Collagen, the most abundant protein in the human body, and its synthesis
Collagens are major components of the extracellular matrices of all metazoan life and play crucial roles in developmental processes and tissue homeostasis 10 Inhibition of the synthesis of either of these classes of collagen in Hydra, a simple diploblastic organism, results in a failure of tissue regeneration after injury. Mutations in similar genes in man are either incompatible with life or result in a number of severe diseases including osteogenesis imperfecta, aortic aneurysms, Alports syndrome and even some forms of cancer. The collagen molecules provide strong and supportive extracellular scaffolds or assemblies as well as contributing other important properties such as defined interaction sites for cells and macromolecules. Collagens are composed of three polypeptide chains (alpha chains) that fold together to form the characteristic triple helical collagenous domain
Collagen is the main structural protein in the extracellular space in the various connective tissues in animal bodies. As the main component of connective tissue, it is the most abundant protein in mammals, making up from 25% to 35% of the whole-body protein content. Depending upon the degree of mineralization, collagen tissues may be rigid (bone), compliant (tendon), or have a gradient from rigid to compliant (cartilage). Collagen, in the form of elongated fibrils, is mostly found in fibrous tissues such as tendons, ligaments and skin. It is also abundant in corneas, cartilage, bones, blood vessels, the gut, intervertebral discs, and the dentin in teeth. In muscle tissue, it serves as a major component of the endomysium. Collagen constitutes one to two percent of muscle tissue, and accounts for 6% of the weight of strong, tendinous muscles. The fibroblast is the most common cell that creates collagen. 1
Collagen molecules are made up of three poly- peptide chains intertwined to form the collagen triple helix. 5 The helix requires a highly specific amino acid composition in order to form. In essence, the single polypeptides of collagen are polyproline chains with glycine amino acids interspersed every third position. Because of their small size, the glycine residues allow the polyproline chains to twist into a helical conformation. Collagen molecules will spontaneously aggregate into fibrils, so they are produced inside the cell in a form that will not self-assemble. This form, pro-collagen, has large globular ends that prevent association of collagen chains. Once produced, the collagen is secreted to the cell's exterior. Then, an enzyme called collagenase cleaves the globular ends from the pro-collagen to form protein chains that can readily aggregate. Because of collagen's key structural role in animal tissues, biochemists long thought this biomolecule was stable at body temperatures for warm-blooded creatures and at environmental temperatures for cold-blooded animals. To nearly everyone's surprise, however, recent work indicates that the collagen triple helix and fibrils are unstable at body temperatures with the chains spontaneously unfolding from the triple helix arrangement into random coils. Even more surprising is the fact that this instability seems carefully orchestrated by precise adjustments to the collagen amino acid composition.
Biochemists think the instability of the collagen triple helix plays an important role in the assembly of fibrils. The unfolded regions of the collagen triple helix promote the lateral association of the collagen triple helices into the higher order structure of the fibrils. If the triple helix was stable, these associations could not occur. Once the fibrils form, the mutual interaction among the collagen molecules stabilizes the triple helix conformation. The tendency of the collagen helices to unfold also imparts collagen fibrils with elasticity. This property makes the tissues that form tendons and ligaments flexible and able to withstand stresses and strains. Finally, the unfolded regions are also sites where collagenase enzymes can break down collagen molecules and hence, collagen fibrils. This breakdown allows tissues to undergo dynamic restructuring as animals face ever-chang- ing demands (e.g., due to growth). The exacting amino acid composition imparts collagen with strength and elasticity and allows biochemical machinery to resculpture it as needed.
Collagen is one of the most plentiful proteins. It’s an important structural component of many tissues, not the least of which is skin. 3 Composed of three strands of rope-like polymers, it provides both strength and flexibility to skin and other tissues. Sometimes collagen fibrils need to be disassembled, however, and that’s the job of collagenase. Providentially, collagen comes with joints called “cleavage sites” where the strands can be easily broken by the enzymes for quick recycling. The collagenase motor clings like an ascender to a rope and walks up the collagen fiber to the nearest cleavage site. Self-propelled by Brownian motion, it goes into action splitting the fibrils as needed. What keeps it from falling back down? Without a ratchet or clutch mechanism, all the motor could hope for when subjected to random forces is symmetric back and forth motion on the cable. Collagenase, it turns out, uses a strategy called the “burnt bridge” technique.
If you were on a monorail buffeted by random winds, but needed to get somewhere, you could avoid rolling backward, away from your destination, by burning your bridges behind you. Assuming your technique braked any backward motion (rather than making you fall off), this strategy would result in a net forward “propulsion.” The collagenase motor does this by “digesting” the fiber after it passes by, preventing backward motion, and allowing the next gust of thermal energy to propel it forward. Wouldn’t this result in traffic jams, when multiple motors bunch up against cleavage sites? No; the motors can switch to nearby collagen fibers. “Relief of the traffic jam is achieved by the transfer of the trapped enzyme to new tracks,” the authors explain. The research team found that this form of propulsion is about 15% efficient but costs no ATP. As a result, collagenase works its way along the fiber at about 4.5 micrometers per second. On a molecular scale, that’s scootin’. On our scale, that would translate to about a thousand miles an hour. Imagine moving that fast on a highway with no gasoline, extracting energy from the road! Senior author Dr. Gregory Goldberg expressed some wonder at this mechanism used by collagenase: “with our model, a whole new principle emerges in which molecular motors in the extracellular matrix operate by extracting energy from the very track they move upon.” What does this mean to you and me? “By digesting collagen, enzymes such as MMP-1 initiate tissue remodeling, which can have a variety of purposes from organ development to tissue repair to metastatic invasion of tumors.”
Molecular motor implicated in tissue remodeling 4
MMPs regulate cell behavior through finely tuned and tightly controlled proteolytic processing of a large variety of signaling molecules that can also have beneficial effects in disease resolution. 6 Moreover, net proteolytic activity relies upon direct interactions between the different protease and protease inhibitor families, interconnected in a complex protease web, with MMPs acting as key nodal components. Further studies then showed that beyond their collagen-degrading functions, MMPs degrade and process many other components of the extracellular matrix (ECM), including the basement membrane. The ECM is a complex mesh of structural and signaling molecules that provides dynamic support to cells and tissues as well as harboring embedded information that modulates cell behavior. Numerous reports have shown that MMPs are directly implicated in almost every biological process involving matrix remodeling throughout the mammalian life span, from embryo implantation [6] to cell death or necrosis. But at what level is their role? For instance, MMP-driven proteolysis has been linked to several morphogenetic events during development, including mammary gland ductal branching, bone ossification and blood vessel remodeling. As well, MMPs play a primary role in normal tissue maintenance functions, including wound healing and repair, menstruation and reproductive processes, and innate immune defense.
As a consequence, altered expression and/or misregulation of MMP activities in vivo has been associated with the development of a wide range of pathologies, including chronic inflammatory diseases and cancer. Recent data have shown more complex roles for MMPs in the direct regulation of a host of signaling molecules, including most and likely all of the 54 chemokines in man, and hence the function of many cells of the stroma and innate immune system.
Question: Is that not indication that a gradual stepwise emergence of MMP would only produce non-functional intermediates, since altered expression and/or misregulation of MMP activities in vivo has been associated with the development of a wide range of pathologies, including chronic inflammatory diseases and cancer ?

