DNA & origin of life scenarios
The importance of finding convincing prebiotic pathways to synthesize nucleosides and nucleotides cannot be overestimated. One can almost say that the world is waiting for the answer for more than 60 years.
Nucleoside Diphosphate Kinase
For three of the four nucleotides—ADP, CDP, and GDP—the conversion from diphosphate (dNDP) to the triphosphate (dNTP) involves simply a phosphorylation of the rNDP reductase product, catalyzed by nucleoside diphosphate kinase. In most cells, this enzyme is very active, and all known forms of the enzyme have very low specificity. Thus, the enzyme catalyzes the reversible transfer of the g-phosphate of any common rNTP or dNTP to the phosphate at the b-position of any common rNDP or dNDP. The equilibrium constant for each reaction catalyzed is close to unity. Thus, the direction in which a nucleoside diphosphate kinase-catalyzed reaction occurs in vivo depends on the concentrations of substrates and products. Because ATP is almost always the most abundant intracellular nucleoside triphosphate, most such reactions involve the ATP-dependent conversion of a ribo- or deoxyribonucleoside diphosphate to the corresponding triphosphate.
Adenine
Adenine is one of the most important organic molecules for life as we know it today.
Adenine and guanine are derived from the nucleotide inosine monophosphate (IMP), which in turn is synthesized from a pre-existing ribose phosphate through a complex pathway using atoms from the amino acids glycine, glutamine, and aspartic acid, as well as the coenzyme tetrahydrofolate. 12
Adenine is one of the most important organic molecules for life as we know it today.
Adenine is an integral part of DNA, RNA, and ATP. DNA, as you might know, is the genetic code used for cellular life on earth. It is through the precise inheritance of on organism's DNA from its parent that the traits of an organism are passed on. Here is the partial structure of DNA with an Adenine group attached. Adenine is a purine. Purines are six-membered rings attached to five-membered rings. When Adenine is attached to DNA, it forms a bond with another molecule called Thymine, a pyrimidine, on the other side of the DNA strand. It is these bonds which give DNA its double-helix structure. The sequence of DNA, or the order in which nucleotides are placed, allows for the diversity among all living organisms. The importance of Adenine to RNA is similar to that of DNA.
Besides DNA and RNA, Adenine is also an important part of adenosine triphosphate, or ATP. Adenosine triphosphate is the nitrogenous base adenine bonded to a five-carbon sugar. This molecule is important because it has the ability to phosphorylize, or add a phosphate group to, other molecules. This transfer of a phosphate group allows energy to be released. It is this energy which is used by cells in living organisms. This is why the molecules ATP, and its nitrogenous base Adenine, are so important.
"Adenine synthesis is perhaps the best example of an irreducibly complex system that can be found in life ..."
The process doesn't work unless all 11 enzymes are present. ( So we have a chicken/egg problem here )
Adenine synthesis requires unreasonable HCN concentrations. Adenine deaminates with a half-life of 80 years (at 37°C, pH 7). Therefore, adenine would never accumulate in any kind of "prebiotic soup." The adenine-uracil interaction is weak and nonspecific, and, therefore, would never be expected to function in any specific recognition scheme under the chaotic conditions of a "prebiotic soup."
a In biochemistry, the addition of a formyl functional group is termed formylation. A formyl functional group consists of a carbonyl bonded to hydrogen. When attached to an R group, a formyl group is called an aldehyde. 3
b 10-Formyltetrahydrofolate (10-CHO-THF) is a form of tetrahydrofolate that acts as a donor of formyl groups in anabolism. In these reactions, 10-CHO-THF is used as a substrate in formyltransferase reactions. This is important in purine biosynthesis, where 10-CHO-THF is a substrate for phosphoribosylaminoimidazolecarboxamide formyltransferase, as well as in the formylation of the methionyl initiator tRNA (fMet-tRNA), when 10-CHO-THF is a substrate for methionyl-tRNA formyltransferase 4
Adenine biosynthesis
The key riddle remains: how do five HCN molecules combine to form adenine under prebiotic conditions? 3
Although the formation of adenine by the pentamerization of HCN is very exothermic, this process is quite unlikely in isolation (gas phase). Not only must five HCN molecules come together, but also the reaction barriers are very high. The intimate participation of an additional molecule, such as H2O or NH3 (or perhaps HCN) is needed to lower the barriers considerably to realistic energies.
Adenine is an integral part of DNA, RNA, and ATP. DNA, as you might know, is the genetic code used for cellular life on earth. It is through the precise inheritance of on organism's DNA from its parent that the traits of an organism are passed on. Here is the partial structure of DNA with an Adenine group attached. Adenine is a purine. Purines are six-membered rings attached to five membered rings. When Adenine is attached to DNA, it forms a bond with another molecule called Thymine, a pyrimidine, on the other side of the DNA strand. It is these bonds which give DNA its double-helix structure. The sequence of DNA, or the order in which nucleotides are placed, allows for the diversity among all living organisms. The importance of Adenine to RNA is similar to that of DNA.
Besides DNA and RNA, Adenine is also an important part of adenosine triphosphate, or ATP. Adenosine triphosphate is the nitrogenous base adenine bonded to a five carbon sugar. This molecule is important because it has the ability to phosphorylize, or add a phosphate group to, other molecules. This transfer of a phosphate group allows energy to be released. It is this energy which is used by cells in living organisms. This is why the molecules ATP, and its nitrogenous base Adenine, are so important.
"Adenine synthesis is perhaps the best example of an irreducibly complex system that can be found in life ..."
the process doesn't work unless all 11 enzymes are present.
Adenine synthesis requires unreasonable HCN concentrations. Adenine deaminates with a half-life of 80 years (at 37°C, pH 7). Therefore, adenine would never accumulate in any kind of "prebiotic soup." The adenine-uracil interaction is weak and nonspecific, and, therefore, would never be expected to function in any specific recognition scheme under the chaotic conditions of a "prebiotic soup." 1
Shapiro also critically analyzed prebiotic simulation experiments that produced the DNA and RNA component adenine. As with cytosine, he showed that adenine formation on early Earth (by currently recognized prebiotic routes) could not reasonably have occurred, for many of the same reasons. 2

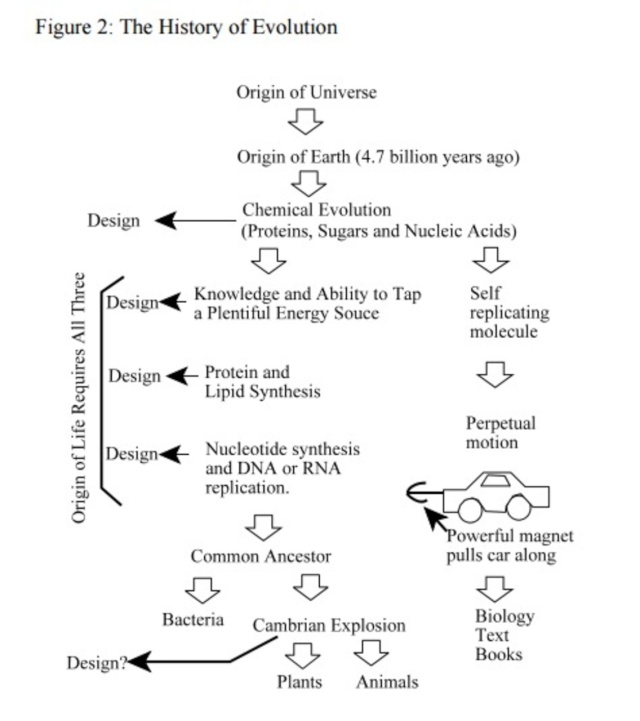
Adenine biosynthesis pathway :
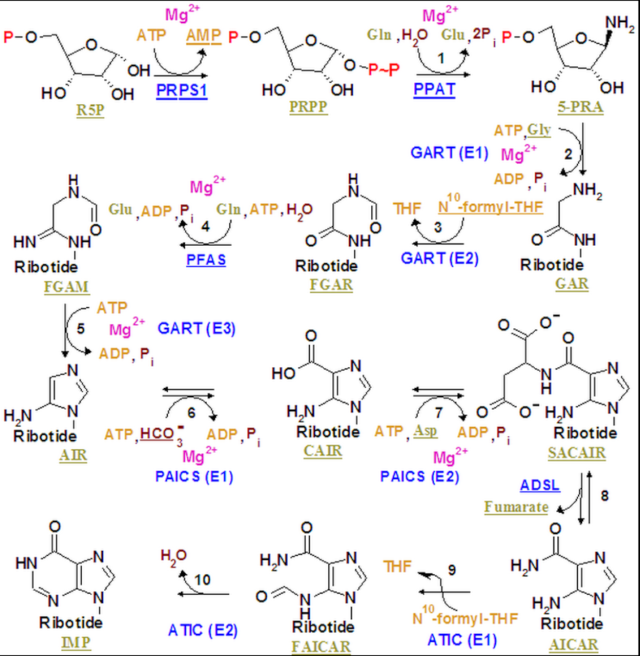
https://books.google.com.br/books?id=5ZGUD49fMcAC&pg=PA206&lpg=PA206&dq=Metabolic+pathways,+irreducible+complexity&source=bl&ots=FdagRE2T-M&sig=pVeMIrlHluDJSkL2Bp3Si4M4Xh4&hl=pt-BR&sa=X&ei=s1pNVZPQAcmpNsb8gbAH&ved=0CFsQ6AEwBzge#v=onepage&q=Metabolic%20pathways%2C%20irreducible%20complexity&f=false

Amidophosphoribosyltransferase
Prebiotic cytosine synthesis: A critical analysis and implications for the origin of life 4
The deamination of cytosine and its destruction by other processes such as photochemical reactions place severe constraints on prebiotic cytosine syntheses. If cytosine concentrations are to be maintained on a worldwide basis, then synthesis must be sufficient to replace depletion. The syntheses described thus far do not possess the necessary speed and selectivity to meet this requirement. The use of drying lagoons as a site for prebiotic synthesis has been suggested as a remedy: synthetic rates would be enhanced by greatly increasing the concentration of the reagents. The lagoon suggestion appears geologically implausible, however. All schemes in which cytosine is synthesized locally and distributed globally also are handicapped in that the enormous dilution that takes place when cytosine is released into a global sea offsets any gain in synthetic efficiency.
