.
These sequences should include instructions for the synthesis of proteins that regulate cell cycle arrest, senescence-associated secretory phenotype (SASP) components, and factors that induce the senescent state.
Regulatory Elements for Expression: Alongside the new DNA sequences, regulatory elements such as promoters, enhancers, and transcription factor binding sites would be necessary.
These regulatory elements would ensure that the genes involved in senescence are expressed at the appropriate times and in the correct cell types.
Protein Interaction Networks: New information would have to emerge to establish protein-protein interaction networks that allow the newly synthesized senescence-associated proteins to function together in a coordinated manner.
This would involve the de novo generation of functional protein domains, motifs, and interaction interfaces.
Signaling Pathways and Feedback Loops: Information would need to originate to establish signaling pathways that sense cellular stress, DNA damage, or other triggers of senescence.
Feedback loops and crosstalk mechanisms would be required to fine-tune the response and duration of the senescence process.
Epigenetic Changes and Chromatin Remodeling: The process would necessitate the emergence of mechanisms that induce epigenetic changes leading to altered gene expression patterns associated with senescence.
Histone modifications, DNA methylation patterns, and chromatin remodeling would need to be established.
Establishment of Senescence Phenotype: The correct sequence of events would need to be orchestrated, starting with the activation of senescence-inducing factors, followed by cell cycle arrest, morphological changes, and the secretion of SASP components.
Coordination with Developmental Programs: The hypothetical process should ensure that the induction of cellular senescence is coordinated with other developmental processes to maintain tissue integrity and overall functionality.
In this speculative scenario, the challenge lies in generating entirely new genetic information de novo, including sequences encoding proteins, regulatory elements, and complex functional networks.
This description simplifies a highly complex and multifaceted biological process and emphasizes the requirement for coordinated and functional information to establish the mechanisms of cellular senescence without invoking evolutionary mechanisms. It's important to note that real biological systems are much more intricate and involve a dynamic interplay of genetics, epigenetics, and molecular networks.
Manufacturing codes and languages that would have to emerge and be employed to instantiate Cellular Senescence
Regulatory Sequences and Transcription Factors: New regulatory sequences, such as enhancers and silencers, would need to emerge de novo. Transcription factors specific to senescence-related genes would need to originate, recognizing these regulatory sequences and activating the genes involved in cellular senescence.
Protein Folding and Modification Codes: A new set of protein folding codes and chaperone molecules would need to be established to ensure that the newly synthesized senescence-associated proteins are correctly folded into their functional three-dimensional structures. Post-translational modification codes, like phosphorylation and acetylation, would need to be created to regulate protein activities.
Signaling Networks and Cascade Codes: Signaling cascades would have to be introduced, involving the de novo generation of receptor-ligand interactions and intracellular signaling codes. Feedback loops and response thresholds would need to be established to coordinate the cellular response to senescence triggers.
Secretory Pathway Codes: Manufacturing codes would be required to assemble the components of the secretory pathway that allow for the packaging and secretion of senescence-associated factors, including inflammatory cytokines and growth factors.
Epigenetic Modification Machinery: Enzymatic codes for DNA methylation and histone modification would need to be introduced to establish the specific epigenetic changes associated with cellular senescence.
Cellular Localization Signals: New signals and codes directing the correct localization of senescence-associated proteins within the cell would need to be instantiated.
Morphological Changes Coordination: Codes would be necessary to coordinate the morphological changes associated with cellular senescence, such as cell enlargement and alterations in organelle distribution.
Crosstalk and Feedback Mechanisms: Complex codes for crosstalk and feedback mechanisms would have to be introduced to ensure the proper coordination of cellular senescence with other cellular processes and environmental cues.
Timed Expression Codes: Codes for temporal regulation of gene expression and protein activity would need to be established to ensure that senescence-associated processes occur at the appropriate times during an organism's life cycle.
In this speculative scenario, the focus is on the non-genetic manufacturing aspects required to transition from an organism lacking cellular senescence to one with a fully developed senescence process. The challenge here lies in conceiving a hypothetical process where intricate codes and languages are instantiated and coordinated to create the mechanisms of cellular senescence, beyond the genetic information previously outlined. The actual biological processes involve a highly sophisticated interplay of molecular interactions, signaling networks, and cellular machineries.
Epigenetic Regulatory Mechanisms necessary to be instantiated for Cellular Senescence
The hypothetical development of cellular senescence from scratch would require intricate epigenetic regulations to establish and maintain the process. Several systems would need to collaborate to ensure the proper instantiation and balance of these regulations:
Epigenetic Regulations
DNA Methylation: A system of DNA methyltransferases would need to be created to introduce DNA methylation marks at specific regulatory regions. Methylation can silence genes involved in inhibiting senescence or activate genes promoting senescence.
Histone Modifications: Enzymatic complexes would be required to introduce histone modifications like H3K9me3 and H4K20me3 associated with gene silencing and heterochromatin formation. These modifications can impact gene expression profiles central to senescence.
Chromatin Remodeling: An ATP-dependent chromatin remodeling system would be necessary to restructure chromatin and provide accessibility to regulatory elements, influencing senescence-associated gene expression.
Non-Coding RNAs: Systems for producing and processing non-coding RNAs, such as microRNAs and long non-coding RNAs, would need to emerge to regulate gene expression involved in senescence.
Collaborating Systems
Cell Signaling Pathways: Intracellular signaling networks would operate in conjunction with epigenetic regulation to detect stress, DNA damage, or other senescence triggers. These pathways would integrate signals and activate downstream effectors that modulate epigenetic marks.
Cell Cycle Machinery: The cell cycle control machinery, including cyclins and cyclin-dependent kinases, would interact with epigenetic regulators to establish cell cycle arrest during senescence.
DNA Repair Systems: DNA repair mechanisms would work in collaboration with epigenetic regulators to ensure the accurate repair of DNA damage, which can influence the activation of senescence pathways.
Inflammatory Signaling: Systems for sensing and responding to inflammatory cues would interact with epigenetic regulators, shaping the senescence-associated secretory phenotype (SASP).
Feedback and Crosstalk
Feedback loops between epigenetic modifications, gene expression, and signaling pathways would ensure the proper progression and termination of cellular senescence. Crosstalk mechanisms between different epigenetic modifications and regulatory systems would help maintain the balance between cellular senescence and other cellular processes, such as cell proliferation and survival. In this speculative scenario, the establishment and operation of epigenetic regulations for cellular senescence involve the orchestration of multiple complex systems. The collaboration between these systems is essential for fine-tuning the activation, maintenance, and coordination of cellular senescence within the broader cellular context.
Signaling Pathways necessary to create, and maintain Cellular Senescence
The emergence of cellular senescence from scratch would require the establishment of intricate signaling pathways that are interconnected, interdependent, and capable of crosstalk with each other and other biological systems:
p53 Pathway
The p53 pathway would need to be established to sense DNA damage, cellular stress, or oncogenic activation. p53 activation leads to transcriptional upregulation of genes involved in cell cycle arrest and senescence. This pathway interacts with the DNA repair machinery to ensure accurate DNA damage response. Crosstalk with other pathways, such as the mTOR pathway, influences the decision between cell cycle arrest and continued proliferation.
mTOR Pathway
The mTOR pathway would need to integrate nutrient and growth factor signals. Inhibition of mTOR can induce senescence through effects on metabolism and autophagy. Interacts with the p53 pathway to modulate senescence outcome based on cellular context.