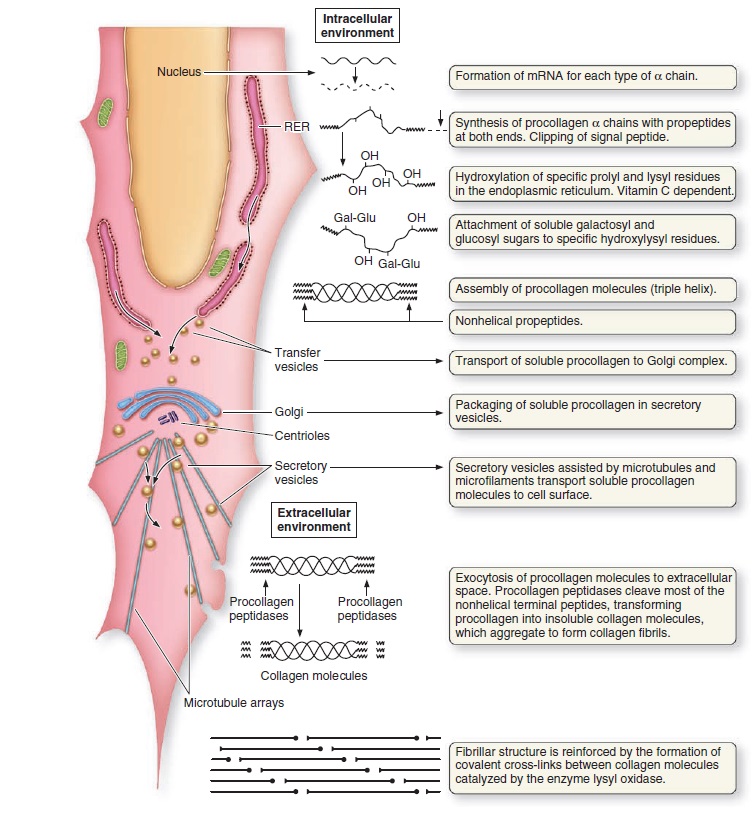
Hydroxylation and glycosylation of procollagen α chains and their assembly into triple helices occur in the RER, and further assembly into fibrils occurs in the ECM after secretion of procollagen. Because there are many slightly different genes for procollagen α chains and collagen production depends on several posttranslational events involving several other enzymes, many diseases involving defective collagen synthesis have been described.
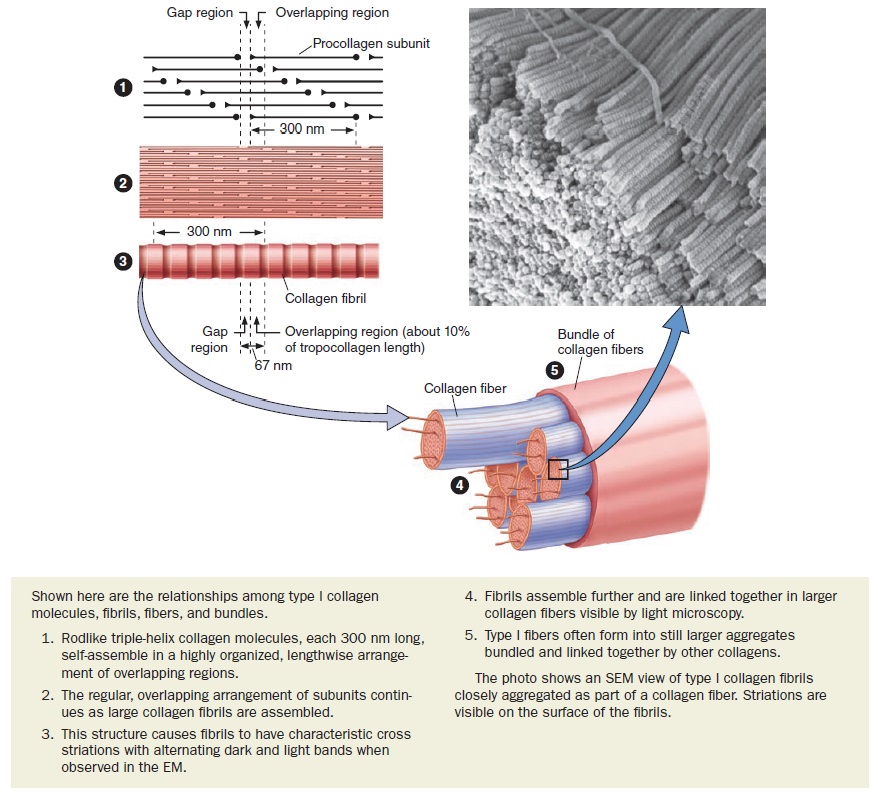
Collagens Are the Major Proteins of the Extracellular Matrix 2
The primary feature of a typical collagen molecule is its long, stiff, triple- stranded helical structure, in which three collagen polypeptide chains, called α chains, are wound around one another in a ropelike superhelix
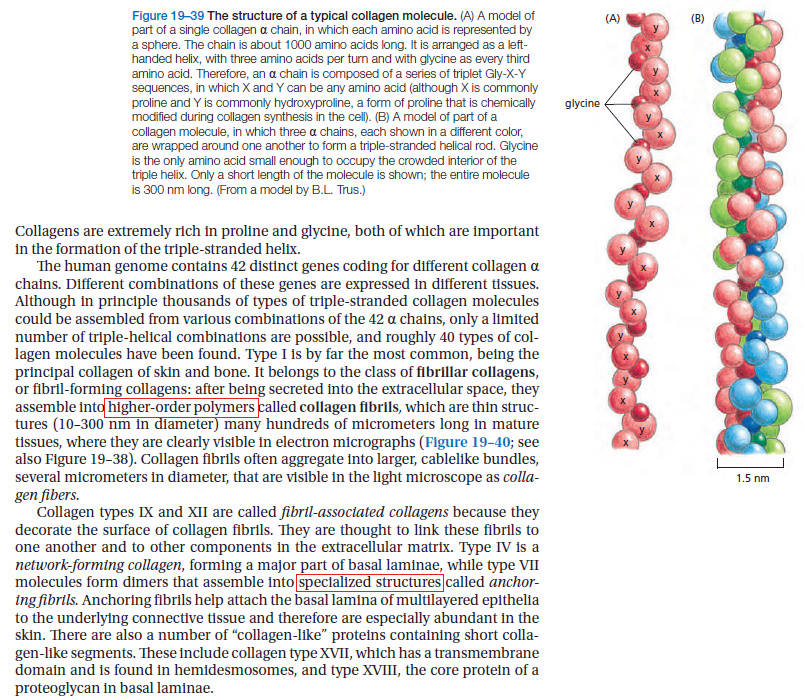
Fibroblasts may have a similar role in organizing the extracellular matrix inside the body. First they synthesize the collagen fibrils and deposit them in the correct orientation. Then they work on the matrix they have secreted, crawling over it and tugging on it so as to create tendons and ligaments and the tough, dense layers of connective tissue that surround and bind together most organs.