Guanine
Guanine is one of the four main nucleobases found in the nucleic acids DNA and RNA.
For scientists attempting to understand how the building blocks of RNA originated on Earth, guanine -- the G in the four-letter code of life -- has proven to be a particular challenge. While the other three bases of RNA -- adenine (A), cytosine (C) and uracil (U) -- could be created by heating a simple precursor compound in the presence of certain naturally occurring catalysts, guanine had not been observed as a product of the same reactions.
Thymine
DNA can only be replicated in the presence of specific enzymes (described below ) which can only be manufactured by the already existing DNA. Each is absolutely essential for the other, and both must be present for the DNA to multiply. Therefore, DNA has to have been in existence in the beginning for life to be controlled by DNA. Scott M. Huse, "The Collapse of Evolution", Baker Book House: Grand Rapids (Michigan), 1983 p:93-94
Prebiotic ribose synthesis: A critical analysis
The evidence that is currently available does not support the availability of ribose on the prebiotic earth, except perhaps for brief periods of time, in low concentration as part of a complex mixture, and under conditions unsuitable for nucleoside synthesis. 1
Sugars are very unstable, and easily decompose or react with other chemicals. This counts against any proposed mechanism to concentrate them to useable proportions. 2
Asphalt, Water, and the Prebiotic Synthesis of Ribose, Ribonucleosides, and RNA 3
RNA has been called a “prebiotic chemist’s nightmare” because of its combination of large size, carbohydrate building blocks, bonds that are thermodynamically unstable in water, and overall intrinsic instability.
However, a discontinuous synthesis model is well-supported by experimental work that might produce RNA from atmospheric CO2, H2O, and N2. For example, electrical discharge in such atmospheres gives formaldehyde (HCHO) in large amounts and glycolaldehyde (HOCH2CHO) in small amounts. When rained into alkaline aquifers generated by serpentinizing rocks, these substances were undoubtedly converted to carbohydrates including ribose. Likewise, atmospherically generated HCN was undoubtedly converted in these aquifers to formamide and ammonium formate, precursors for RNA nucleobases. Finally, high reduction potentials maintained by mantle-derived rocks and minerals would allow phosphite to be present in equilibrium with phosphate, mobilizing otherwise insoluble phosphorus for the prebiotic synthesis of phosphite and phosphate esters after oxidation.
So why does the community not view this discontinuous synthesis model as compelling evidence for the RNA-first hypothesis for the origin of life? In part, the model is deficient because no experiments have joined together those steps without human intervention. Further, many steps in the model have problems. Some are successful only if reactive compounds are presented in a specific order in large amounts. Failing controlled addition, the result produces complex mixtures that are inauspicious precursors for biology, a situation described as the “asphalt problem”. Many bonds in RNA are thermodynamically unstable with respect to hydrolysis in water, creating a “water problem”. Finally, some bonds in RNA appear to be “impossible” to form under any conditions considered plausible for early Earth.
A new chicken-and-egg paradox relating to the origin of life
http://www.uncommondescent.com/intelligent-design/do-viruses-help-explain-the-origin-of-life/
Cells could not have evolved without viruses, as they need reverse transcriptase (which is found only in viruses) in order to move from RNA to DNA.
In other words, instead of helping to solve the problem of the origin of life on Earth, recent research has only served to highlight one of its central paradoxes. And yet the science media reports the latest discoveries as if the solution is just around the corner. Don’t you find that just a little strange?
“In order to move from RNA to DNA, you need an enzyme called reverse transcriptase,” Dolja said. “It’s only found in viruses like HIV, not in cells. So how could cells begin to use DNA without the help of a virus?”
https://en.wikipedia.org/wiki/Reverse_transcriptase#In_eukaryotes
Creation of double-stranded DNA occurs in the cytosol as a series of these steps:
A specific cellular tRNA acts as a primer and hybridizes to a complementary part of the virus RNA genome called the primer binding site or PBS
Complementary DNA then binds to the U5 (non-coding region) and R region (a direct repeat found at both ends of the RNA molecule) of the viral RNA
A domain on the reverse transcriptase enzyme called RNAse H degrades the 5’ end of the RNA which removes the U5 and R region
The primer then ‘jumps’ to the 3’ end of the viral genome and the newly synthesised DNA strands hybridizes to the complementary R region on the RNA
The first strand of complementary DNA (cDNA) is extended and the majority of viral RNA is degraded by RNAse H
Once the strand is completed, second strand synthesis is initiated from the viral RNA
There is then another ‘jump’ where the PBS from the second strand hybridizes with the complementary PBS on the first strand
Both strands are extended further and can be incorporated into the hosts genome by the enzyme integrase
Creation of double-stranded DNA also involves strand transfer, in which there is a translocation of short DNA product from initial RNA dependent DNA synthesis to acceptor template regions at the other end of the genome, which are later reached and processed by the reverse transcriptase for its DNA-dependent DNA activity
To date, scientists have failed to produce cytosine in a spark-discharge experiment, nor has cytosine been recovered from meteorites or extraterrestrial sources. Because meteorites (and other extraterrestrial materials) serve as a proxy for early Earth’s chemistry, the absence of cytosine in these sources would seem to affirm Shapiro’s conclusion. Shapiro also critically analyzed prebiotic simulation experiments that produced the DNA and RNA component adenine. As with cytosine, he showed that adenine formation on early Earth (by currently recognized prebiotic routes) could not reasonably have occurred, for many of the same reasons. Recent work by James Cleaves and Stanley Miller uncovers an additional problem. Nucleobases readily react with formaldehyde and acetaldehyde, compounds most certainly present on early Earth, to form both small molecule derivatives and large intractable molecules. Even under mild conditions, these reactions take place so rapidly that they would preferentially occur at the expense of reactions that could lead to RNA. Thus, if nucleobases could form, competing reactions would likely consume them.