Rb Pathway
The Rb pathway would need to control the cell cycle by inhibiting E2F transcription factors. Rb pathway dysfunction can lead to senescence. Crosstalk with other pathways, including p53 and mTOR, influences the decision between senescence and proliferation.
MAPK Pathways
MAPK pathways, including ERK and p38, would need to integrate stress and growth factor signals. Activation of p38 MAPK can trigger senescence through regulation of p53 and other downstream effectors. Crosstalk between MAPK pathways and the p53 pathway can influence the decision to undergo senescence.
TGF-β Pathway
The TGF-β pathway would need to be established to regulate cell growth, differentiation, and senescence. Activation of TGF-β can induce cell cycle arrest and senescence. Interacts with other pathways to modulate senescence in response to external cues.
NF-κB Pathway
The NF-κB pathway would need to be involved in sensing inflammation and oxidative stress. NF-κB activation leads to the transcription of genes associated with the senescence-associated secretory phenotype (SASP).
Crosstalk with other pathways, such as p53 and MAPK, influences the coordination between inflammation and senescence.
Interconnectedness and Crosstalk
These pathways are interconnected, with molecules and signals shared between them. Crosstalk occurs through direct interactions, shared transcriptional targets, and feedback loops. The balance between these pathways determines the decision between senescence, proliferation, and other cellular responses.
Interdependence with Other Systems
Signaling pathways crosstalk not only with each other but also with other biological systems. They interact with epigenetic regulation, DNA repair, metabolic networks, and cellular machinery involved in senescence.
Together, these interactions ensure the integration of senescence signals with broader cellular functions and responses. In this speculative scenario, the emergence of cellular senescence requires the intricate establishment and coordination of signaling pathways that are interconnected, interdependent, and capable of crosstalk. These pathways collaboratively govern the decision-making processes that lead to the initiation, maintenance, and regulation of cellular senescence within the context of broader biological systems.
Regulatory codes necessary for maintenance and operation of Cellular Senescence
The establishment, maintenance, and operation of cellular senescence would necessitate the instantiation of intricate regulatory codes and languages that orchestrate the complex processes involved:
Epigenetic Regulatory Codes
DNA Methylation Patterns: Specific DNA methylation marks would need to be established at regulatory regions to silence or activate genes involved in cellular senescence.
Histone Modification Codes: Codes for histone modifications, such as H3K9me3 and H4K20me3, would need to be instantiated to influence gene expression profiles during senescence.
Chromatin Remodeling Instructions: Codes would be required to direct ATP-dependent chromatin remodeling complexes to modulate chromatin accessibility.
Transcriptional Regulatory Languages
Promoter and Enhancer Codes: Regulatory sequences like promoters and enhancers would need to be established to drive gene expression of senescence-related factors.
Transcription Factor Binding Instructions: Binding sites for transcription factors like those associated with p53 and other senescence-inducing proteins would need to be instantiated.
Signaling Integration and Interpretation
Signaling Code Interpretation: Cellular signaling networks would need codes to interpret inputs from DNA damage, oxidative stress, inflammation, and other triggers of senescence.
Activation and Inhibition Codes: Codes for activation and inhibition of specific signaling pathways would determine the overall cellular response to senescence-inducing cues.
Protein Interaction and Modification Instructions
Protein Interaction Domains: Specific protein interaction domains would be needed to enable the assembly of senescence-associated protein complexes.
Post-Translational Modification Codes: Codes for post-translational modifications like phosphorylation, acetylation, and ubiquitination would influence protein function and stability.
Feedback and Threshold Codes
Feedback Loop Codes: Codes for positive and negative feedback loops would help regulate the progression and termination of cellular senescence.
Threshold Detection Instructions: Codes would need to be established to detect certain thresholds of DNA damage or other senescence-inducing cues.
Temporal and Spatial Coordination Languages
Temporal Regulation Codes: Instructions for temporal regulation would ensure that cellular senescence is initiated and maintained at appropriate times during an organism's life cycle.
Spatial Localization Codes: Codes for spatial localization would direct senescence-related processes to specific cellular compartments.
Secretory Phenotype Code
SASP Regulation Codes: Codes would be necessary to regulate the expression and secretion of components of the senescence-associated secretory phenotype (SASP).
In this speculative scenario, the regulatory codes and languages required for the maintenance and operation of cellular senescence encompass diverse aspects of gene expression, signaling integration, protein interactions, and feedback mechanisms. The orchestration of these codes ensures the intricate and coordinated execution of cellular senescence within the broader cellular context.
How did the mechanisms for cellular senescence supposedly evolve to balance tissue homeostasis and longevity in different species?
The supposed evolution of mechanisms for cellular senescence and their role in balancing tissue homeostasis and longevity in different species is a complex topic that involves a combination of genetic, environmental, and selective pressures. While the exact evolutionary pathways are not fully elucidated, researchers propose several mechanisms and theories to explain how cellular senescence could have evolved to contribute to these balances:
Antagonistic Pleiotropy Theory: This theory suggests that certain genes or mechanisms that confer benefits early in life but have detrimental effects later in life would have been favored by natural selection. In the context of cellular senescence, genes that promote growth and development early in life would also contribute to cellular damage and senescence later in life. Such genes would be selected for their positive effects on reproduction and fitness, even though they lead to aging-related effects.
Trade-Offs between Growth and Longevity: Evolutionary trade-offs between allocating resources for growth and reproduction versus maintenance and longevity would influence the emergence of senescence mechanisms. Species that allocate more resources toward growth and reproduction would have shorter lifespans due to increased cellular damage and senescence, whereas species that prioritize maintenance would have longer lifespans.
Species-Specific Adaptations: Different species would have evolved unique strategies to cope with environmental challenges, predation pressures, and reproductive demands. Cellular senescence would have evolved differently in various species to adapt to their specific ecological niches and life history traits.
Genetic Diversity and Mutation Accumulation: Genetic diversity within and between species, as well as the accumulation of mutations over generations, would lead to variations in the efficiency and regulation of cellular senescence mechanisms. Some species would have evolved more effective cellular repair and maintenance mechanisms, leading to extended lifespans.
Evolutionary Constraints: Evolution is constrained by existing genetic and molecular networks. The emergence of cellular senescence would have been influenced by the pre-existing genetic architecture, limiting the range of possible evolutionary trajectories.
Social and Cooperative Behavior: In social species, where cooperative behavior and division of labor are prominent, cellular senescence would have evolved to ensure proper allocation of resources within the colony or group. This would impact individual longevity.
Environmental Factors and Evolutionary Pressure: Environmental factors, such as exposure to predators, pathogens, and resource availability, would shape the evolution of senescence mechanisms. Species facing high mortality rates due to external factors would evolve senescence mechanisms to ensure rapid turnover and reproduction.