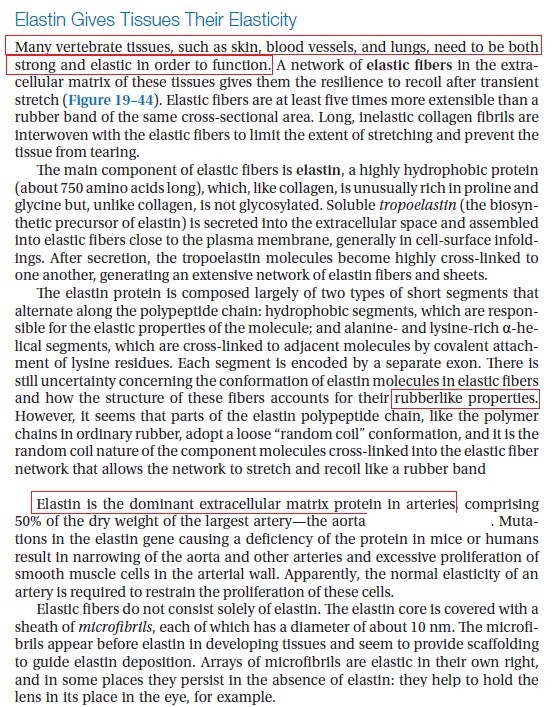
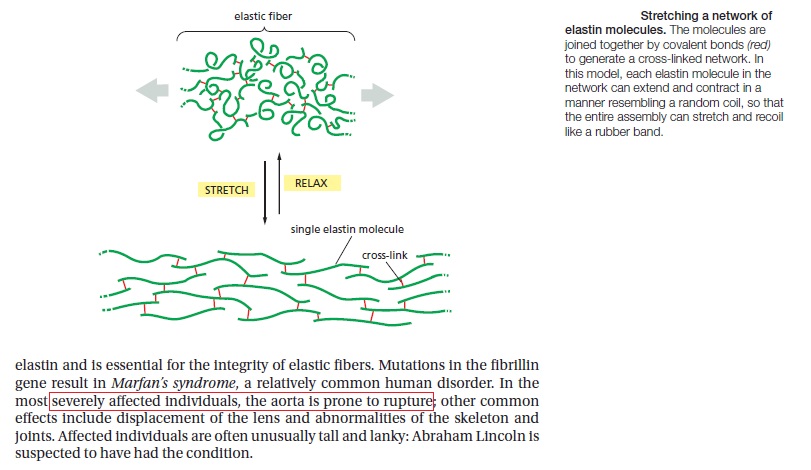
Evolution of Collagen
Fibril-forming (fibrillar) collagens are extracellular matrix proteins conserved in all multicellular animals. 10 Vertebrate members of the fibrillar collagen family are essential for the formation of bone and teeth, tissues that characterise vertebrates. The potential role played by fibrillar collagens in vertebrate evolution has not been considered previously largely because the family has been around since the sponge and it was unclear precisely how and when those particular members now found in vertebrates first arose.
Collagen cross-linking: insights on the evolution of metazoan extracellular matrix 7
Collagens constitute a large family of extracellular matrix (ECM) proteins that play a fundamental role in supporting the structure of various tissues in multicellular animals. The mechanical strength of fibrillar collagens is highly dependent on the formation of covalent cross-links between individual fibrils, a process initiated by the enzymatic action of members of the lysyl oxidase (LOX) family. Fibrillar collagens are present in a wide variety of animals, therefore often being associated with metazoan evolution, where the emergence of an ancestral collagen chain has been proposed to lead to the formation of different clades. While LOX-generated collagen cross-linking metabolites have been detected in different metazoan families, there is limited information about when and how collagen acquired this particular modification. By analyzing telopeptide and helical sequences, we identified highly conserved, potential cross-linking sites throughout the metazoan tree of life. Based on this analysis, we propose that they have importantly contributed to the formation and further expansion of fibrillar collagens.
How are collagens synthesized?
Collagen biosynthesis is complex, involving numerous intracellular and extracellular steps, all of which contribute to the structure and biomechanical properties of the final fibrils. The first event following synthesis of procollagen chains on the ribosome is their import into the rough endoplasmic reticulum. There they undergo a series of post-translational modifications resulting in the assembly of procollagen molecules (Fig. 2.4)

These steps include
modification of proline residues to hydroxyprolines,
modification of lysines to hydroxylysines,
N- and O-linked glycosylation,
trimerization,
disulfide bonding,
prolyl cis–trans isomerization and folding of the triple helix.
Molecules then transit the Golgi network where they are packaged into secretory vesicles prior to export into the extracellular matrix.
Procollagen processing occurs during or shortly after secretion followed by assembly of fibrils. Finally, fibrils are stabilized by the formation of covalent cross-links.
Post-translationalModifications of Polypeptide Chains
It has long been known that stability of the collagen triple helix is related to the total content of the amino acids proline and hydroxyproline, which together make up about 20% of the total amino acids in human fibrillar collagens. This is a result of the cyclic nature of the side chains that restrict flexibility about the peptide bond. Prolyl hydroxylation provides further stabilization, as shown by the approximately 30◦C drop in melting temperature when this process is prevented. Hydroxylation occurs mainly on prolines in the Y position of the Gly-X-Y triplet, through the action of prolyl 4-hydroxylases (P4H), and also to a much lesser extent on prolines in the X-position, through the action of prolyl 3-hydroxylases (P3H).
Prolyl 4-hydroxylase The collagen prolyl 4-hydroxylases (P4Hs), enzymes residing within the endoplasmic reticulum, have a central role in the biosynthesis of collagens. 11 The best known role for Hyp is in stabilizing the collagen triple helix
Prolyl 3-hydroxylation is a rare but conserved post-translational modification in many collagen types and, when defective, may be linked to a number of human diseases with musculoskeletal and potentially ocular and renal pathologies. Prolyl 3-hydroxylase-1 (P3H1), the enzyme responsible for converting proline to 3-hydroxyproline (3Hyp) in type I collagen, requires the coenzyme CRTAP for activity. 11
The enzymes responsible are 2-oxoglutarate and non-heme-Fe(II)-dependent dioxygenases, which require Fe2+, 2-oxoglutarate, O2 and ascorbate for activity. In humans, three forms of P4H and three forms of P3H have been described. P4H is a tetramer consisting of two subunits and two subunits. The subunit is common to all forms of the enzyme and also exists as an isolated protein with both enzyme and chaperone activity, protein disulfide isomerase (PDI). Different forms of P4H therefore differ in the subunit, which shows tissue specificity, the type II enzyme being the major form in chondrocytes, osteoblasts and endothelial cells. While prolyl hydroxylation in the Y position is the norm, hydroxylation of prolines in the X-position is much less common, there being only one such residue per chain in collagens I and II, in contrast to collagen IV which has several. Nevertheless, this modification performs a vital function, as recently shown by loss of prolyl 3-hydroxylase activity which leads to a recessive metabolic bone disorder resembling lethal/severe osteogenesis imperfecta. It should be noted that for steric reasons, prolyl hydroxylation occurs only prior to assembly of the triple-helical procollagen molecule.
Following transcription, collagen synthesis starts with the translation of the alpha chain mRNAs on the rough ER. As the growing a chains are translocated into the lumen of the ER, the collagenous domains are post-translationally modified by hydroxylation of peptidyl prolyl and lysyl residues to form hydroxyproline and hydroxylysine respectively. In addition, some hydroxylysine residues are glycosylated with galactose and glucose residues. Once the full-length a chain has been translocated into the ER lumen, the carboxyl terminal noncollagenous (NC1) domain folds. Correctly folded NC1 domains select appropriate partners and trimerize bringing the three a chain collagenous domains together in register. The triple helix nucleates at its carboxyl terminal end allowing the complete collagenous triple helical domain to subsequently fold or ‘zip up’ in the C-to-N direction (Fig. 1). Upon secretion, collagen molecules assemble to form macromolecular aggregates. For fibril-forming or ‘fibrillar’ collagens, which are the focus of this article, the mechanisms controlling macromolecular assembly are relatively well understood (in vertebrate systems—Fig. 1). A full-length fibrillar collagen molecule, or ‘procollagen’, consists of the C-terminal NC1 domain (C-propeptide), a collagenous domain with approximately 337–340 Gly-X-Y repeats (major helix), and an Npropeptide consisting of a short triple helical domain (minor helix) and a non-collagenous NC2 domain. The N- and C propeptides are highly soluble and keep the insoluble collagenous domain in solution. As the fibrillar procollagen is secreted from the cell, specific enzymes (C- and N-proteinases) cleave the propeptides from the collagenous domains. The dramatic drop in solubility causes the collagenous domains to laterally aggregate with a 1 4 = 1 3 staggers to the overlap regions. Lysyl oxidase catalyzes covalent cross linking between adjacent collagen molecules stabilizing the fibril (Fig. 1). More detailed accounts of collagen synthesis and assembly are given in other recent reviews.