https://books.google.com.br/books?id=rlIyVJ9dpzkC&pg=PA149&lpg=PA149&dq=thymidylate+synthase+origin+of+life&source=bl&ots=cocJIaoVZg&sig=VtlcxunaYAkxx4DZwpEjvHnh_-0&hl=en&sa=X&ei=AnyHVYyRA8qy-AG1v4HYBw&ved=0CDAQ6AEwAw#v=onepage&q=thymidylate%20synthase%20origin%20of%20life&f=false

Prebiotic thymine synthesis

1) http://www.pnas.org/content/96/8/4396.full
2) http://www.trueorigin.org/originoflife.php
3) http://www.talkorigins.org/faqs/abioprob/originoflife.html
4) http://www.evolutionnews.org/2009/07/scientists_say_intelligent_des022621.html
5) http://www.rsc.org/chemistryworld/News/2009/May/13050902.asp
6) http://www.sciencedaily.com/releases/2009/04/090416161133.htm
7) http://www.ncbi.nlm.nih.gov/pubmed/20525631
1) http://www.ncbi.nlm.nih.gov/pubmed/11536683?dopt=Abstract
2) Hugh Ross & Fazale Rana, Origins of life pg.79
3) http://www.pnas.org/content/104/44/17272.full
1) http://www.ncbi.nlm.nih.gov/pubmed/2453009
2) http://creationwiki.org/Origin_of_life
3) http://pubs.acs.org/doi/abs/10.1021/ar200332w
further readings :
https://www.c4id.org.uk/index.php?option=com_content&view=article&id=211:the-problem-of-the-origin-of-life&catid=50:genetics&Itemid=43
http://www.ncbi.nlm.nih.gov/books/NBK6360/figure/A43731/?report=objectonly
Metabolic pathways for RNA and DNA precursors biosynthesis: a palimpsest from the RNA to DNA world transition? The biosynthetic pathways for purine and pyrimidine nucleotides both start with ribose 5-monophosphate. The formation of the four bases requires several amino-acids, formate and carbamyl-phosphate. Nucleotide monophosphates (NMP) are converted into RNA precursors (NTP) by NMP kinases (k) and NDP kinases (K). These reactions probably are relics of the RNA-protein world. DNA precursors are produced from NDP and/or NTP by ribonucleotide reductases (RNR), except for dTTP, which results from methylation of dUMP. dTMP is produced from dUMP by Thymidylate synthases (ThyA or ThyX) and converted into dTTP by the same kinases that convert NMP into NTP. dUMP can be produced either by dUTPAse or by dCTP deaminase. In the U-DNA world, it could have been also produced by degradation of U-DNA. The mode of dTMP production clearly suggests that U-DNA was an evolutionary intermediate between RNA and T-DNA. Some viruses contain U-DNA, whereas others contain HMC-DNA (HMC= hydroxymethyl-cytosine). Transformation of C into HMC occurs at the level of dCMP, and conversion of dCMP into dHMCMP is catalyzed by a dCMP hydroxy-methyl transferase (dCMP HM transferase), which is homologue to ThyA (See refs. 11, 14, and 19 for more details).
[url=https://servimg.com/view/17307623/802][img]https://i.servimg.com/u/f18/17/30/76/23/ch314f1
The importance of finding convincing prebiotic pathways to synthesize nucleosides and nucleotides cannot be overestimated. One can almost say that the world is waiting for the answer for more than 60 years.
Nucleoside Diphosphate Kinase
For three of the four nucleotides—ADP, CDP, and GDP—the conversion from diphosphate (dNDP) to the triphosphate (dNTP) involves simply a phosphorylation of the rNDP reductase product, catalyzed by nucleoside diphosphate kinase. In most cells, this enzyme is very active, and all known forms of the enzyme have very low specificity. Thus, the enzyme catalyzes the reversible transfer of the g-phosphate of any common rNTP or dNTP to the phosphate at the b-position of any common rNDP or dNDP. The equilibrium constant for each reaction catalyzed is close to unity. Thus, the direction in which a nucleoside diphosphate kinase-catalyzed reaction occurs in vivo depends on the concentrations of substrates and products. Because ATP is almost always the most abundant intracellular nucleoside triphosphate, most such reactions involve the ATP-dependent conversion of a ribo- or deoxyribonucleoside diphosphate to the corresponding triphosphate.
Adenine
Adenine is one of the most important organic molecules for life as we know it today.
Adenine and guanine are derived from the nucleotide inosine monophosphate (IMP), which in turn is synthesized from a pre-existing ribose phosphate through a complex pathway using atoms from the amino acids glycine, glutamine, and aspartic acid, as well as the coenzyme tetrahydrofolate. 12
Adenine is one of the most important organic molecules for life as we know it today.
Adenine is an integral part of DNA, RNA, and ATP. DNA, as you might know, is the genetic code used for cellular life on earth. It is through the precise inheritance of on organism's DNA from its parent that the traits of an organism are passed on. Here is the partial structure of DNA with an Adenine group attached. Adenine is a purine. Purines are six-membered rings attached to five-membered rings. When Adenine is attached to DNA, it forms a bond with another molecule called Thymine, a pyrimidine, on the other side of the DNA strand. It is these bonds which give DNA its double-helix structure. The sequence of DNA, or the order in which nucleotides are placed, allows for the diversity among all living organisms. The importance of Adenine to RNA is similar to that of DNA.
Besides DNA and RNA, Adenine is also an important part of adenosine triphosphate, or ATP. Adenosine triphosphate is the nitrogenous base adenine bonded to a five-carbon sugar. This molecule is important because it has the ability to phosphorylize, or add a phosphate group to, other molecules. This transfer of a phosphate group allows energy to be released. It is this energy which is used by cells in living organisms. This is why the molecules ATP, and its nitrogenous base Adenine, are so important.
"Adenine synthesis is perhaps the best example of an irreducibly complex system that can be found in life ..."
The process doesn't work unless all 11 enzymes are present. ( So we have a chicken/egg problem here )
Adenine synthesis requires unreasonable HCN concentrations. Adenine deaminates with a half-life of 80 years (at 37°C, pH 7). Therefore, adenine would never accumulate in any kind of "prebiotic soup." The adenine-uracil interaction is weak and nonspecific, and, therefore, would never be expected to function in any specific recognition scheme under the chaotic conditions of a "prebiotic soup."
a In biochemistry, the addition of a formyl functional group is termed formylation. A formyl functional group consists of a carbonyl bonded to hydrogen. When attached to an R group, a formyl group is called an aldehyde. 3
b 10-Formyltetrahydrofolate (10-CHO-THF) is a form of tetrahydrofolate that acts as a donor of formyl groups in anabolism. In these reactions, 10-CHO-THF is used as a substrate in formyltransferase reactions. This is important in purine biosynthesis, where 10-CHO-THF is a substrate for phosphoribosylaminoimidazolecarboxamide formyltransferase, as well as in the formylation of the methionyl initiator tRNA (fMet-tRNA), when 10-CHO-THF is a substrate for methionyl-tRNA formyltransferase 4
Adenine biosynthesis
The key riddle remains: how do five HCN molecules combine to form adenine under prebiotic conditions? 3
Although the formation of adenine by the pentamerization of HCN is very exothermic, this process is quite unlikely in isolation (gas phase). Not only must five HCN molecules come together, but also the reaction barriers are very high. The intimate participation of an additional molecule, such as H2O or NH3 (or perhaps HCN) is needed to lower the barriers considerably to realistic energies.
Adenine is an integral part of DNA, RNA, and ATP. DNA, as you might know, is the genetic code used for cellular life on earth. It is through the precise inheritance of on organism's DNA from its parent that the traits of an organism are passed on. Here is the partial structure of DNA with an Adenine group attached. Adenine is a purine. Purines are six-membered rings attached to five membered rings. When Adenine is attached to DNA, it forms a bond with another molecule called Thymine, a pyrimidine, on the other side of the DNA strand. It is these bonds which give DNA its double-helix structure. The sequence of DNA, or the order in which nucleotides are placed, allows for the diversity among all living organisms. The importance of Adenine to RNA is similar to that of DNA.
Besides DNA and RNA, Adenine is also an important part of adenosine triphosphate, or ATP. Adenosine triphosphate is the nitrogenous base adenine bonded to a five carbon sugar. This molecule is important because it has the ability to phosphorylize, or add a phosphate group to, other molecules. This transfer of a phosphate group allows energy to be released. It is this energy which is used by cells in living organisms. This is why the molecules ATP, and its nitrogenous base Adenine, are so important.