In summary, the evolution of mechanisms for cellular senescence and their effects on tissue homeostasis and longevity involve a complex interplay of genetic, environmental, and selective factors. Different species would have adapted unique strategies to balance the advantages of cellular senescence in terms of tissue maintenance and growth with its potential costs in terms of aging and decreased longevity.
Is there scientific evidence supporting the idea that cellular senescence systems were brought about by the process of evolution?
The stepwise evolution of cellular senescence, as required by conventional evolutionary theory, faces insurmountable challenges due to the intricate interdependence of various components. The complexity and functional requirements of the mechanisms involved in cellular senescence raise significant doubts about the feasibility of a gradual evolutionary pathway. Cellular senescence entails the integration of multiple levels of information, including regulatory codes, languages, signaling networks, and protein interactions. These components need to be operational from the beginning to create a functional system. Without the simultaneous existence and proper coordination of all these components, any intermediate stages would likely bear no selective advantage. Consider, for instance, the establishment of regulatory codes and languages. The instantiation of epigenetic marks, transcription factor binding sites, and promoter sequences requires a coordinated effort. Without functional signaling pathways to interpret cues and regulate gene expression, these codes would lack context and purpose. Similarly, the presence of protein interaction domains without the relevant proteins and their specific post-translational modifications would be inconsequential. The signaling pathways involved in cellular senescence present another challenge. Signaling networks are intricately intertwined, crosstalk between pathways is common, and the activation of one pathway often requires the presence and interaction of proteins from other pathways. This interdependence suggests that the full set of pathways needed for cellular senescence would need to be in place right from the start. Any intermediate stage lacking crucial components would likely lead to non-functional or detrimental outcomes, leaving no room for gradual selection. Furthermore, the concept of antagonistic pleiotropy, often invoked to explain the evolution of aging mechanisms, faces difficulties in explaining the complex orchestration of cellular senescence. While some genes may provide benefits early in life at the cost of later-life effects, the regulatory systems for senescence involve numerous genes, pathways, and processes that require simultaneous coordination. A gradual accumulation of such changes without an intelligent design process would likely lead to non-functional or maladaptive outcomes.
Irreducibility and Interdependence of the systems to instantiate and operate cellular senescence
Cellular senescence involves a complex interplay of manufacturing, signaling, and regulatory codes and languages that are not only irreducible but also intricately interdependent. The seamless operation of these components is essential for the functional establishment and maintenance of cellular senescence.
Epigenetic Codes and Regulatory Languages
Irreducibility: Epigenetic codes, such as DNA methylation and histone modifications, work in conjunction with transcriptional regulatory languages. These codes determine whether genes are silenced or activated. Without these codes, genes involved in senescence, including those regulating cell cycle arrest and senescence-associated secretory phenotype (SASP), would not be properly regulated.
Interdependence: Regulatory languages, such as transcription factor binding sites and promoter sequences, communicate with epigenetic codes. The binding of transcription factors to specific sites on DNA is influenced by epigenetic marks. Without the correct epigenetic context, these regulatory languages would lack functionality and specificity.
Signaling Pathways and Crosstalk
Irreducibility: Signaling pathways, such as the p53, mTOR, and MAPK pathways, are essential for interpreting cellular cues that trigger senescence. These pathways interconnect and influence each other's activation states. Without all relevant pathways, cells would be unable to appropriately respond to senescence-inducing triggers.
Interdependence: Crosstalk between signaling pathways allows cells to integrate multiple inputs and determine the appropriate response. For example, the p53 pathway interacts with the mTOR and MAPK pathways to make decisions between cell cycle arrest and proliferation. Without this interplay, cells might be unable to navigate complex decisions.
Protein Interaction and Modification Codes
Irreducibility: Protein interaction domains, post-translational modification codes, and chaperone systems are integral for proper protein function during senescence. Proteins must be folded correctly, modified appropriately, and interact with other proteins to carry out senescence-related tasks. Without these codes, proteins involved in cell cycle arrest and SASP would lack functionality.
Interdependence: Protein modifications, such as phosphorylation and acetylation, communicate with protein interaction domains. Modifications can influence protein interactions, localization, and stability. Without proper modifications, protein networks critical for senescence would not function as intended.
Feedback and Communication Systems
Irreducibility: Feedback loops are essential for controlling the progression and termination of cellular senescence. These loops involve various codes and signaling pathways. Without feedback mechanisms, cells might enter into a state of permanent senescence or fail to properly terminate the process.
Interdependence: Communication between feedback loops and other systems, including epigenetic regulation and signaling pathways, ensures the balanced operation of cellular senescence. These interactions prevent uncontrolled cell cycle arrest or prolonged secretion of inflammatory factors.
Given the irreducible and interdependent nature of these manufacturing, signaling, and regulatory codes and languages, the stepwise evolution of cellular senescence becomes implausible. The intricate coordination and simultaneous existence of all these components are required for functional senescence mechanisms. Intermediate stages with incomplete or partial components would likely be non-functional and disadvantageous, thus rendering a gradual evolutionary pathway unlikely. Instead, an intelligent design perspective suggests that cellular senescence needed to be instantiated and created all at once, fully operational, to achieve the intricacies of its function within complex cellular systems.
Once cellular senescence is instantiated and operational, what other intra and extracellular systems is it interdependent with?
Once cellular senescence is instantiated and operational, it becomes interdependent with a range of intra and extracellular systems, as its effects extend beyond individual cells and influence various aspects of tissue and organismal function. Some of these interdependent systems include:
Tissue Homeostasis and Repair Mechanisms
Cellular senescence is interconnected with tissue homeostasis and repair processes. It helps prevent the proliferation of damaged or potentially harmful cells, maintaining tissue integrity.
The clearance of senescent cells by immune cells and other mechanisms is crucial for tissue regeneration and healing.
Immune System: Cellular senescence contributes to immune system regulation and inflammation. Senescent cells can secrete factors that recruit immune cells to clear them.
The immune response to senescent cells, including immune surveillance and immune-mediated clearance, is an important aspect of senescence control.
Inflammation and SASP: Senescence-associated secretory phenotype (SASP) involves the secretion of various factors, including cytokines and growth factors.
The SASP influences local and systemic inflammation, immune responses, tissue remodeling, and even the progression of age-related diseases.
DNA Repair and Maintenance Pathways: Cellular senescence is closely linked to DNA damage response pathways. It can be triggered by irreparable DNA damage, serving as a mechanism to prevent the propagation of damaged genetic material. DNA repair and maintenance systems influence the induction and regulation of senescence.
Metabolism and Nutrient Sensing Pathways: Metabolic pathways and nutrient sensing systems can regulate senescence. Nutrient deprivation or specific metabolic conditions can induce or delay the senescence process.
Senescent cells also exhibit metabolic changes that influence their secretory profile.
Aging and Longevity: Cellular senescence is connected to aging and longevity. Accumulation of senescent cells over time contributes to age-related tissue dysfunction. The balance between senescence, tissue repair, and regeneration influences overall organismal aging trajectories.
Cancer Suppression: Cellular senescence plays a role in cancer suppression by halting the proliferation of potentially tumorigenic cells. Tumor suppression mechanisms and DNA repair pathways intersect with the induction of senescence.