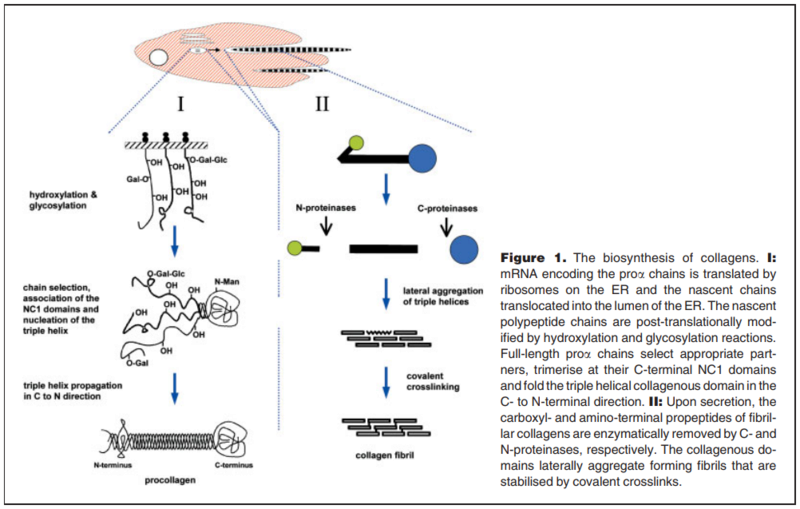
Collagens constitute a large family of extracellular matrix (ECM) proteins that play a fundamental role in providing the structural integrity and biomechanical properties of different tissues. In vertebrates, 28 types of collagens have been described (I-XXVIII), which are divided into several families, the most important being fibrillar collagens (I-III, V, XI, XXIV, and XXVII) and basement membrane-forming collagen IV2.
The biosynthesis of collagen is a highly complicated process involving numerous steps, including chain association and folding, secretion, procollagen processing and cross-linking
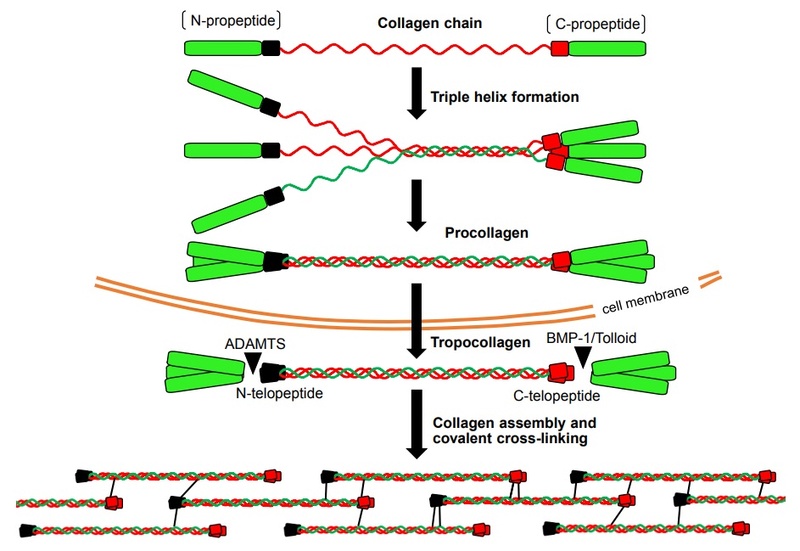
As exemplified for human type I collagen, a heterotrimeric molecule composed of two α1 and one α2 chain, after synthesis on the ribosome and their import into the rough endoplasmic reticulum, collagen chains are subjected to a series of post-translational modifications resulting in the assembly of procollagen chains. These include hydroxylation of specific proline residues, catalyzed by prolyl-4- and prolyl-3-hydroxylases (P4H and P3H enzymes); hydroxylation of specific lysine residues by lysyl hydroxylases (encoded in human by PLOD1-3 genes); N- and O-linked glycosylation, disulfide bonding and prolyl cis-trans isomerization. Association of the three α-chains occurs through a process governed by the C-terminus, and the formation of the triple helix is propagated towards the N-terminal end in a zipper-like fashion to form the procollagen molecule. This precursor molecule is then transported to the Golgi network where it is packaged into specialized secretory vesicles prior to export into the extracellular medium. Formation of fibrils from procollagen chains requires their proteolytic processing. The N- and C-propeptides are cleaved off by metalloproteinases belonging to the ADAMTS (a disintegrin and metalloproteinase with thrombospondin motifs) and BMP1 (bone morphogenetic protein 1)/Tolloid-like families, respectively, yielding the tropocollagen molecule, which retains a short portion of the propeptides termed telopeptides. Then, lysine or hydroxylysine residues within these non-collagenous domains are oxidatively deaminated by LOX, yielding the corresponding aldehydes which constitute the initiation products for the cross-linking formation. Within hours of helix formation, these telopeptide aldehydes spontaneously react with helical lysines or hydroxylysines to form immature cross-links, which further react among them and with remaining lysine or hydroxylysine residues over months/years to form permanent cross-links. The formation of these permanent or mature cross-links is fundamental as they determine the topology of adjacent molecules and contribute to the stiffness of the collagen fibril, where variations in the usage of lysine or hydroxylysine in both telopeptide and helix sites modulate the mechanical properties of the collagen matrix. In fact, defects in PLOD2, the lysyl hydroxylase isoform that specifically acts on lysine residues in collagen telopeptides, are responsible for Brück syndrome, a heritable disorder in the osteogenesis imperfecta spectrum, characterized by severe reduction or elimination of the telopeptide hydroxylysine-derived cross-links, resulting in bone fragility. In addition to lysyl hydroxylases, collagen-associated proteins such as the small leucine-rich protein (SLRP), fibromodulin, have been shown to influence collagen cross-linking, as recently evidenced in fibromodulin-deficient mice.
As cDNA and genomic sequences became available, it became increasingly evident that many ECM genes such as collagens and laminins are very ancient, and, in the last decade, as genomic sequences were determined for many metazoa, it was recognized that many ECM-encoding genes originated early in metazoan evolution. 9
The collagen architecture is the major determinant of the function and mechanical behavior of cardiovascular tissues. 8 Different collagen architectures, including collagen organization, cross-linking and collagen content, result in variation in biomechanical behavior between and within tissues.