"Adenine synthesis is perhaps the best example of an irreducibly complex system that can be found in life ..."
the process doesn't work unless all 11 enzymes are present.
Adenine synthesis requires unreasonable HCN concentrations. Adenine deaminates with a half-life of 80 years (at 37°C, pH 7). Therefore, adenine would never accumulate in any kind of "prebiotic soup." The adenine-uracil interaction is weak and nonspecific, and, therefore, would never be expected to function in any specific recognition scheme under the chaotic conditions of a "prebiotic soup." 1
Shapiro also critically analyzed prebiotic simulation experiments that produced the DNA and RNA component adenine. As with cytosine, he showed that adenine formation on early Earth (by currently recognized prebiotic routes) could not reasonably have occurred, for many of the same reasons. 2

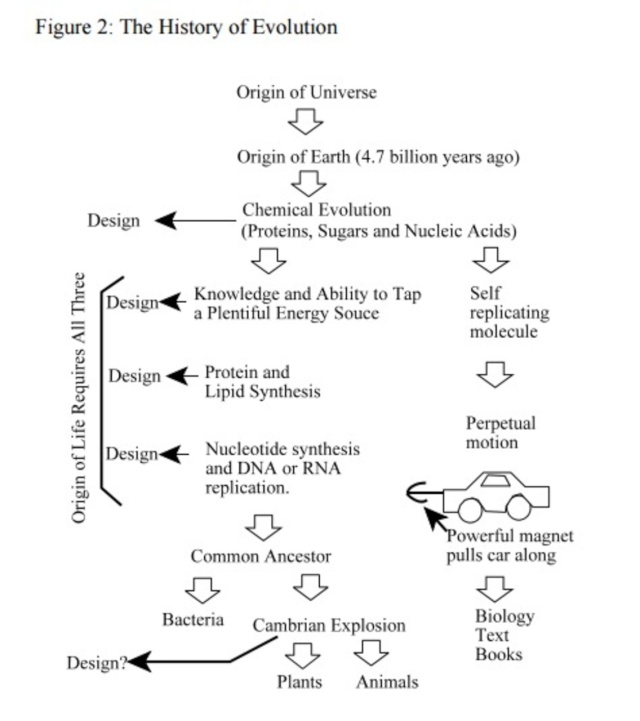
Adenine biosynthesis pathway :
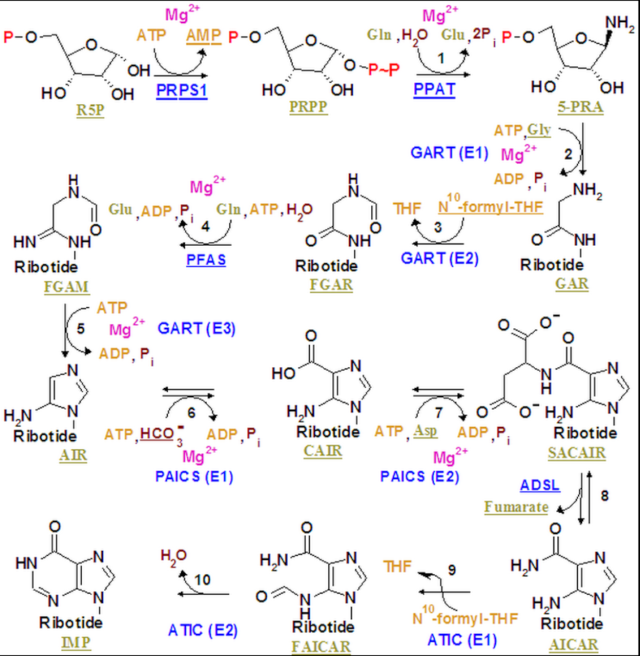
https://books.google.com.br/books?id=5ZGUD49fMcAC&pg=PA206&lpg=PA206&dq=Metabolic+pathways,+irreducible+complexity&source=bl&ots=FdagRE2T-M&sig=pVeMIrlHluDJSkL2Bp3Si4M4Xh4&hl=pt-BR&sa=X&ei=s1pNVZPQAcmpNsb8gbAH&ved=0CFsQ6AEwBzge#v=onepage&q=Metabolic%20pathways%2C%20irreducible%20complexity&f=false

Amidophosphoribosyltransferase
Prebiotic cytosine synthesis: A critical analysis and implications for the origin of life 4
The deamination of cytosine and its destruction by other processes such as photochemical reactions place severe constraints on prebiotic cytosine syntheses. If cytosine concentrations are to be maintained on a worldwide basis, then synthesis must be sufficient to replace depletion. The syntheses described thus far do not possess the necessary speed and selectivity to meet this requirement. The use of drying lagoons as a site for prebiotic synthesis has been suggested as a remedy: synthetic rates would be enhanced by greatly increasing the concentration of the reagents. The lagoon suggestion appears geologically implausible, however. All schemes in which cytosine is synthesized locally and distributed globally also are handicapped in that the enormous dilution that takes place when cytosine is released into a global sea offsets any gain in synthetic efficiency.
Guanine
Guanine is one of the four main nucleobases found in the nucleic acids DNA and RNA.
For scientists attempting to understand how the building blocks of RNA originated on Earth, guanine -- the G in the four-letter code of life -- has proven to be a particular challenge. While the other three bases of RNA -- adenine (A), cytosine (C) and uracil (U) -- could be created by heating a simple precursor compound in the presence of certain naturally occurring catalysts, guanine had not been observed as a product of the same reactions.
Thymine
DNA can only be replicated in the presence of specific enzymes (described below ) which can only be manufactured by the already existing DNA. Each is absolutely essential for the other, and both must be present for the DNA to multiply. Therefore, DNA has to have been in existence in the beginning for life to be controlled by DNA. Scott M. Huse, "The Collapse of Evolution", Baker Book House: Grand Rapids (Michigan), 1983 p:93-94
Thymidylate synthases (Thy) are key enzymes in the synthesis of deoxythymidylate, 1 of the 4 building blocks of DNA. As such, they are essential for all DNA-based forms of life and therefore implicated in the hypothesized transition from RNA genomes to DNA genomes. Two unrelated Thy enzymes, ThyA and ThyX, are known to catalyze the same biochemical reaction. 7
Thymidylate synthase (Thy) is a fundamental enzyme in DNA synthesis because it catalyzes the formation of deoxythymidine 5′-monophosphate (dTMP) from deoxyuridine 5′-monophosphate (dUMP). For decades, only one family of thymidylate synthase enzymes was known, and its presence was considered necessary to maintain all DNA-based forms of life. Then, a gene encoding an alternative enzyme was discovered and characterized , and the novel enzyme was named ThyX, whereas the other enzyme was renamed ThyA. Even though both reactions accomplish the same key step, the reaction mechanisms, or steps, catalyzed by the FDTS and TS enzymes are structurally different. The 2 enzymes, ThyA and ThyX, were found to have distinctly different sequences and structures, thus alluding to independent origins.
Thats interesting, as we find two distinct enzymes with two different sequences and structures synthesizing the same reaction, thus being a example of convergence right in the beginning. How remote was the chance for this to happen by natural means , considering, that convergence does not favour naturalistic explanations ?
as Stephen J.Gould wrote: “…No finale can be specified at the start, none would ever occur a second time in the same way, because any pathway proceeds through thousands of improbable stages. Alter any early event, ever so slightly, and without apparent importance at the time, and evolution cascades into a radically different channel.1
Stephen J. Gould, Wonderful Life: The Burgess Shale and the Nature of History (New York, NY: W.W. Norton & Company, 1989), 51.
By virtue of their function and phyletic distribution, Thys are ancient enzymes, implying 1) the likely participation of one or both enzymes during the transition from an RNA world to a DNA world (based on protein catalysts: Joyce 2002) and 2) the probable presence of a gene encoding Thy in the genome of the common ancestors of eukaryotes, bacteria, and archaea . Thus, tracing back the pathway of genes encoding ThyA and ThyX may shed light on the actively debated wider issue regarding the origins of viral and cellular DNA
This brings us to the same problem as with Ribonucleotide Reductase enzymes (RNR), which is the classic chicken and egg, catch22 situation. ThyA and ThyX enzymes are required to make DNA. DNA is however required to make these enzymes. What came first ?? We can conclude with high certainty that this enzyme buries any RNA world fantasies, and any possibility of transition from RNA to DNA world scenarios, since both had to come into existence at the same time.