Extracellular Matrix Remodeling: Senescent cells can secrete enzymes that remodel the extracellular matrix, which can impact tissue architecture and function. Interactions between senescent cells and the extracellular matrix influence tissue structure and integrity.
Stem Cell Dynamics: Senescence can affect stem cell populations and their regenerative potential. The presence of senescent cells can alter the stem cell microenvironment and influence tissue regeneration.
In summary, cellular senescence is not an isolated process but is tightly interwoven with a network of intra and extracellular systems. Its effects on tissue homeostasis, inflammation, immune response, DNA repair, metabolism, aging, and more emphasize its complex interactions within the broader context of cellular and organismal biology. These interdependencies contribute to the overall function and impact of cellular senescence on various physiological processes.
Complex Biological Systems Require Purposeful Design
1. Complex biological systems, such as cellular senescence, exhibit intricate interactions involving semiotic codes, languages, and interdependent mechanisms. In our observations of the natural world, systems that involve intricate codes, languages, and dependencies are typically associated with intentional design.
2. Cellular Senescence Involves Semiotic Codes and Languages: Cellular senescence relies on regulatory codes, epigenetic languages, and signaling pathways that communicate critical information. These codes determine gene expression, coordinate responses, and regulate cell behavior.
3. Interdependence of Cellular Senescence and Other Systems: Cellular senescence demonstrates an interdependence with various systems, including tissue homeostasis, the immune response, inflammation, DNA repair, metabolism, aging, and more. These interconnected systems collectively contribute to the orchestration of cellular behavior and tissue function.
4. Simultaneous Emergence Points to Design: The simultaneous emergence of multiple interdependent systems necessary for cellular senescence, all functioning coherently, indicates purposeful design. Gradual, stepwise evolution would not account for the interlocking requirements of these systems.
5. Complexity Beyond Chance: The complexity of cellular senescence and its intricate interactions within diverse systems suggests a level of sophistication that surpasses what random chance or unguided processes could reasonably produce.
Design as a Reasonable Explanation: The evident design in the interdependent systems governing cellular senescence aligns with the idea of an intelligent designer orchestrating these mechanisms. The intricate coordination and interplay among these systems point to an intentional setup rather than a series of chance events. In conclusion, the interdependent nature of cellular senescence and its integration with other biological systems, all relying on semiotic codes, languages, and intricate coordination, provides compelling evidence for a purposeful design that goes beyond the realm of random processes. The complexity and coherence of these systems strongly suggest an intelligent designer as the best explanation for their existence and function.
Alejandra Hernandez-Segura: Hallmarks of Cellular Senescence June 2018
Cellular senescence is a biological phenomenon in which cells lose their ability to divide and function properly due to various factors, including DNA damage, stress, or reaching the maximum number of divisions (replicative senescence). Senescent cells undergo distinct changes in gene expression and morphology, often becoming larger and developing a characteristic senescence-associated secretory phenotype (SASP). This phenotype involves the secretion of various molecules, including inflammatory cytokines, growth factors, and proteases, which can impact neighboring cells and the tissue microenvironment.
Importance in Biological Systems
Cellular senescence plays a dual role in biological systems. On one hand, it serves as a mechanism to prevent damaged or potentially cancerous cells from proliferating, which helps maintain tissue integrity and suppress tumor formation. On the other hand, the accumulation of senescent cells over time contributes to aging and age-related diseases. Senescent cells can promote inflammation, tissue dysfunction, and impair the regenerative capacity of tissues. Cellular senescence has an important role in the developmental processes that shape organismal form and function. During embryonic development, senescence helps regulate tissue morphogenesis by eliminating cells that are not needed or have the potential to disrupt proper development. This process is called developmental senescence. It ensures that organs and tissues form correctly and that unnecessary structures are removed. Senescence also contributes to tissue repair and regeneration during development. For example, during limb development, some cells undergo senescence to guide the precise patterning of fingers or toes.
How does cellular senescence contribute to tissue aging and development?
The effects of cellular senescence can be both beneficial and detrimental, depending on the context and timing. Here's how cellular senescence influences tissue aging and development:
Tissue Aging
Accumulation of Senescent Cells: Over time, senescent cells can accumulate in tissues due to various factors such as exposure to stress, DNA damage, and chronic inflammation. These cells have lost their ability to divide and can persist in tissues, contributing to age-related changes.
Senescence-Associated Secretory Phenotype (SASP): Senescent cells undergo changes in gene expression, leading to the secretion of inflammatory cytokines, growth factors, and other molecules. This SASP can promote chronic inflammation, disrupt tissue homeostasis, and contribute to age-related diseases.
Tissue Dysfunction: The presence of senescent cells and their SASP can impair tissue function by promoting fibrosis (scar tissue formation), altering the extracellular matrix, and interfering with the regenerative capacity of stem cells. This can lead to decreased tissue functionality and an overall decline in organ performance.
Loss of Regenerative Potential: Senescent cells can negatively affect the regenerative capacity of tissues. They can impair the activity of nearby stem cells and hinder tissue repair and renewal, leading to decreased resilience against damage and aging.
Development
Tissue Patterning and Morphogenesis: During embryonic development, cellular senescence plays a role in tissue patterning and morphogenesis. Some cells undergo senescence to help sculpt and refine developing structures. This process ensures proper formation of organs and body parts.
Clearance of Unwanted Cells: Senescence can help eliminate cells that are no longer needed during development. This ensures the removal of structures or cell populations that could interfere with proper tissue formation.
Guidance of Tissue Regeneration: In certain developmental contexts, senescent cells can guide tissue regeneration. For instance, during limb development, senescent cells may contribute to precise finger or toe patterning.
What are the molecular triggers and pathways that lead to cellular senescence?
Cellular senescence is a complex process regulated by various molecular triggers and pathways. Several factors can induce senescence, including DNA damage, telomere attrition, oncogene activation, and oxidative stress.
DNA Damage: DNA damage activates the DNA damage response (DDR) pathway, involving proteins like ATM, ATR, and p53. Activation of p53 can lead to cell cycle arrest and senescence through transcriptional regulation of genes involved in cell cycle control and senescence.
Telomere Attrition: Telomeres, protective caps at the ends of chromosomes, shorten with each cell division. Critically short telomeres trigger a DNA damage response, leading to p53 activation and cell cycle arrest.
Oncogene Activation: Certain oncogenes (genes that promote cell growth) can induce senescence when activated. The RAS oncogene pathway is known to contribute to senescence induction.
Oxidative Stress: High levels of reactive oxygen species (ROS) can cause cellular damage. ROS-induced DNA damage and activation of DDR can lead to senescence.
Epigenetic Changes: Alterations in epigenetic marks, such as DNA methylation and histone modifications, can drive senescence.
Senescence-associated heterochromatin foci (SAHF) form due to epigenetic changes and contribute to gene silencing.
Mitochondrial Dysfunction: Impaired mitochondrial function and increased ROS production can lead to senescence. Mitochondrial dysfunction contributes to the aging process and senescence.
Inflammatory Signaling: The senescence-associated secretory phenotype (SASP) involves the secretion of inflammatory cytokines, chemokines, and growth factors. SASP promotes inflammation and can affect neighboring cells and the tissue microenvironment.