1. https://en.wikipedia.org/wiki/Collagen
2. Molecular biology of the cell, 6th edition, page 1061
3. http://creationsafaris.com/crev200410.htm
4. https://www.eurekalert.org/pub_releases/2004-09/wuso-mmi093004.php
5. Fazale Rana, Cell's design , page 117
6. http://www.sciencedirect.com/science/article/pii/S0167488909002407
7. https://www.nature.com/articles/srep37374
8. http://journals.plos.org/plosone/article?id=10.1371/journal.pone.0127847
9. http://jcb.rupress.org/content/196/6/671
10. http://www.marisolcollazos.es/Sociologia-complemento/Articulos/Molecular_Incesto.pdf
11. https://www.ncbi.nlm.nih.gov/pmc/articles/PMC3995746/
12. http://www.sciencedirect.com/science/article/pii/S0945053X03000064
Collagens are major components of the extracellular matrices of all metazoan life and play crucial roles in developmental processes and tissue homeostasis 10 Inhibition of the synthesis of either of these classes of collagen in Hydra, a simple diploblastic organism, results in a failure of tissue regeneration after injury. Mutations in similar genes in man are either incompatible with life or result in a number of severe diseases including osteogenesis imperfecta, aortic aneurysms, Alports syndrome and even some forms of cancer. The collagen molecules provide strong and supportive extracellular scaffolds or assemblies as well as contributing other important properties such as defined interaction sites for cells and macromolecules. Collagens are composed of three polypeptide chains (alpha chains) that fold together to form the characteristic triple helical collagenous domain
Collagen is the main structural protein in the extracellular space in the various connective tissues in animal bodies. As the main component of connective tissue, it is the most abundant protein in mammals, making up from 25% to 35% of the whole-body protein content. Depending upon the degree of mineralization, collagen tissues may be rigid (bone), compliant (tendon), or have a gradient from rigid to compliant (cartilage). Collagen, in the form of elongated fibrils, is mostly found in fibrous tissues such as tendons, ligaments and skin. It is also abundant in corneas, cartilage, bones, blood vessels, the gut, intervertebral discs, and the dentin in teeth. In muscle tissue, it serves as a major component of the endomysium. Collagen constitutes one to two percent of muscle tissue, and accounts for 6% of the weight of strong, tendinous muscles. The fibroblast is the most common cell that creates collagen. 1
Collagen molecules are made up of three poly- peptide chains intertwined to form the collagen triple helix. 5 The helix requires a highly specific amino acid composition in order to form. In essence, the single polypeptides of collagen are polyproline chains with glycine amino acids interspersed every third position. Because of their small size, the glycine residues allow the polyproline chains to twist into a helical conformation. Collagen molecules will spontaneously aggregate into fibrils, so they are produced inside the cell in a form that will not self-assemble. This form, pro-collagen, has large globular ends that prevent association of collagen chains. Once produced, the collagen is secreted to the cell's exterior. Then, an enzyme called collagenase cleaves the globular ends from the pro-collagen to form protein chains that can readily aggregate. Because of collagen's key structural role in animal tissues, biochemists long thought this biomolecule was stable at body temperatures for warm-blooded creatures and at environmental temperatures for cold-blooded animals. To nearly everyone's surprise, however, recent work indicates that the collagen triple helix and fibrils are unstable at body temperatures with the chains spontaneously unfolding from the triple helix arrangement into random coils. Even more surprising is the fact that this instability seems carefully orchestrated by precise adjustments to the collagen amino acid composition.
Biochemists think the instability of the collagen triple helix plays an important role in the assembly of fibrils. The unfolded regions of the collagen triple helix promote the lateral association of the collagen triple helices into the higher order structure of the fibrils. If the triple helix was stable, these associations could not occur. Once the fibrils form, the mutual interaction among the collagen molecules stabilizes the triple helix conformation. The tendency of the collagen helices to unfold also imparts collagen fibrils with elasticity. This property makes the tissues that form tendons and ligaments flexible and able to withstand stresses and strains. Finally, the unfolded regions are also sites where collagenase enzymes can break down collagen molecules and hence, collagen fibrils. This breakdown allows tissues to undergo dynamic restructuring as animals face ever-chang- ing demands (e.g., due to growth). The exacting amino acid composition imparts collagen with strength and elasticity and allows biochemical machinery to resculpture it as needed.
Collagen is one of the most plentiful proteins. It’s an important structural component of many tissues, not the least of which is skin. 3 Composed of three strands of rope-like polymers, it provides both strength and flexibility to skin and other tissues. Sometimes collagen fibrils need to be disassembled, however, and that’s the job of collagenase. Providentially, collagen comes with joints called “cleavage sites” where the strands can be easily broken by the enzymes for quick recycling. The collagenase motor clings like an ascender to a rope and walks up the collagen fiber to the nearest cleavage site. Self-propelled by Brownian motion, it goes into action splitting the fibrils as needed. What keeps it from falling back down? Without a ratchet or clutch mechanism, all the motor could hope for when subjected to random forces is symmetric back and forth motion on the cable. Collagenase, it turns out, uses a strategy called the “burnt bridge” technique.
If you were on a monorail buffeted by random winds, but needed to get somewhere, you could avoid rolling backward, away from your destination, by burning your bridges behind you. Assuming your technique braked any backward motion (rather than making you fall off), this strategy would result in a net forward “propulsion.” The collagenase motor does this by “digesting” the fiber after it passes by, preventing backward motion, and allowing the next gust of thermal energy to propel it forward. Wouldn’t this result in traffic jams, when multiple motors bunch up against cleavage sites? No; the motors can switch to nearby collagen fibers. “Relief of the traffic jam is achieved by the transfer of the trapped enzyme to new tracks,” the authors explain. The research team found that this form of propulsion is about 15% efficient but costs no ATP. As a result, collagenase works its way along the fiber at about 4.5 micrometers per second. On a molecular scale, that’s scootin’. On our scale, that would translate to about a thousand miles an hour. Imagine moving that fast on a highway with no gasoline, extracting energy from the road! Senior author Dr. Gregory Goldberg expressed some wonder at this mechanism used by collagenase: “with our model, a whole new principle emerges in which molecular motors in the extracellular matrix operate by extracting energy from the very track they move upon.” What does this mean to you and me? “By digesting collagen, enzymes such as MMP-1 initiate tissue remodeling, which can have a variety of purposes from organ development to tissue repair to metastatic invasion of tumors.”
Molecular motor implicated in tissue remodeling 4
MMPs regulate cell behavior through finely tuned and tightly controlled proteolytic processing of a large variety of signaling molecules that can also have beneficial effects in disease resolution. 6 Moreover, net proteolytic activity relies upon direct interactions between the different protease and protease inhibitor families, interconnected in a complex protease web, with MMPs acting as key nodal components. Further studies then showed that beyond their collagen-degrading functions, MMPs degrade and process many other components of the extracellular matrix (ECM), including the basement membrane. The ECM is a complex mesh of structural and signaling molecules that provides dynamic support to cells and tissues as well as harboring embedded information that modulates cell behavior. Numerous reports have shown that MMPs are directly implicated in almost every biological process involving matrix remodeling throughout the mammalian life span, from embryo implantation [6] to cell death or necrosis. But at what level is their role? For instance, MMP-driven proteolysis has been linked to several morphogenetic events during development, including mammary gland ductal branching, bone ossification and blood vessel remodeling. As well, MMPs play a primary role in normal tissue maintenance functions, including wound healing and repair, menstruation and reproductive processes, and innate immune defense.
As a consequence, altered expression and/or misregulation of MMP activities in vivo has been associated with the development of a wide range of pathologies, including chronic inflammatory diseases and cancer. Recent data have shown more complex roles for MMPs in the direct regulation of a host of signaling molecules, including most and likely all of the 54 chemokines in man, and hence the function of many cells of the stroma and innate immune system.
Question: Is that not indication that a gradual stepwise emergence of MMP would only produce non-functional intermediates, since altered expression and/or misregulation of MMP activities in vivo has been associated with the development of a wide range of pathologies, including chronic inflammatory diseases and cancer ?