Prebiotic cytosine synthesisThymidylate synthase (Thy) is a fundamental enzyme in DNA synthesis because it catalyzes the formation of deoxythymidine 5′-monophosphate (dTMP) from deoxyuridine 5′-monophosphate (dUMP). For decades, only one family of thymidylate synthase enzymes was known, and its presence was considered necessary to maintain all DNA-based forms of life. Then, a gene encoding an alternative enzyme was discovered and characterized , and the novel enzyme was named ThyX, whereas the other enzyme was renamed ThyA. Even though both reactions accomplish the same key step, the reaction mechanisms, or steps, catalyzed by the FDTS and TS enzymes are structurally different. The 2 enzymes, ThyA and ThyX, were found to have distinctly different sequences and structures, thus alluding to independent origins.
Thats interesting, as we find two distinct enzymes with two different sequences and structures synthesizing the same reaction, thus being a example of convergence right in the beginning. How remote was the chance for this to happen by natural means , considering, that convergence does not favour naturalistic explanations ?
as Stephen J.Gould wrote: “…No finale can be specified at the start, none would ever occur a second time in the same way, because any pathway proceeds through thousands of improbable stages. Alter any early event, ever so slightly, and without apparent importance at the time, and evolution cascades into a radically different channel.1
Stephen J. Gould, Wonderful Life: The Burgess Shale and the Nature of History (New York, NY: W.W. Norton & Company, 1989), 51.
By virtue of their function and phyletic distribution, Thys are ancient enzymes, implying 1) the likely participation of one or both enzymes during the transition from an RNA world to a DNA world (based on protein catalysts: Joyce 2002) and 2) the probable presence of a gene encoding Thy in the genome of the common ancestors of eukaryotes, bacteria, and archaea . Thus, tracing back the pathway of genes encoding ThyA and ThyX may shed light on the actively debated wider issue regarding the origins of viral and cellular DNA
This brings us to the same problem as with Ribonucleotide Reductase enzymes (RNR), which is the classic chicken and egg, catch22 situation. ThyA and ThyX enzymes are required to make DNA. DNA is however required to make these enzymes. What came first ?? We can conclude with high certainty that this enzyme buries any RNA world fantasies, and any possibility of transition from RNA to DNA world scenarios, since both had to come into existence at the same time.
Cytosine is one of the four main bases found in DNA and RNA, along with adenine, guanine, and thymine (uracil in RNA).
Cytosine has not been reported in analyses of meteorites nor is it among the products of electric spark discharge experiments. The reported prebiotic syntheses of cytosine involve the reaction of cyanoacetylene (or its hydrolysis product, cyanoacetaldehyde), with cyanate, cyanogen, or urea. These substances undergo side reactions with common nucleophiles that appear to proceed more rapidly than cytosine formation. To favor cytosine formation, reactant concentrations are required that are implausible in a natural setting. Furthermore, cytosine is consumed by deamination (the half-life for deamination at 25°C is ≈340 yr) and other reactions. No reactions have been described thus far that would produce cytosine, even in a specialized local setting, at a rate sufficient to compensate for its decomposition. 1
The evidence that is available at the present time does not support the idea that RNA, or an alternative replicator that uses the current set of RNA bases, was present at the start of life.
The RNA/DNA base cytosine is not produced in spark discharge experiments. The proposed prebiotic productions are chemically unrealistic because the alleged precursors are unlikely to be concentrated enough, and they would undergo side reactions with other organic compounds, or hydrolyse. Cytosine itself is too unstable to accumulate over alleged geological ‘deep time’, as its half life for deamination is 340 years at 25 °C. 2
For a long time the synthesis of RNA monomers under prebiotic conditions appeared to be a fundamental problem since the condensation of sugar (ribose) and nucleobase (purines and pyrimidines) does not work (Orgel, 2004). The prebiotic synthesis of purine ribonucleotides is still unclear, yet recently a breakthrough has been made with regard to the synthesis of pyrimidine ribonucleotide monomers (which incorporate cytosine and uracil). It now appears in principle to be solved, in a completely unexpected manner. The study by the group of John Sutherland (Powner et al. 2009) shows how nature could have spontaneously assembled pyrimidine ribonucleotide monomers from prebiotically plausible molecules through intermediates that contribute atoms to both the sugar and base portions of the ribonucleotides, thus avoiding a condensation step of sugar and base altogether 3
Sutherland's research produced only 2 of the 4 RNA nucleobases, and Dr. Garner also explained why, as is often the case, the basic chemistry itself also required the hand of an intelligent chemist:
As far as being relevant to OOL, the chemistry has all of the usual problems. The starting materials are "plausibly" obtainable by abiotic means, but need to be kept isolated from one another until the right step, as Sutherland admits. One of the starting materials is a single mirror image for which there is no plausible way to get it that way abiotically. Then Sutherland ran these reactions as any organic chemist would, with pure materials under carefully controlled conditions. In general, he purified the desired products after each step, and adjusted the conditions (pH, temperature, etc.) to maximum advantage along the way. Not at all what one would expect from a lagoon of organic soup. He recognized that making of a lot of biologically problematic side products was inevitable, but found that UV light applied at the right time and for the right duration could destroy much (?) of the junk without too much damage to the desired material. Meaning, of course, that without great care little of the desired chemistry would plausibly occur. But it is more than enough for true believers in OOL to rejoice over, and, predictably, to way overstate in the press. 4
Robert Shapiro, professor emeritus of chemistry at New York University disagrees.
'Although as an exercise in chemistry this represents some very elegant work, this has nothing to do with the origin of life on Earth whatsoever,' he says. According to Shapiro, it is hard to imagine RNA forming in a prebiotic world along the lines of Sutherland's synthesis.
'The chances that blind, undirected, inanimate chemistry would go out of its way in multiple steps and use of reagents in just the right sequence to form RNA is highly unlikely,' argues Shapiro. 5
In the book origins of life, Hugh Ross writes at page 79 :
Cytosine is one of the molecular components of nucleic acids (DNA and RNA), cytosine assumes an important place in both RNA-world and pre-RNA-world origin-of-life models (this posits that RNA or some RNA precursor chemically evolved before proteins and DNA). Cytosine, a pyrimidine, is a six-membered ring composed of four carbon atoms and two nitrogen atoms. Along with other ring compounds, such as adenine, guanine, thymine, and uracil, cytosine repeatedly extends from the chainlike backbone of DNA and RNA. The nitrogen-containing rings sequenced along DNA or RNA provide the chemical information that determines biochemical function. Chemists have discovered two possible pathways that produce cytosine. One route involves a reaction between cyanoacetylene and cyanate, and the other reaction begins with cyanoacetaldehyde and urea.6 These four compounds represent essential ingredients of early Earth’s supposed prebiotic soup. Chemist Robert Shapiro demonstrated, however, that the two chemical routes lack any relevance.7 He points out the unlikelihood that cyanoacetylene, cyanate, cyanoacetaldehyde, and urea existed at sufficient levels on primordial Earth to effect the production of cytosine. Even if they had occurred at appropriate levels, interfering chemical reactions would have quickly consumed these compounds before cytosine could form. Cyanoacetylene rapidly reacts with ammonia, amines, thiols, and hydrogen cyanide. Cyanate undergoes rapid reaction with water. In the presence of water, cyanoacetaldehyde decomposes into acetonitrile and formate. When cytosine does form, it rapidly decomposes. At room temperature and with a neutral pH, cytosine breaks down, losing half its molecules in 340 years. At 32 °F (0 °C), its half-life is 17,000 years—still too short a time for cytosine to be part of the supposed first self-replicator. To date, scientists have failed to produce cytosine in a spark-discharge experiment, nor has cytosine been recovered from meteorites or extraterrestrial sources. Because meteorites (and other extraterrestrial materials) serve as a proxy for early Earth’s chemistry, the absence of cytosine in these sources would seem to affirm Shapiro’s conclusion. Shapiro also critically analyzed prebiotic simulation experiments that produced the DNA and RNA component adenine. As with cytosine, he showed that adenine formation on early Earth (by currently recognized prebiotic routes) could not reasonably have occurred, for many of the same reasons. Recent work by James Cleaves and Stanley Miller uncovers an additional problem.11 Nucleobases readily react with formaldehyde and acetaldehyde, compounds most certainly present on early Earth, to form both small molecule derivatives and large intractable molecules. Even under mild conditions, these reactions take place so rapidly that they would preferentially occur at the expense of reactions that could lead to RNA. Thus, if nucleobases could form, competing reactions would likely consume them.