Senescence-Associated Kinase Pathways: Various kinases, such as mTOR (mammalian target of rapamycin) and p38 MAPK (mitogen-activated protein kinase), are implicated in senescence regulation.
Autophagy Deficiency: Impaired autophagy (cellular recycling process) can lead to accumulation of damaged cellular components and senescence.
Non-Coding RNAs: MicroRNAs and long non-coding RNAs can regulate senescence-associated pathways and gene expression. The interplay of these triggers and pathways contributes to the intricate regulation of cellular senescence.
Depending on the context and combination of factors, cells can enter a state of senescence, which can have both protective and detrimental effects on the organism. Senescence serves as a mechanism to prevent damaged cells from proliferating and potentially becoming cancerous, but the accumulation of senescent cells can contribute to aging and age-related diseases.
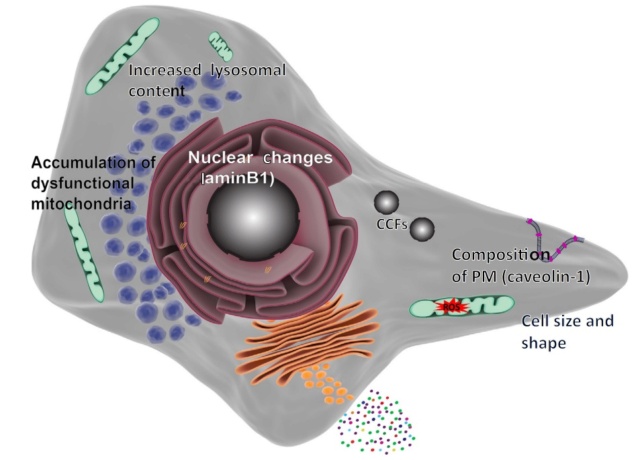
Hallmarks of Senescence Morphological Alterations. The molecular pathways of senescence result in morphological alterations. Senescent cells are enlarged and have an irregular shape; their nuclear integrity is compromised
due to the loss of laminB1, which also leads to the appearance of cytoplasmic chromatin fragments (CCFs); they have an increased lysosomal content, which is often detected as high b-galactosidase activity; they have large but dysfunctional mitochondria that produce high levels of reactive oxygen species (ROS); and their plasma membrane (PM) changes its composition (for instance, upregulating caveolin-1). 1
Appearance of Cellular Senescence in the evolutionary timeline
Cellular senescence has likely emerged relatively early in the evolution of complex multicellular organisms. While the exact timing and origins of cellular senescence are not fully elucidated, evidence suggests that its emergence is intertwined with the evolution of multicellularity and complex life forms. Here's a general overview of how cellular senescence might have appeared in the evolutionary timeline:
Origin of Simple Multicellularity: Around 1 to 2 billion years ago, simple multicellular organisms, such as algae and early colonial organisms, would have started to evolve. As cells came together to form tissues, mechanisms to prevent uncontrolled growth and maintain tissue integrity would have emerged. These mechanisms would be considered rudimentary forms of what we now understand as cellular senescence.
Emergence of More Complex Multicellular Life: About 600 million years ago, more complex multicellular life forms would have began to evolve, including early metazoans (animals). With the development of more specialized cell types and tissues, the need to regulate cell division and prevent runaway growth would have become more critical.
Balancing Senescence and Regeneration: Throughout evolution, organisms would have needed to strike a balance between the protective effects of cellular senescence (preventing cancer and tissue overgrowth) and the necessity for tissue regeneration and repair. Cellular senescence would have evolved as a way to prevent damaged cells from propagating in multicellular organisms, contributing to the overall fitness of the organism.
Variability in Senescence Mechanisms: Different organisms would have developed variations of senescence mechanisms based on their specific needs and environmental pressures. Senescence would have evolved independently in different lineages, leading to diverse regulatory pathways and outcomes.
Evolution of Longevity and Aging: As organisms would have evolved, longer lifespans would have become advantageous for various reasons, such as increased reproductive opportunities and the development of more complex behaviors. Cellular senescence would have become intertwined with aging processes, contributing to age-related changes and the development of age-related diseases.
De Novo Genetic Information necessary to instantiate Cellular Senescence
Creating a hypothetical process for generating new genetic information and introducing it in the correct sequence to establish the mechanisms of cellular senescence involves conceptualizing a scenario where new functional elements are added to an existing genetic system. Here's a simplified outline of what such a process might entail:
DNA Sequences Encoding Senescence Factors: New DNA sequences would need to originate that encode for specific proteins and regulatory elements involved in cellular senescence.
These sequences should include instructions for the synthesis of proteins that regulate cell cycle arrest, senescence-associated secretory phenotype (SASP) components, and factors that induce the senescent state.
Regulatory Elements for Expression: Alongside the new DNA sequences, regulatory elements such as promoters, enhancers, and transcription factor binding sites would be necessary.
These regulatory elements would ensure that the genes involved in senescence are expressed at the appropriate times and in the correct cell types.
Protein Interaction Networks: New information would have to emerge to establish protein-protein interaction networks that allow the newly synthesized senescence-associated proteins to function together in a coordinated manner.
This would involve the de novo generation of functional protein domains, motifs, and interaction interfaces.
Signaling Pathways and Feedback Loops: Information would need to originate to establish signaling pathways that sense cellular stress, DNA damage, or other triggers of senescence.
Feedback loops and crosstalk mechanisms would be required to fine-tune the response and duration of the senescence process.
Epigenetic Changes and Chromatin Remodeling: The process would necessitate the emergence of mechanisms that induce epigenetic changes leading to altered gene expression patterns associated with senescence.
Histone modifications, DNA methylation patterns, and chromatin remodeling would need to be established.
Establishment of Senescence Phenotype: The correct sequence of events would need to be orchestrated, starting with the activation of senescence-inducing factors, followed by cell cycle arrest, morphological changes, and the secretion of SASP components.
Coordination with Developmental Programs: The hypothetical process should ensure that the induction of cellular senescence is coordinated with other developmental processes to maintain tissue integrity and overall functionality.
In this speculative scenario, the challenge lies in generating entirely new genetic information de novo, including sequences encoding proteins, regulatory elements, and complex functional networks.
This description simplifies a highly complex and multifaceted biological process and emphasizes the requirement for coordinated and functional information to establish the mechanisms of cellular senescence without invoking evolutionary mechanisms. It's important to note that real biological systems are much more intricate and involve a dynamic interplay of genetics, epigenetics, and molecular networks.
Manufacturing codes and languages that would have to emerge and be employed to instantiate Cellular Senescence
Regulatory Sequences and Transcription Factors: New regulatory sequences, such as enhancers and silencers, would need to emerge de novo. Transcription factors specific to senescence-related genes would need to originate, recognizing these regulatory sequences and activating the genes involved in cellular senescence.
Protein Folding and Modification Codes: A new set of protein folding codes and chaperone molecules would need to be established to ensure that the newly synthesized senescence-associated proteins are correctly folded into their functional three-dimensional structures. Post-translational modification codes, like phosphorylation and acetylation, would need to be created to regulate protein activities.