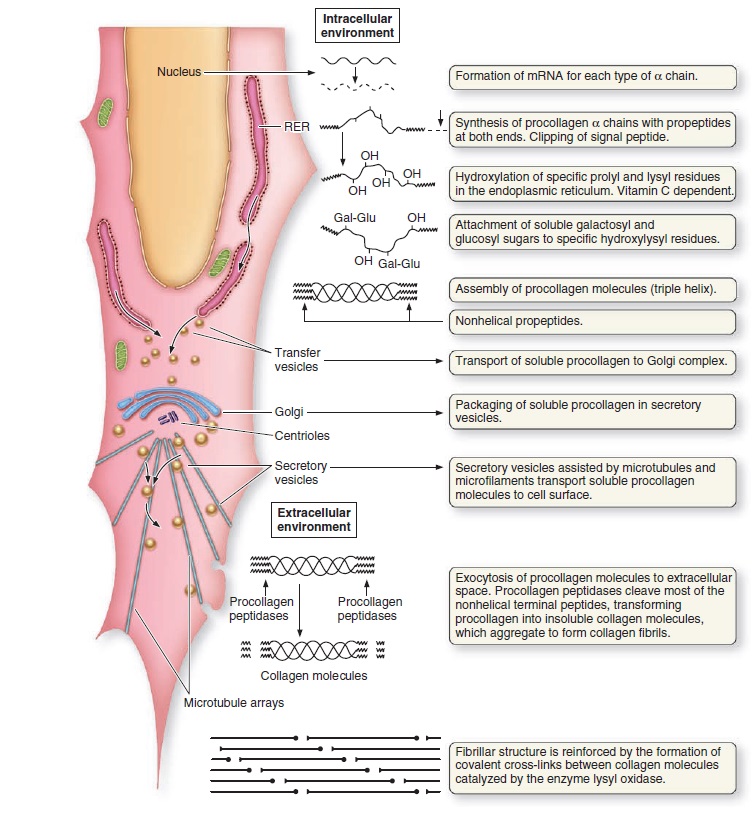
Hydroxylation and glycosylation of procollagen α chains and their assembly into triple helices occur in the RER, and further assembly into fibrils occurs in the ECM after secretion of procollagen. Because there are many slightly different genes for procollagen α chains and collagen production depends on several posttranslational events involving several other enzymes, many diseases involving defective collagen synthesis have been described.
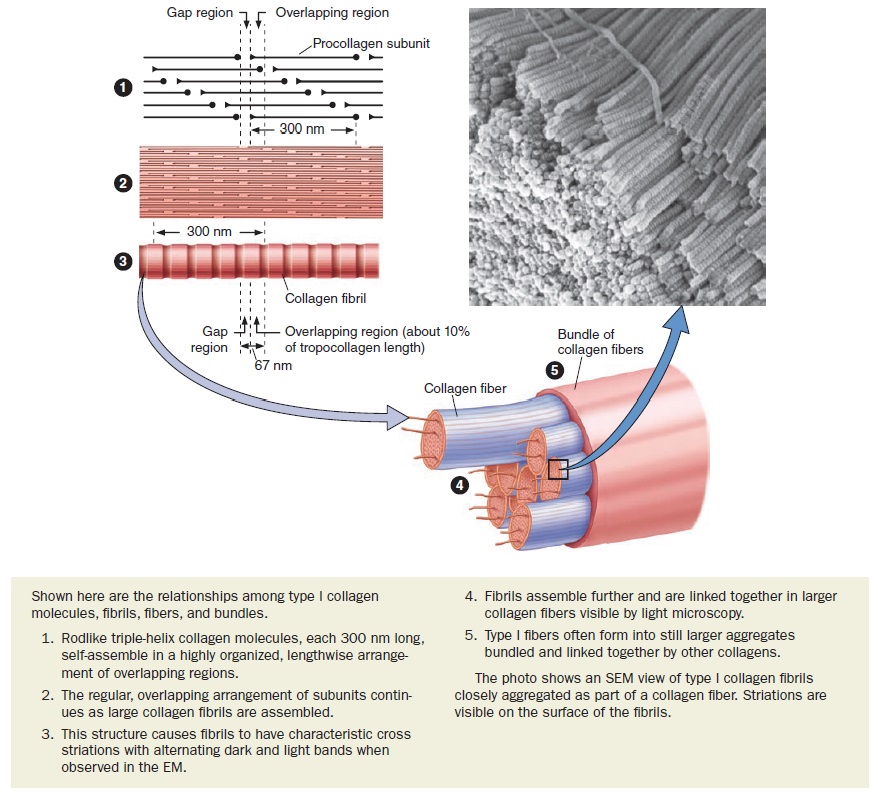
Collagens Are the Major Proteins of the Extracellular Matrix 2
The primary feature of a typical collagen molecule is its long, stiff, triple- stranded helical structure, in which three collagen polypeptide chains, called α chains, are wound around one another in a ropelike superhelix
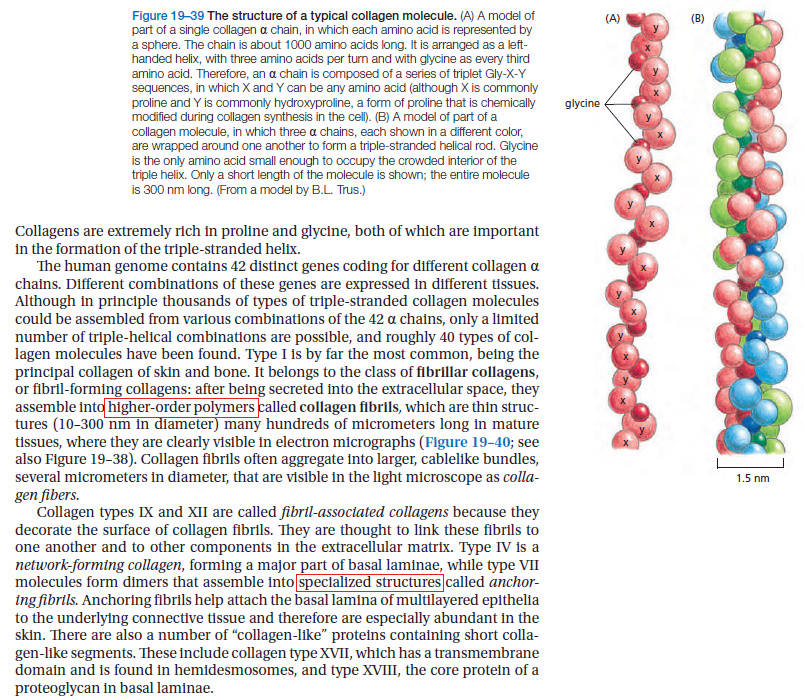
Fibroblasts may have a similar role in organizing the extracellular matrix inside the body. First they synthesize the collagen fibrils and deposit them in the correct orientation. Then they work on the matrix they have secreted, crawling over it and tugging on it so as to create tendons and ligaments and the tough, dense layers of connective tissue that surround and bind together most organs.