Prebiotic cytosine synthesis: A critical analysis and implications for the origin of life 1
The deamination of cytosine and its destruction by other processes such as photochemical reactions place severe constraints on prebiotic cytosine syntheses. If cytosine concentrations are to be maintained on a worldwide basis, then synthesis must be sufficient to replace depletion. The syntheses described thus far do not possess the necessary speed and selectivity to meet this requirement. The use of drying lagoons as a site for prebiotic synthesis has been suggested as a remedy: synthetic rates would be enhanced by greatly increasing the concentration of the reagents. The lagoon suggestion appears geologically implausible, however. All schemes in which cytosine is synthesized locally and distributed globally also are handicapped in that the enormous dilution that takes place when cytosine is released into a global sea offsets any gain in synthetic efficiency.
Prebiotic guanine synthesis
Guanine is one of the four main nucleobases found in the nucleic acids DNA and RNA.
For scientists attempting to understand how the building blocks of RNA originated on Earth, guanine -- the G in the four-letter code of life -- has proven to be a particular challenge. While the other three bases of RNA -- adenine (A), cytosine (C) and uracil (U) -- could be created by heating a simple precursor compound in the presence of certain naturally occurring catalysts, guanine had not been observed as a product of the same reactions. 1
The backbone made up of (deoxy-ribose) sugar moleculesCytosine has not been reported in analyses of meteorites nor is it among the products of electric spark discharge experiments. The reported prebiotic syntheses of cytosine involve the reaction of cyanoacetylene (or its hydrolysis product, cyanoacetaldehyde), with cyanate, cyanogen, or urea. These substances undergo side reactions with common nucleophiles that appear to proceed more rapidly than cytosine formation. To favor cytosine formation, reactant concentrations are required that are implausible in a natural setting. Furthermore, cytosine is consumed by deamination (the half-life for deamination at 25°C is ≈340 yr) and other reactions. No reactions have been described thus far that would produce cytosine, even in a specialized local setting, at a rate sufficient to compensate for its decomposition. 1
The evidence that is available at the present time does not support the idea that RNA, or an alternative replicator that uses the current set of RNA bases, was present at the start of life.
The RNA/DNA base cytosine is not produced in spark discharge experiments. The proposed prebiotic productions are chemically unrealistic because the alleged precursors are unlikely to be concentrated enough, and they would undergo side reactions with other organic compounds, or hydrolyse. Cytosine itself is too unstable to accumulate over alleged geological ‘deep time’, as its half life for deamination is 340 years at 25 °C. 2
For a long time the synthesis of RNA monomers under prebiotic conditions appeared to be a fundamental problem since the condensation of sugar (ribose) and nucleobase (purines and pyrimidines) does not work (Orgel, 2004). The prebiotic synthesis of purine ribonucleotides is still unclear, yet recently a breakthrough has been made with regard to the synthesis of pyrimidine ribonucleotide monomers (which incorporate cytosine and uracil). It now appears in principle to be solved, in a completely unexpected manner. The study by the group of John Sutherland (Powner et al. 2009) shows how nature could have spontaneously assembled pyrimidine ribonucleotide monomers from prebiotically plausible molecules through intermediates that contribute atoms to both the sugar and base portions of the ribonucleotides, thus avoiding a condensation step of sugar and base altogether 3
Sutherland's research produced only 2 of the 4 RNA nucleobases, and Dr. Garner also explained why, as is often the case, the basic chemistry itself also required the hand of an intelligent chemist:
As far as being relevant to OOL, the chemistry has all of the usual problems. The starting materials are "plausibly" obtainable by abiotic means, but need to be kept isolated from one another until the right step, as Sutherland admits. One of the starting materials is a single mirror image for which there is no plausible way to get it that way abiotically. Then Sutherland ran these reactions as any organic chemist would, with pure materials under carefully controlled conditions. In general, he purified the desired products after each step, and adjusted the conditions (pH, temperature, etc.) to maximum advantage along the way. Not at all what one would expect from a lagoon of organic soup. He recognized that making of a lot of biologically problematic side products was inevitable, but found that UV light applied at the right time and for the right duration could destroy much (?) of the junk without too much damage to the desired material. Meaning, of course, that without great care little of the desired chemistry would plausibly occur. But it is more than enough for true believers in OOL to rejoice over, and, predictably, to way overstate in the press. 4
Robert Shapiro, professor emeritus of chemistry at New York University disagrees.
'Although as an exercise in chemistry this represents some very elegant work, this has nothing to do with the origin of life on Earth whatsoever,' he says. According to Shapiro, it is hard to imagine RNA forming in a prebiotic world along the lines of Sutherland's synthesis.
'The chances that blind, undirected, inanimate chemistry would go out of its way in multiple steps and use of reagents in just the right sequence to form RNA is highly unlikely,' argues Shapiro. 5
In the book origins of life, Hugh Ross writes at page 79 :
Cytosine is one of the molecular components of nucleic acids (DNA and RNA), cytosine assumes an important place in both RNA-world and pre-RNA-world origin-of-life models (this posits that RNA or some RNA precursor chemically evolved before proteins and DNA). Cytosine, a pyrimidine, is a six-membered ring composed of four carbon atoms and two nitrogen atoms. Along with other ring compounds, such as adenine, guanine, thymine, and uracil, cytosine repeatedly extends from the chainlike backbone of DNA and RNA. The nitrogen-containing rings sequenced along DNA or RNA provide the chemical information that determines biochemical function. Chemists have discovered two possible pathways that produce cytosine. One route involves a reaction between cyanoacetylene and cyanate, and the other reaction begins with cyanoacetaldehyde and urea.6 These four compounds represent essential ingredients of early Earth’s supposed prebiotic soup. Chemist Robert Shapiro demonstrated, however, that the two chemical routes lack any relevance.7 He points out the unlikelihood that cyanoacetylene, cyanate, cyanoacetaldehyde, and urea existed at sufficient levels on primordial Earth to effect the production of cytosine. Even if they had occurred at appropriate levels, interfering chemical reactions would have quickly consumed these compounds before cytosine could form. Cyanoacetylene rapidly reacts with ammonia, amines, thiols, and hydrogen cyanide. Cyanate undergoes rapid reaction with water. In the presence of water, cyanoacetaldehyde decomposes into acetonitrile and formate. When cytosine does form, it rapidly decomposes. At room temperature and with a neutral pH, cytosine breaks down, losing half its molecules in 340 years. At 32 °F (0 °C), its half-life is 17,000 years—still too short a time for cytosine to be part of the supposed first self-replicator. To date, scientists have failed to produce cytosine in a spark-discharge experiment, nor has cytosine been recovered from meteorites or extraterrestrial sources. Because meteorites (and other extraterrestrial materials) serve as a proxy for early Earth’s chemistry, the absence of cytosine in these sources would seem to affirm Shapiro’s conclusion. Shapiro also critically analyzed prebiotic simulation experiments that produced the DNA and RNA component adenine. As with cytosine, he showed that adenine formation on early Earth (by currently recognized prebiotic routes) could not reasonably have occurred, for many of the same reasons. Recent work by James Cleaves and Stanley Miller uncovers an additional problem.11 Nucleobases readily react with formaldehyde and acetaldehyde, compounds most certainly present on early Earth, to form both small molecule derivatives and large intractable molecules. Even under mild conditions, these reactions take place so rapidly that they would preferentially occur at the expense of reactions that could lead to RNA. Thus, if nucleobases could form, competing reactions would likely consume them.