Signaling Networks and Cascade Codes: Signaling cascades would have to be introduced, involving the de novo generation of receptor-ligand interactions and intracellular signaling codes. Feedback loops and response thresholds would need to be established to coordinate the cellular response to senescence triggers.
Secretory Pathway Codes: Manufacturing codes would be required to assemble the components of the secretory pathway that allow for the packaging and secretion of senescence-associated factors, including inflammatory cytokines and growth factors.
Epigenetic Modification Machinery: Enzymatic codes for DNA methylation and histone modification would need to be introduced to establish the specific epigenetic changes associated with cellular senescence.
Cellular Localization Signals: New signals and codes directing the correct localization of senescence-associated proteins within the cell would need to be instantiated.
Morphological Changes Coordination: Codes would be necessary to coordinate the morphological changes associated with cellular senescence, such as cell enlargement and alterations in organelle distribution.
Crosstalk and Feedback Mechanisms: Complex codes for crosstalk and feedback mechanisms would have to be introduced to ensure the proper coordination of cellular senescence with other cellular processes and environmental cues.
Timed Expression Codes: Codes for temporal regulation of gene expression and protein activity would need to be established to ensure that senescence-associated processes occur at the appropriate times during an organism's life cycle.
In this speculative scenario, the focus is on the non-genetic manufacturing aspects required to transition from an organism lacking cellular senescence to one with a fully developed senescence process. The challenge here lies in conceiving a hypothetical process where intricate codes and languages are instantiated and coordinated to create the mechanisms of cellular senescence, beyond the genetic information previously outlined. The actual biological processes involve a highly sophisticated interplay of molecular interactions, signaling networks, and cellular machineries.
Epigenetic Regulatory Mechanisms necessary to be instantiated for Cellular Senescence
The hypothetical development of cellular senescence from scratch would require intricate epigenetic regulations to establish and maintain the process. Several systems would need to collaborate to ensure the proper instantiation and balance of these regulations:
Epigenetic Regulations
DNA Methylation: A system of DNA methyltransferases would need to be created to introduce DNA methylation marks at specific regulatory regions. Methylation can silence genes involved in inhibiting senescence or activate genes promoting senescence.
Histone Modifications: Enzymatic complexes would be required to introduce histone modifications like H3K9me3 and H4K20me3 associated with gene silencing and heterochromatin formation. These modifications can impact gene expression profiles central to senescence.
Chromatin Remodeling: An ATP-dependent chromatin remodeling system would be necessary to restructure chromatin and provide accessibility to regulatory elements, influencing senescence-associated gene expression.
Non-Coding RNAs: Systems for producing and processing non-coding RNAs, such as microRNAs and long non-coding RNAs, would need to emerge to regulate gene expression involved in senescence.
Collaborating Systems
Cell Signaling Pathways: Intracellular signaling networks would operate in conjunction with epigenetic regulation to detect stress, DNA damage, or other senescence triggers. These pathways would integrate signals and activate downstream effectors that modulate epigenetic marks.
Cell Cycle Machinery: The cell cycle control machinery, including cyclins and cyclin-dependent kinases, would interact with epigenetic regulators to establish cell cycle arrest during senescence.
DNA Repair Systems: DNA repair mechanisms would work in collaboration with epigenetic regulators to ensure the accurate repair of DNA damage, which can influence the activation of senescence pathways.
Inflammatory Signaling: Systems for sensing and responding to inflammatory cues would interact with epigenetic regulators, shaping the senescence-associated secretory phenotype (SASP).
Feedback and Crosstalk
Feedback loops between epigenetic modifications, gene expression, and signaling pathways would ensure the proper progression and termination of cellular senescence. Crosstalk mechanisms between different epigenetic modifications and regulatory systems would help maintain the balance between cellular senescence and other cellular processes, such as cell proliferation and survival. In this speculative scenario, the establishment and operation of epigenetic regulations for cellular senescence involve the orchestration of multiple complex systems. The collaboration between these systems is essential for fine-tuning the activation, maintenance, and coordination of cellular senescence within the broader cellular context.
Signaling Pathways necessary to create, and maintain Cellular Senescence
The emergence of cellular senescence from scratch would require the establishment of intricate signaling pathways that are interconnected, interdependent, and capable of crosstalk with each other and other biological systems:
p53 Pathway
The p53 pathway would need to be established to sense DNA damage, cellular stress, or oncogenic activation. p53 activation leads to transcriptional upregulation of genes involved in cell cycle arrest and senescence. This pathway interacts with the DNA repair machinery to ensure accurate DNA damage response. Crosstalk with other pathways, such as the mTOR pathway, influences the decision between cell cycle arrest and continued proliferation.
mTOR Pathway
The mTOR pathway would need to integrate nutrient and growth factor signals. Inhibition of mTOR can induce senescence through effects on metabolism and autophagy. Interacts with the p53 pathway to modulate senescence outcome based on cellular context.
Rb Pathway
The Rb pathway would need to control the cell cycle by inhibiting E2F transcription factors. Rb pathway dysfunction can lead to senescence. Crosstalk with other pathways, including p53 and mTOR, influences the decision between senescence and proliferation.
MAPK Pathways
MAPK pathways, including ERK and p38, would need to integrate stress and growth factor signals. Activation of p38 MAPK can trigger senescence through regulation of p53 and other downstream effectors. Crosstalk between MAPK pathways and the p53 pathway can influence the decision to undergo senescence.
TGF-β Pathway
The TGF-β pathway would need to be established to regulate cell growth, differentiation, and senescence. Activation of TGF-β can induce cell cycle arrest and senescence. Interacts with other pathways to modulate senescence in response to external cues.
NF-κB Pathway
The NF-κB pathway would need to be involved in sensing inflammation and oxidative stress. NF-κB activation leads to the transcription of genes associated with the senescence-associated secretory phenotype (SASP).
Crosstalk with other pathways, such as p53 and MAPK, influences the coordination between inflammation and senescence.
Interconnectedness and Crosstalk
These pathways are interconnected, with molecules and signals shared between them. Crosstalk occurs through direct interactions, shared transcriptional targets, and feedback loops. The balance between these pathways determines the decision between senescence, proliferation, and other cellular responses.
Interdependence with Other Systems
Signaling pathways crosstalk not only with each other but also with other biological systems. They interact with epigenetic regulation, DNA repair, metabolic networks, and cellular machinery involved in senescence.
Together, these interactions ensure the integration of senescence signals with broader cellular functions and responses. In this speculative scenario, the emergence of cellular senescence requires the intricate establishment and coordination of signaling pathways that are interconnected, interdependent, and capable of crosstalk. These pathways collaboratively govern the decision-making processes that lead to the initiation, maintenance, and regulation of cellular senescence within the context of broader biological systems.
Regulatory codes necessary for maintenance and operation of Cellular Senescence
The establishment, maintenance, and operation of cellular senescence would necessitate the instantiation of intricate regulatory codes and languages that orchestrate the complex processes involved:
Epigenetic Regulatory Codes
DNA Methylation Patterns: Specific DNA methylation marks would need to be established at regulatory regions to silence or activate genes involved in cellular senescence.