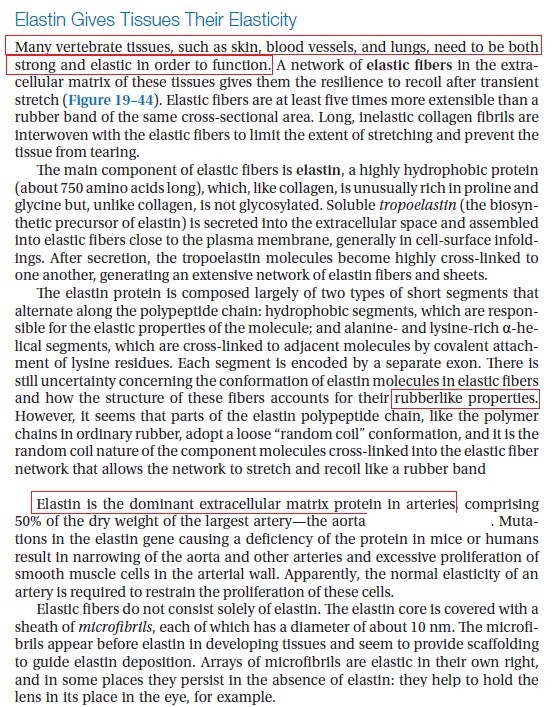
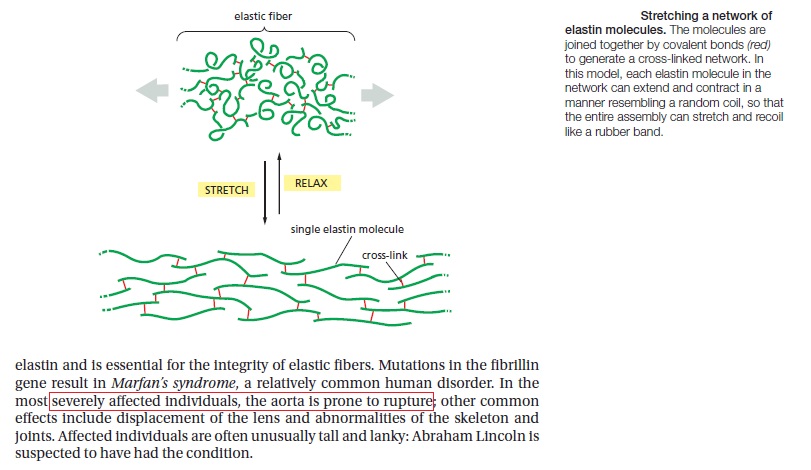
Evolution of Collagen
Fibril-forming (fibrillar) collagens are extracellular matrix proteins conserved in all multicellular animals. 10 Vertebrate members of the fibrillar collagen family are essential for the formation of bone and teeth, tissues that characterise vertebrates. The potential role played by fibrillar collagens in vertebrate evolution has not been considered previously largely because the family has been around since the sponge and it was unclear precisely how and when those particular members now found in vertebrates first arose.
Collagen cross-linking: insights on the evolution of metazoan extracellular matrix 7
Collagens constitute a large family of extracellular matrix (ECM) proteins that play a fundamental role in supporting the structure of various tissues in multicellular animals. The mechanical strength of fibrillar collagens is highly dependent on the formation of covalent cross-links between individual fibrils, a process initiated by the enzymatic action of members of the lysyl oxidase (LOX) family. Fibrillar collagens are present in a wide variety of animals, therefore often being associated with metazoan evolution, where the emergence of an ancestral collagen chain has been proposed to lead to the formation of different clades. While LOX-generated collagen cross-linking metabolites have been detected in different metazoan families, there is limited information about when and how collagen acquired this particular modification. By analyzing telopeptide and helical sequences, we identified highly conserved, potential cross-linking sites throughout the metazoan tree of life. Based on this analysis, we propose that they have importantly contributed to the formation and further expansion of fibrillar collagens.
How are collagens synthesized?
Collagen biosynthesis is complex, involving numerous intracellular and extracellular steps, all of which contribute to the structure and biomechanical properties of the final fibrils. The first event following synthesis of procollagen chains on the ribosome is their import into the rough endoplasmic reticulum. There they undergo a series of post-translational modifications resulting in the assembly of procollagen molecules (Fig. 2.4)

These steps include
modification of proline residues to hydroxyprolines,
modification of lysines to hydroxylysines,
N- and O-linked glycosylation,
trimerization,
disulfide bonding,
prolyl cis–trans isomerization and folding of the triple helix.
Molecules then transit the Golgi network where they are packaged into secretory vesicles prior to export into the extracellular matrix.
Procollagen processing occurs during or shortly after secretion followed by assembly of fibrils. Finally, fibrils are stabilized by the formation of covalent cross-links.
Post-translationalModifications of Polypeptide Chains
It has long been known that stability of the collagen triple helix is related to the total content of the amino acids proline and hydroxyproline, which together make up about 20% of the total amino acids in human fibrillar collagens. This is a result of the cyclic nature of the side chains that restrict flexibility about the peptide bond. Prolyl hydroxylation provides further stabilization, as shown by the approximately 30◦C drop in melting temperature when this process is prevented. Hydroxylation occurs mainly on prolines in the Y position of the Gly-X-Y triplet, through the action of prolyl 4-hydroxylases (P4H), and also to a much lesser extent on prolines in the X-position, through the action of prolyl 3-hydroxylases (P3H).
Prolyl 4-hydroxylase The collagen prolyl 4-hydroxylases (P4Hs), enzymes residing within the endoplasmic reticulum, have a central role in the biosynthesis of collagens. 11 The best known role for Hyp is in stabilizing the collagen triple helix
Prolyl 3-hydroxylation is a rare but conserved post-translational modification in many collagen types and, when defective, may be linked to a number of human diseases with musculoskeletal and potentially ocular and renal pathologies. Prolyl 3-hydroxylase-1 (P3H1), the enzyme responsible for converting proline to 3-hydroxyproline (3Hyp) in type I collagen, requires the coenzyme CRTAP for activity. 11
The enzymes responsible are 2-oxoglutarate and non-heme-Fe(II)-dependent dioxygenases, which require Fe2+, 2-oxoglutarate, O2 and ascorbate for activity. In humans, three forms of P4H and three forms of P3H have been described. P4H is a tetramer consisting of two subunits and two subunits. The subunit is common to all forms of the enzyme and also exists as an isolated protein with both enzyme and chaperone activity, protein disulfide isomerase (PDI). Different forms of P4H therefore differ in the subunit, which shows tissue specificity, the type II enzyme being the major form in chondrocytes, osteoblasts and endothelial cells. While prolyl hydroxylation in the Y position is the norm, hydroxylation of prolines in the X-position is much less common, there being only one such residue per chain in collagens I and II, in contrast to collagen IV which has several. Nevertheless, this modification performs a vital function, as recently shown by loss of prolyl 3-hydroxylase activity which leads to a recessive metabolic bone disorder resembling lethal/severe osteogenesis imperfecta. It should be noted that for steric reasons, prolyl hydroxylation occurs only prior to assembly of the triple-helical procollagen molecule.
Following transcription, collagen synthesis starts with the translation of the alpha chain mRNAs on the rough ER. As the growing a chains are translocated into the lumen of the ER, the collagenous domains are post-translationally modified by hydroxylation of peptidyl prolyl and lysyl residues to form hydroxyproline and hydroxylysine respectively. In addition, some hydroxylysine residues are glycosylated with galactose and glucose residues. Once the full-length a chain has been translocated into the ER lumen, the carboxyl terminal noncollagenous (NC1) domain folds. Correctly folded NC1 domains select appropriate partners and trimerize bringing the three a chain collagenous domains together in register. The triple helix nucleates at its carboxyl terminal end allowing the complete collagenous triple helical domain to subsequently fold or ‘zip up’ in the C-to-N direction (Fig. 1). Upon secretion, collagen molecules assemble to form macromolecular aggregates. For fibril-forming or ‘fibrillar’ collagens, which are the focus of this article, the mechanisms controlling macromolecular assembly are relatively well understood (in vertebrate systems—Fig. 1). A full-length fibrillar collagen molecule, or ‘procollagen’, consists of the C-terminal NC1 domain (C-propeptide), a collagenous domain with approximately 337–340 Gly-X-Y repeats (major helix), and an Npropeptide consisting of a short triple helical domain (minor helix) and a non-collagenous NC2 domain. The N- and C propeptides are highly soluble and keep the insoluble collagenous domain in solution. As the fibrillar procollagen is secreted from the cell, specific enzymes (C- and N-proteinases) cleave the propeptides from the collagenous domains. The dramatic drop in solubility causes the collagenous domains to laterally aggregate with a 1 4 = 1 3 staggers to the overlap regions. Lysyl oxidase catalyzes covalent cross linking between adjacent collagen molecules stabilizing the fibril (Fig. 1). More detailed accounts of collagen synthesis and assembly are given in other recent reviews.