Prebiotic cytosine synthesis: A critical analysis and implications for the origin of life 1
The deamination of cytosine and its destruction by other processes such as photochemical reactions place severe constraints on prebiotic cytosine syntheses. If cytosine concentrations are to be maintained on a worldwide basis, then synthesis must be sufficient to replace depletion. The syntheses described thus far do not possess the necessary speed and selectivity to meet this requirement. The use of drying lagoons as a site for prebiotic synthesis has been suggested as a remedy: synthetic rates would be enhanced by greatly increasing the concentration of the reagents. The lagoon suggestion appears geologically implausible, however. All schemes in which cytosine is synthesized locally and distributed globally also are handicapped in that the enormous dilution that takes place when cytosine is released into a global sea offsets any gain in synthetic efficiency.
Prebiotic guanine synthesis
Guanine is one of the four main nucleobases found in the nucleic acids DNA and RNA.
For scientists attempting to understand how the building blocks of RNA originated on Earth, guanine -- the G in the four-letter code of life -- has proven to be a particular challenge. While the other three bases of RNA -- adenine (A), cytosine (C) and uracil (U) -- could be created by heating a simple precursor compound in the presence of certain naturally occurring catalysts, guanine had not been observed as a product of the same reactions. 1
Prebiotic ribose synthesis: A critical analysis
The evidence that is currently available does not support the availability of ribose on the prebiotic earth, except perhaps for brief periods of time, in low concentration as part of a complex mixture, and under conditions unsuitable for nucleoside synthesis. 1
Sugars are very unstable, and easily decompose or react with other chemicals. This counts against any proposed mechanism to concentrate them to useable proportions. 2
Asphalt, Water, and the Prebiotic Synthesis of Ribose, Ribonucleosides, and RNA 3
RNA has been called a “prebiotic chemist’s nightmare” because of its combination of large size, carbohydrate building blocks, bonds that are thermodynamically unstable in water, and overall intrinsic instability.
However, a discontinuous synthesis model is well-supported by experimental work that might produce RNA from atmospheric CO2, H2O, and N2. For example, electrical discharge in such atmospheres gives formaldehyde (HCHO) in large amounts and glycolaldehyde (HOCH2CHO) in small amounts. When rained into alkaline aquifers generated by serpentinizing rocks, these substances were undoubtedly converted to carbohydrates including ribose. Likewise, atmospherically generated HCN was undoubtedly converted in these aquifers to formamide and ammonium formate, precursors for RNA nucleobases. Finally, high reduction potentials maintained by mantle-derived rocks and minerals would allow phosphite to be present in equilibrium with phosphate, mobilizing otherwise insoluble phosphorus for the prebiotic synthesis of phosphite and phosphate esters after oxidation.
So why does the community not view this discontinuous synthesis model as compelling evidence for the RNA-first hypothesis for the origin of life? In part, the model is deficient because no experiments have joined together those steps without human intervention. Further, many steps in the model have problems. Some are successful only if reactive compounds are presented in a specific order in large amounts. Failing controlled addition, the result produces complex mixtures that are inauspicious precursors for biology, a situation described as the “asphalt problem”. Many bonds in RNA are thermodynamically unstable with respect to hydrolysis in water, creating a “water problem”. Finally, some bonds in RNA appear to be “impossible” to form under any conditions considered plausible for early Earth.
A new chicken-and-egg paradox relating to the origin of life
http://www.uncommondescent.com/intelligent-design/do-viruses-help-explain-the-origin-of-life/
Cells could not have evolved without viruses, as they need reverse transcriptase (which is found only in viruses) in order to move from RNA to DNA.
In other words, instead of helping to solve the problem of the origin of life on Earth, recent research has only served to highlight one of its central paradoxes. And yet the science media reports the latest discoveries as if the solution is just around the corner. Don’t you find that just a little strange?
“In order to move from RNA to DNA, you need an enzyme called reverse transcriptase,” Dolja said. “It’s only found in viruses like HIV, not in cells. So how could cells begin to use DNA without the help of a virus?”
https://en.wikipedia.org/wiki/Reverse_transcriptase#In_eukaryotes
Creation of double-stranded DNA occurs in the cytosol as a series of these steps:
A specific cellular tRNA acts as a primer and hybridizes to a complementary part of the virus RNA genome called the primer binding site or PBS
Complementary DNA then binds to the U5 (non-coding region) and R region (a direct repeat found at both ends of the RNA molecule) of the viral RNA
A domain on the reverse transcriptase enzyme called RNAse H degrades the 5’ end of the RNA which removes the U5 and R region
The primer then ‘jumps’ to the 3’ end of the viral genome and the newly synthesised DNA strands hybridizes to the complementary R region on the RNA
The first strand of complementary DNA (cDNA) is extended and the majority of viral RNA is degraded by RNAse H
Once the strand is completed, second strand synthesis is initiated from the viral RNA
There is then another ‘jump’ where the PBS from the second strand hybridizes with the complementary PBS on the first strand
Both strands are extended further and can be incorporated into the hosts genome by the enzyme integrase
Creation of double-stranded DNA also involves strand transfer, in which there is a translocation of short DNA product from initial RNA dependent DNA synthesis to acceptor template regions at the other end of the genome, which are later reached and processed by the reverse transcriptase for its DNA-dependent DNA activity
To date, scientists have failed to produce cytosine in a spark-discharge experiment, nor has cytosine been recovered from meteorites or extraterrestrial sources. Because meteorites (and other extraterrestrial materials) serve as a proxy for early Earth’s chemistry, the absence of cytosine in these sources would seem to affirm Shapiro’s conclusion. Shapiro also critically analyzed prebiotic simulation experiments that produced the DNA and RNA component adenine. As with cytosine, he showed that adenine formation on early Earth (by currently recognized prebiotic routes) could not reasonably have occurred, for many of the same reasons. Recent work by James Cleaves and Stanley Miller uncovers an additional problem. Nucleobases readily react with formaldehyde and acetaldehyde, compounds most certainly present on early Earth, to form both small molecule derivatives and large intractable molecules. Even under mild conditions, these reactions take place so rapidly that they would preferentially occur at the expense of reactions that could lead to RNA. Thus, if nucleobases could form, competing reactions would likely consume them.
https://books.google.com.br/books?id=rlIyVJ9dpzkC&pg=PA149&lpg=PA149&dq=thymidylate+synthase+origin+of+life&source=bl&ots=cocJIaoVZg&sig=VtlcxunaYAkxx4DZwpEjvHnh_-0&hl=en&sa=X&ei=AnyHVYyRA8qy-AG1v4HYBw&ved=0CDAQ6AEwAw#v=onepage&q=thymidylate%20synthase%20origin%20of%20life&f=false

Prebiotic thymine synthesis
Thymidylate synthases (Thy) are key enzymes in the synthesis of deoxythymidylate, 1 of the 4 building blocks of DNA. As such, they are essential for all DNA-based forms of life and therefore implicated in the hypothesized transition from RNA genomes to DNA genomes. Two unrelated Thy enzymes, ThyA and ThyX, are known to catalyze the same biochemical reaction. 7
Thymidylate synthase (Thy) is a fundamental enzyme in DNA synthesis because it catalyzes the formation of deoxythymidine 5′-monophosphate (dTMP) from deoxyuridine 5′-monophosphate (dUMP). For decades, only one family of thymidylate synthase enzymes was known, and its presence was considered necessary to maintain all DNA-based forms of life. Then, a gene encoding an alternative enzyme was discovered and characterized (Dynes and Firtel 1989; Myllykallio et al. 2002), and the novel enzyme was named ThyX, whereas the other enzyme was renamed ThyA. Even though both reactions accomplish the same key step, the reaction mechanisms, or steps, catalyzed by the FDTS and TS enzymes are structurally different.The 2 enzymes, ThyA and ThyX, were found to have distinctly different sequences and structures, thus alluding to independent origins.
Thats interesting, as we find two distinct enzymes with two different sequences and structures synthesizing the same reaction, thus being a example of convergence right in the beginning. How remote was the chance for this to happen by natural means , considering, that convergence does not favour naturalistic explanations ?
as Stephen J.Gould wrote: “…No finale can be specified at the start, none would ever occur a second time in the same way, because any pathway proceeds through thousands of improbable stages. Alter any early event, ever so slightly, and without apparent importance at the time, and evolution cascades into a radically different channel.1
Stephen J. Gould, Wonderful Life: The Burgess Shale and the Nature of History (New York, NY: W.W. Norton & Company, 1989), 51.