Histone Modification Codes: Codes for histone modifications, such as H3K9me3 and H4K20me3, would need to be instantiated to influence gene expression profiles during senescence.
Chromatin Remodeling Instructions: Codes would be required to direct ATP-dependent chromatin remodeling complexes to modulate chromatin accessibility.
Transcriptional Regulatory Languages
Promoter and Enhancer Codes: Regulatory sequences like promoters and enhancers would need to be established to drive gene expression of senescence-related factors.
Transcription Factor Binding Instructions: Binding sites for transcription factors like those associated with p53 and other senescence-inducing proteins would need to be instantiated.
Signaling Integration and Interpretation
Signaling Code Interpretation: Cellular signaling networks would need codes to interpret inputs from DNA damage, oxidative stress, inflammation, and other triggers of senescence.
Activation and Inhibition Codes: Codes for activation and inhibition of specific signaling pathways would determine the overall cellular response to senescence-inducing cues.
Protein Interaction and Modification Instructions
Protein Interaction Domains: Specific protein interaction domains would be needed to enable the assembly of senescence-associated protein complexes.
Post-Translational Modification Codes: Codes for post-translational modifications like phosphorylation, acetylation, and ubiquitination would influence protein function and stability.
Feedback and Threshold Codes
Feedback Loop Codes: Codes for positive and negative feedback loops would help regulate the progression and termination of cellular senescence.
Threshold Detection Instructions: Codes would need to be established to detect certain thresholds of DNA damage or other senescence-inducing cues.
Temporal and Spatial Coordination Languages
Temporal Regulation Codes: Instructions for temporal regulation would ensure that cellular senescence is initiated and maintained at appropriate times during an organism's life cycle.
Spatial Localization Codes: Codes for spatial localization would direct senescence-related processes to specific cellular compartments.
Secretory Phenotype Code
SASP Regulation Codes: Codes would be necessary to regulate the expression and secretion of components of the senescence-associated secretory phenotype (SASP).
In this speculative scenario, the regulatory codes and languages required for the maintenance and operation of cellular senescence encompass diverse aspects of gene expression, signaling integration, protein interactions, and feedback mechanisms. The orchestration of these codes ensures the intricate and coordinated execution of cellular senescence within the broader cellular context.
How did the mechanisms for cellular senescence supposedly evolve to balance tissue homeostasis and longevity in different species?
The supposed evolution of mechanisms for cellular senescence and their role in balancing tissue homeostasis and longevity in different species is a complex topic that involves a combination of genetic, environmental, and selective pressures. While the exact evolutionary pathways are not fully elucidated, researchers propose several mechanisms and theories to explain how cellular senescence could have evolved to contribute to these balances:
Antagonistic Pleiotropy Theory: This theory suggests that certain genes or mechanisms that confer benefits early in life but have detrimental effects later in life would have been favored by natural selection. In the context of cellular senescence, genes that promote growth and development early in life would also contribute to cellular damage and senescence later in life. Such genes would be selected for their positive effects on reproduction and fitness, even though they lead to aging-related effects.
Trade-Offs between Growth and Longevity: Evolutionary trade-offs between allocating resources for growth and reproduction versus maintenance and longevity would influence the emergence of senescence mechanisms. Species that allocate more resources toward growth and reproduction would have shorter lifespans due to increased cellular damage and senescence, whereas species that prioritize maintenance would have longer lifespans.
Species-Specific Adaptations: Different species would have evolved unique strategies to cope with environmental challenges, predation pressures, and reproductive demands. Cellular senescence would have evolved differently in various species to adapt to their specific ecological niches and life history traits.
Genetic Diversity and Mutation Accumulation: Genetic diversity within and between species, as well as the accumulation of mutations over generations, would lead to variations in the efficiency and regulation of cellular senescence mechanisms. Some species would have evolved more effective cellular repair and maintenance mechanisms, leading to extended lifespans.
Evolutionary Constraints: Evolution is constrained by existing genetic and molecular networks. The emergence of cellular senescence would have been influenced by the pre-existing genetic architecture, limiting the range of possible evolutionary trajectories.
Social and Cooperative Behavior: In social species, where cooperative behavior and division of labor are prominent, cellular senescence would have evolved to ensure proper allocation of resources within the colony or group. This would impact individual longevity.
Environmental Factors and Evolutionary Pressure: Environmental factors, such as exposure to predators, pathogens, and resource availability, would shape the evolution of senescence mechanisms. Species facing high mortality rates due to external factors would evolve senescence mechanisms to ensure rapid turnover and reproduction.
In summary, the evolution of mechanisms for cellular senescence and their effects on tissue homeostasis and longevity involve a complex interplay of genetic, environmental, and selective factors. Different species would have adapted unique strategies to balance the advantages of cellular senescence in terms of tissue maintenance and growth with its potential costs in terms of aging and decreased longevity.
Is there scientific evidence supporting the idea that cellular senescence systems were brought about by the process of evolution?
The stepwise evolution of cellular senescence, as required by conventional evolutionary theory, faces insurmountable challenges due to the intricate interdependence of various components. The complexity and functional requirements of the mechanisms involved in cellular senescence raise significant doubts about the feasibility of a gradual evolutionary pathway. Cellular senescence entails the integration of multiple levels of information, including regulatory codes, languages, signaling networks, and protein interactions. These components need to be operational from the beginning to create a functional system. Without the simultaneous existence and proper coordination of all these components, any intermediate stages would likely bear no selective advantage. Consider, for instance, the establishment of regulatory codes and languages. The instantiation of epigenetic marks, transcription factor binding sites, and promoter sequences requires a coordinated effort. Without functional signaling pathways to interpret cues and regulate gene expression, these codes would lack context and purpose. Similarly, the presence of protein interaction domains without the relevant proteins and their specific post-translational modifications would be inconsequential. The signaling pathways involved in cellular senescence present another challenge. Signaling networks are intricately intertwined, crosstalk between pathways is common, and the activation of one pathway often requires the presence and interaction of proteins from other pathways. This interdependence suggests that the full set of pathways needed for cellular senescence would need to be in place right from the start. Any intermediate stage lacking crucial components would likely lead to non-functional or detrimental outcomes, leaving no room for gradual selection. Furthermore, the concept of antagonistic pleiotropy, often invoked to explain the evolution of aging mechanisms, faces difficulties in explaining the complex orchestration of cellular senescence. While some genes may provide benefits early in life at the cost of later-life effects, the regulatory systems for senescence involve numerous genes, pathways, and processes that require simultaneous coordination. A gradual accumulation of such changes without an intelligent design process would likely lead to non-functional or maladaptive outcomes.
Irreducibility and Interdependence of the systems to instantiate and operate cellular senescence
Cellular senescence involves a complex interplay of manufacturing, signaling, and regulatory codes and languages that are not only irreducible but also intricately interdependent. The seamless operation of these components is essential for the functional establishment and maintenance of cellular senescence.
Epigenetic Codes and Regulatory Languages
Irreducibility: Epigenetic codes, such as DNA methylation and histone modifications, work in conjunction with transcriptional regulatory languages. These codes determine whether genes are silenced or activated. Without these codes, genes involved in senescence, including those regulating cell cycle arrest and senescence-associated secretory phenotype (SASP), would not be properly regulated.