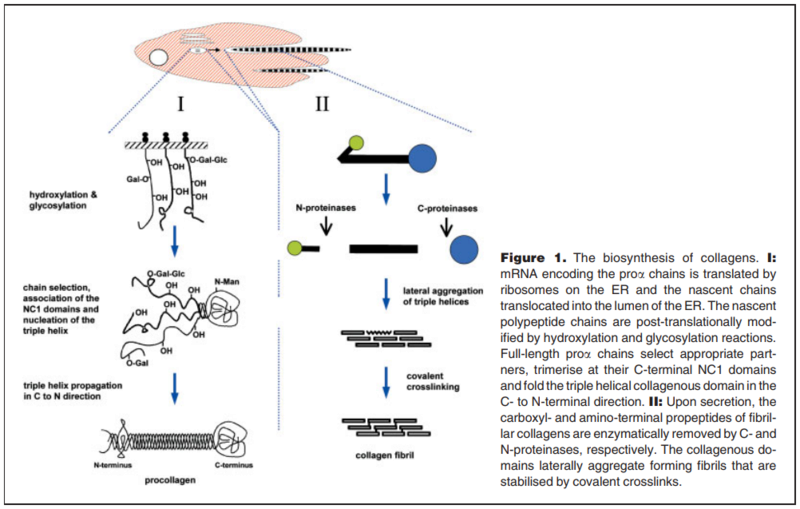
Collagens constitute a large family of extracellular matrix (ECM) proteins that play a fundamental role in providing the structural integrity and biomechanical properties of different tissues. In vertebrates, 28 types of collagens have been described (I-XXVIII), which are divided into several families, the most important being fibrillar collagens (I-III, V, XI, XXIV, and XXVII) and basement membrane-forming collagen IV2.
The biosynthesis of collagen is a highly complicated process involving numerous steps, including chain association and folding, secretion, procollagen processing and cross-linking
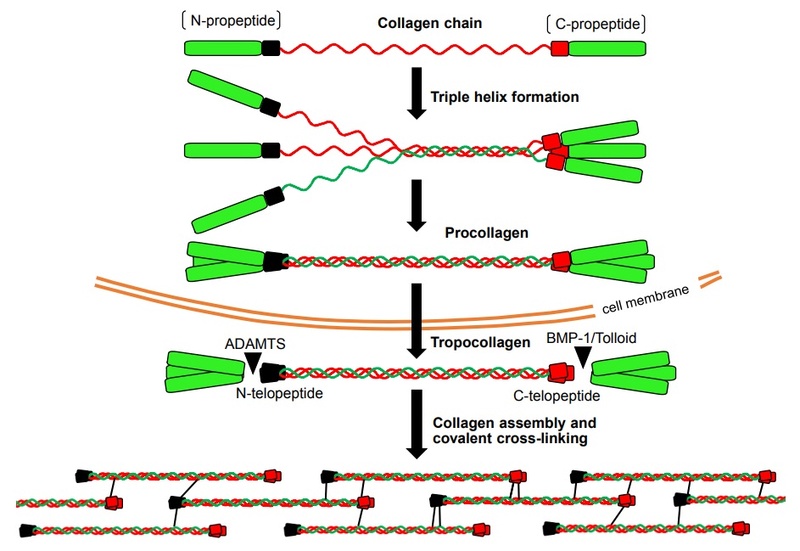
As exemplified for human type I collagen, a heterotrimeric molecule composed of two α1 and one α2 chain, after synthesis on the ribosome and their import into the rough endoplasmic reticulum, collagen chains are subjected to a series of post-translational modifications resulting in the assembly of procollagen chains. These include hydroxylation of specific proline residues, catalyzed by prolyl-4- and prolyl-3-hydroxylases (P4H and P3H enzymes); hydroxylation of specific lysine residues by lysyl hydroxylases (encoded in human by PLOD1-3 genes); N- and O-linked glycosylation, disulfide bonding and prolyl cis-trans isomerization. Association of the three α-chains occurs through a process governed by the C-terminus, and the formation of the triple helix is propagated towards the N-terminal end in a zipper-like fashion to form the procollagen molecule. This precursor molecule is then transported to the Golgi network where it is packaged into specialized secretory vesicles prior to export into the extracellular medium. Formation of fibrils from procollagen chains requires their proteolytic processing. The N- and C-propeptides are cleaved off by metalloproteinases belonging to the ADAMTS (a disintegrin and metalloproteinase with thrombospondin motifs) and BMP1 (bone morphogenetic protein 1)/Tolloid-like families, respectively, yielding the tropocollagen molecule, which retains a short portion of the propeptides termed telopeptides. Then, lysine or hydroxylysine residues within these non-collagenous domains are oxidatively deaminated by LOX, yielding the corresponding aldehydes which constitute the initiation products for the cross-linking formation. Within hours of helix formation, these telopeptide aldehydes spontaneously react with helical lysines or hydroxylysines to form immature cross-links, which further react among them and with remaining lysine or hydroxylysine residues over months/years to form permanent cross-links. The formation of these permanent or mature cross-links is fundamental as they determine the topology of adjacent molecules and contribute to the stiffness of the collagen fibril, where variations in the usage of lysine or hydroxylysine in both telopeptide and helix sites modulate the mechanical properties of the collagen matrix. In fact, defects in PLOD2, the lysyl hydroxylase isoform that specifically acts on lysine residues in collagen telopeptides, are responsible for Brück syndrome, a heritable disorder in the osteogenesis imperfecta spectrum, characterized by severe reduction or elimination of the telopeptide hydroxylysine-derived cross-links, resulting in bone fragility. In addition to lysyl hydroxylases, collagen-associated proteins such as the small leucine-rich protein (SLRP), fibromodulin, have been shown to influence collagen cross-linking, as recently evidenced in fibromodulin-deficient mice.
As cDNA and genomic sequences became available, it became increasingly evident that many ECM genes such as collagens and laminins are very ancient, and, in the last decade, as genomic sequences were determined for many metazoa, it was recognized that many ECM-encoding genes originated early in metazoan evolution. 9
The collagen architecture is the major determinant of the function and mechanical behavior of cardiovascular tissues. 8 Different collagen architectures, including collagen organization, cross-linking and collagen content, result in variation in biomechanical behavior between and within tissues.
1. https://en.wikipedia.org/wiki/Collagen
2. Molecular biology of the cell, 6th edition, page 1061
3. http://creationsafaris.com/crev200410.htm
4. https://www.eurekalert.org/pub_releases/2004-09/wuso-mmi093004.php
5. Fazale Rana, Cell's design , page 117
6. http://www.sciencedirect.com/science/article/pii/S0167488909002407
7. https://www.nature.com/articles/srep37374
8. http://journals.plos.org/plosone/article?id=10.1371/journal.pone.0127847
9. http://jcb.rupress.org/content/196/6/671
10. http://www.marisolcollazos.es/Sociologia-complemento/Articulos/Molecular_Incesto.pdf
11. https://www.ncbi.nlm.nih.gov/pmc/articles/PMC3995746/
12. http://www.sciencedirect.com/science/article/pii/S0945053X03000064