By virtue of their function and phyletic distribution, Thys are ancient enzymes, implying 1) the likely participation of one or both enzymes during the transition from an RNA world to a DNA world (based on protein catalysts: Joyce 2002) and 2) the probable presence of a gene encoding Thy in the genome of the common ancestors of eukaryotes, bacteria, and archaea (Penny and Poole 1999; Woese 2002; Koonin 2003; Kurland et al. 2006). Thus, tracing back the pathway of genes encoding ThyA and ThyX may shed light on the actively debated wider issue regarding the origins of viral and cellular DNA
This brings us to the same problem as with Ribonucleotide Reductase enzymes (RNR), which is the classic chicken and egg, catch22 situation. ThyA and ThyX enzymes are required to make DNA. DNA is however required to make these enzymes. What came first ?? We can conclude with high certainty that this enzyme buries any RNA world fantasies, and any possibility of transition from RNA to DNA world scenarios, since both had to come into existence at the same time.
Further implications : There is a third enzyme doing doing the same reaction:
New Chemical Reaction For DNA Production In Bacteria And Viruses Discovered 6
Scientists have discovered a new chemical reaction for producing one of the four nucleotides, or building blocks, needed to build DNA. The reaction includes an unusual first step, or mechanism, and unlike other known reactions that produce the DNA building block, uses an enzyme that speeds up, or catalyzes, the reaction without bonding to any of the compounds, or substrates, in the reaction.
The chemical reaction discovered by the researchers uses an enzyme called flavin-dependent thymidylate synthase, or FDTS. The enzyme is coded by the thyX gene and has been found primarily in bacteria and viruses, including several human pathogens and biological warfare agents.
Both the new and classical enzymatic reactions complete a key step in producing the DNA building block by adding a methyl group--one carbon atom attached to three hydrogen atoms--to the building block's precursor molecule called deoxy-uridine monophosphate, or dUMP.
Thymidylate synthase (Thy) is a fundamental enzyme in DNA synthesis because it catalyzes the formation of deoxythymidine 5′-monophosphate (dTMP) from deoxyuridine 5′-monophosphate (dUMP). For decades, only one family of thymidylate synthase enzymes was known, and its presence was considered necessary to maintain all DNA-based forms of life. Then, a gene encoding an alternative enzyme was discovered and characterized (Dynes and Firtel 1989; Myllykallio et al. 2002), and the novel enzyme was named ThyX, whereas the other enzyme was renamed ThyA. Even though both reactions accomplish the same key step, the reaction mechanisms, or steps, catalyzed by the FDTS and TS enzymes are structurally different.The 2 enzymes, ThyA and ThyX, were found to have distinctly different sequences and structures, thus alluding to independent origins.
Thats interesting, as we find two distinct enzymes with two different sequences and structures synthesizing the same reaction, thus being a example of convergence right in the beginning. How remote was the chance for this to happen by natural means , considering, that convergence does not favour naturalistic explanations ?
as Stephen J.Gould wrote: “…No finale can be specified at the start, none would ever occur a second time in the same way, because any pathway proceeds through thousands of improbable stages. Alter any early event, ever so slightly, and without apparent importance at the time, and evolution cascades into a radically different channel.1
Stephen J. Gould, Wonderful Life: The Burgess Shale and the Nature of History (New York, NY: W.W. Norton & Company, 1989), 51.
By virtue of their function and phyletic distribution, Thys are ancient enzymes, implying 1) the likely participation of one or both enzymes during the transition from an RNA world to a DNA world (based on protein catalysts: Joyce 2002) and 2) the probable presence of a gene encoding Thy in the genome of the common ancestors of eukaryotes, bacteria, and archaea (Penny and Poole 1999; Woese 2002; Koonin 2003; Kurland et al. 2006). Thus, tracing back the pathway of genes encoding ThyA and ThyX may shed light on the actively debated wider issue regarding the origins of viral and cellular DNA
This brings us to the same problem as with Ribonucleotide Reductase enzymes (RNR), which is the classic chicken and egg, catch22 situation. ThyA and ThyX enzymes are required to make DNA. DNA is however required to make these enzymes. What came first ?? We can conclude with high certainty that this enzyme buries any RNA world fantasies, and any possibility of transition from RNA to DNA world scenarios, since both had to come into existence at the same time.
Further implications : There is a third enzyme doing doing the same reaction:
New Chemical Reaction For DNA Production In Bacteria And Viruses Discovered 6
Scientists have discovered a new chemical reaction for producing one of the four nucleotides, or building blocks, needed to build DNA. The reaction includes an unusual first step, or mechanism, and unlike other known reactions that produce the DNA building block, uses an enzyme that speeds up, or catalyzes, the reaction without bonding to any of the compounds, or substrates, in the reaction.
The chemical reaction discovered by the researchers uses an enzyme called flavin-dependent thymidylate synthase, or FDTS. The enzyme is coded by the thyX gene and has been found primarily in bacteria and viruses, including several human pathogens and biological warfare agents.
Both the new and classical enzymatic reactions complete a key step in producing the DNA building block by adding a methyl group--one carbon atom attached to three hydrogen atoms--to the building block's precursor molecule called deoxy-uridine monophosphate, or dUMP.

1) http://www.pnas.org/content/96/8/4396.full
2) http://www.trueorigin.org/originoflife.php
3) http://www.talkorigins.org/faqs/abioprob/originoflife.html
4) http://www.evolutionnews.org/2009/07/scientists_say_intelligent_des022621.html
5) http://www.rsc.org/chemistryworld/News/2009/May/13050902.asp
6) http://www.sciencedaily.com/releases/2009/04/090416161133.htm
7) http://www.ncbi.nlm.nih.gov/pubmed/20525631
1) http://www.ncbi.nlm.nih.gov/pubmed/11536683?dopt=Abstract
2) Hugh Ross & Fazale Rana, Origins of life pg.79
3) http://www.pnas.org/content/104/44/17272.full
1) http://www.ncbi.nlm.nih.gov/pubmed/2453009
2) http://creationwiki.org/Origin_of_life
3) http://pubs.acs.org/doi/abs/10.1021/ar200332w
further readings :
https://www.c4id.org.uk/index.php?option=com_content&view=article&id=211:the-problem-of-the-origin-of-life&catid=50:genetics&Itemid=43
http://www.ncbi.nlm.nih.gov/books/NBK6360/figure/A43731/?report=objectonly
Metabolic pathways for RNA and DNA precursors biosynthesis: a palimpsest from the RNA to DNA world transition? The biosynthetic pathways for purine and pyrimidine nucleotides both start with ribose 5-monophosphate. The formation of the four bases requires several amino-acids, formate and carbamyl-phosphate. Nucleotide monophosphates (NMP) are converted into RNA precursors (NTP) by NMP kinases (k) and NDP kinases (K). These reactions probably are relics of the RNA-protein world. DNA precursors are produced from NDP and/or NTP by ribonucleotide reductases (RNR), except for dTTP, which results from methylation of dUMP. dTMP is produced from dUMP by Thymidylate synthases (ThyA or ThyX) and converted into dTTP by the same kinases that convert NMP into NTP. dUMP can be produced either by dUTPAse or by dCTP deaminase. In the U-DNA world, it could have been also produced by degradation of U-DNA. The mode of dTMP production clearly suggests that U-DNA was an evolutionary intermediate between RNA and T-DNA. Some viruses contain U-DNA, whereas others contain HMC-DNA (HMC= hydroxymethyl-cytosine). Transformation of C into HMC occurs at the level of dCMP, and conversion of dCMP into dHMCMP is catalyzed by a dCMP hydroxy-methyl transferase (dCMP HM transferase), which is homologue to ThyA (See refs. 11, 14, and 19 for more details).
[url=https://servimg.com/view/17307623/802][img]https://i.servimg.com/u/f18/17/30/76/23/ch314f1
Last edited by Admin on Wed Feb 27, 2019 5:47 pm; edited 1 time in total