Interdependence: Regulatory languages, such as transcription factor binding sites and promoter sequences, communicate with epigenetic codes. The binding of transcription factors to specific sites on DNA is influenced by epigenetic marks. Without the correct epigenetic context, these regulatory languages would lack functionality and specificity.
Signaling Pathways and Crosstalk
Irreducibility: Signaling pathways, such as the p53, mTOR, and MAPK pathways, are essential for interpreting cellular cues that trigger senescence. These pathways interconnect and influence each other's activation states. Without all relevant pathways, cells would be unable to appropriately respond to senescence-inducing triggers.
Interdependence: Crosstalk between signaling pathways allows cells to integrate multiple inputs and determine the appropriate response. For example, the p53 pathway interacts with the mTOR and MAPK pathways to make decisions between cell cycle arrest and proliferation. Without this interplay, cells might be unable to navigate complex decisions.
Protein Interaction and Modification Codes
Irreducibility: Protein interaction domains, post-translational modification codes, and chaperone systems are integral for proper protein function during senescence. Proteins must be folded correctly, modified appropriately, and interact with other proteins to carry out senescence-related tasks. Without these codes, proteins involved in cell cycle arrest and SASP would lack functionality.
Interdependence: Protein modifications, such as phosphorylation and acetylation, communicate with protein interaction domains. Modifications can influence protein interactions, localization, and stability. Without proper modifications, protein networks critical for senescence would not function as intended.
Feedback and Communication Systems
Irreducibility: Feedback loops are essential for controlling the progression and termination of cellular senescence. These loops involve various codes and signaling pathways. Without feedback mechanisms, cells might enter into a state of permanent senescence or fail to properly terminate the process.
Interdependence: Communication between feedback loops and other systems, including epigenetic regulation and signaling pathways, ensures the balanced operation of cellular senescence. These interactions prevent uncontrolled cell cycle arrest or prolonged secretion of inflammatory factors.
Given the irreducible and interdependent nature of these manufacturing, signaling, and regulatory codes and languages, the stepwise evolution of cellular senescence becomes implausible. The intricate coordination and simultaneous existence of all these components are required for functional senescence mechanisms. Intermediate stages with incomplete or partial components would likely be non-functional and disadvantageous, thus rendering a gradual evolutionary pathway unlikely. Instead, an intelligent design perspective suggests that cellular senescence needed to be instantiated and created all at once, fully operational, to achieve the intricacies of its function within complex cellular systems.
Once cellular senescence is instantiated and operational, what other intra and extracellular systems is it interdependent with?
Once cellular senescence is instantiated and operational, it becomes interdependent with a range of intra and extracellular systems, as its effects extend beyond individual cells and influence various aspects of tissue and organismal function. Some of these interdependent systems include:
Tissue Homeostasis and Repair Mechanisms
Cellular senescence is interconnected with tissue homeostasis and repair processes. It helps prevent the proliferation of damaged or potentially harmful cells, maintaining tissue integrity.
The clearance of senescent cells by immune cells and other mechanisms is crucial for tissue regeneration and healing.
Immune System: Cellular senescence contributes to immune system regulation and inflammation. Senescent cells can secrete factors that recruit immune cells to clear them.
The immune response to senescent cells, including immune surveillance and immune-mediated clearance, is an important aspect of senescence control.
Inflammation and SASP: Senescence-associated secretory phenotype (SASP) involves the secretion of various factors, including cytokines and growth factors.
The SASP influences local and systemic inflammation, immune responses, tissue remodeling, and even the progression of age-related diseases.
DNA Repair and Maintenance Pathways: Cellular senescence is closely linked to DNA damage response pathways. It can be triggered by irreparable DNA damage, serving as a mechanism to prevent the propagation of damaged genetic material. DNA repair and maintenance systems influence the induction and regulation of senescence.
Metabolism and Nutrient Sensing Pathways: Metabolic pathways and nutrient sensing systems can regulate senescence. Nutrient deprivation or specific metabolic conditions can induce or delay the senescence process.
Senescent cells also exhibit metabolic changes that influence their secretory profile.
Aging and Longevity: Cellular senescence is connected to aging and longevity. Accumulation of senescent cells over time contributes to age-related tissue dysfunction. The balance between senescence, tissue repair, and regeneration influences overall organismal aging trajectories.
Cancer Suppression: Cellular senescence plays a role in cancer suppression by halting the proliferation of potentially tumorigenic cells. Tumor suppression mechanisms and DNA repair pathways intersect with the induction of senescence.
Extracellular Matrix Remodeling: Senescent cells can secrete enzymes that remodel the extracellular matrix, which can impact tissue architecture and function. Interactions between senescent cells and the extracellular matrix influence tissue structure and integrity.
Stem Cell Dynamics: Senescence can affect stem cell populations and their regenerative potential. The presence of senescent cells can alter the stem cell microenvironment and influence tissue regeneration.
In summary, cellular senescence is not an isolated process but is tightly interwoven with a network of intra and extracellular systems. Its effects on tissue homeostasis, inflammation, immune response, DNA repair, metabolism, aging, and more emphasize its complex interactions within the broader context of cellular and organismal biology. These interdependencies contribute to the overall function and impact of cellular senescence on various physiological processes.
Complex Biological Systems Require Purposeful Design
1. Complex biological systems, such as cellular senescence, exhibit intricate interactions involving semiotic codes, languages, and interdependent mechanisms. In our observations of the natural world, systems that involve intricate codes, languages, and dependencies are typically associated with intentional design.
2. Cellular Senescence Involves Semiotic Codes and Languages: Cellular senescence relies on regulatory codes, epigenetic languages, and signaling pathways that communicate critical information. These codes determine gene expression, coordinate responses, and regulate cell behavior.
3. Interdependence of Cellular Senescence and Other Systems: Cellular senescence demonstrates an interdependence with various systems, including tissue homeostasis, the immune response, inflammation, DNA repair, metabolism, aging, and more. These interconnected systems collectively contribute to the orchestration of cellular behavior and tissue function.
4. Simultaneous Emergence Points to Design: The simultaneous emergence of multiple interdependent systems necessary for cellular senescence, all functioning coherently, indicates purposeful design. Gradual, stepwise evolution would not account for the interlocking requirements of these systems.
5. Complexity Beyond Chance: The complexity of cellular senescence and its intricate interactions within diverse systems suggests a level of sophistication that surpasses what random chance or unguided processes could reasonably produce.
Design as a Reasonable Explanation: The evident design in the interdependent systems governing cellular senescence aligns with the idea of an intelligent designer orchestrating these mechanisms. The intricate coordination and interplay among these systems point to an intentional setup rather than a series of chance events. In conclusion, the interdependent nature of cellular senescence and its integration with other biological systems, all relying on semiotic codes, languages, and intricate coordination, provides compelling evidence for a purposeful design that goes beyond the realm of random processes. The complexity and coherence of these systems strongly suggest an intelligent designer as the best explanation for their existence and function.
Alejandra Hernandez-Segura: Hallmarks of Cellular Senescence June 2018