https://reasonandscience.catsboard.com/t1546-the-make-of-chlorophyll-and-what-it-tells-us-about-intelligent-design
Is photosynthesis irreducibly complex ?
https://www.youtube.com/watch?v=ktXnLnUA8XA
Tetrapyrroles such as chlorophyll and heme are indispensable for life because they are involved in energy fixation and consumption, i.e. photosynthesis and oxidative phosphorylation. 14 Tetrapyrroles are cyclic porphyrins coordinated by iron (heme) or magnesium (chlorophyll). They are essential for life, since heme is a substantial component of the respiratory chain and chlorophyll is an indispensable compound in the conversion of light energy to chemical bonds in carbohydrates through photosynthesis. Light is of fundamental importance for life on earth. Through photosynthesis, light ultimately provides the sole source of energy for the growth and development of almost all living organisms. 13 The ability to harvest and to convert the sunlight into chemical energy to be conserved in ATP and NADPH2 is one of the most striking characteristics of plant organisms. In higher plants, chlorophylls (chlorophyll a plus chlorophyll b) are involved in these processes. Chlorophyll is an extraordinary molecule. In 1818, the term chlorophyll (Chl), the green (Greek chloros) of leaf (Greek phyllon), was introduced for the pigments extracted from leaves. Experiments carried out between 1905 and 1913 led to the discovery of its structural formula. Chlorophyll is the world’s most abundant organic material. Without chlorophyll, no light is absorbed, and photosynthesis cannot occur, and therefore energy in the form of Glucose cannot be stored. They absorb photons, which are transmitted to the reaction center in photosystem II. Chlorophyll pigment molecules are essential in photosynthesis, allowing plants and other organisms to absorb solar energy from light. No chlorophyll, no caption of photons, no photosynthesis, no plant and no advanced life. In photosynthesis, 26 protein complexes are required to transform sunlight into chemical energy. that can later be released to fuel the organisms' activities (energy transformation). Each of these 26 protein complexes is essential. None can be missing. All proteins of photosynthesis must be fully developed and interlinked, they are interdependent.
Chlorophylls must be made by a complex manufacturing process. It requires a complex pathway of 17 highly specific steps, like in a production line, and at each step, specific molecular machines advance in the make of chlorophyll , one step after the other, one enzyme handing over the subproduct to the next enzyme, by which in the eighth last steps, specific enzymes are used, uniquely in this pathway. That is as to say, specific robot-like machines are in place, to do their job only and exclusively for that specific manufacturing step and process. The pathway must go all the way through, otherwise, chlorophyll is not produced. Any intermediate product has no biological function. Chlorophyll by its own has no function. It would be meaningless to produce chlorophyll exempt employed in the photosynthesis pathway.
The enzymes used in each single step use by themselves highly coordinated and complex multistep manufacturing procedures to produce the intermediate products. For example, Porphobilinogen deaminase enzyme (PBGD) is highly complex and specified in its structure, using co-factors for catalysis, and using 4 highly coordinated, ordered, sequenced and complex steps, forming a geometrically correct tetrapyrrole ( a part of chlorophyll ), and repeats the first two manufacturing steps in total 4 times.
Photovoltaic Power systems as illustration and comparison to Photosynthesis
Biological cell factories and molecular machines find often their equivalents in human-made artifacts. Photosynthesis finds its equivalent in human-made Solar Electric Power Systems which make electricity from sunlight. It would be meaningless to produce Solar panels without the other parts required in the process to produce electrical energy. Solar panels by their own are useless. In Solar Electric Power Systems, a minimal number of five interdependent and interconnected parts is required, or the process is not functional. In a similar manner, the making of solar panels requires complex factories and machines, and at least seven processing steps, of which each requires complex machines which produce the intermediate products. The process must go all through, or functional Solar panels are not produced. In the same manner, as Solar panels require complex manufacturing processes to be made, and to become functional, all other parts of the Solar Power Systems, to be made, require equally complex manufacturing processes.
Human-made solar panels exercise the same function as chlorophyll. A solar power system is designed to supply usable solar power, using solar panels in the first step to capturing solar energy. We can draw interesting parallels between human-made solar panels and power systems, to its equivalent in nature, chlorophylls, and photosynthesis, and point out, why they provide an excellent illustration of intelligent design.
In order to gain electricity through solar power, the installation of a solar power system is required. It consists of an arrangement of several components, including solar panels, a solar inverter, cabling, solar tracking system, and an integrated battery solution to store the energy produced. If any of the mentioned components are missing, solar energy cannot be gained and stored. The final goal cannot be achieved. Each of the components is essential, and need to be interconnected correctly. One of the indispensable components are solar panels.
The make of solar panels requires complex factories and machines
The basic component of solar panels, pure silicon, is not pure in its natural state. It must be brought to the manufacturing site, the solar panel factory, cleaned, and prepared for further steps. The same happens in cells, where raw material must be imported in complex mechanisms, through gates in the cell membrane. The manufacturing process goes from Purification of silicon, Making single crystal silicon, silicon wafers, doping, placing electrical contacts, anti-reflective coating, and finally encapsulating the cell. Finally, the panel goes through a quality control process and implantation. That's an eight-step production process, which must go all the way through; each step is essential and requires also several complex machines, which are specifically designed for specific processing and manufacturing goals.
It's evident that the whole process requires the invention and implementation of intelligence. That is, engineers, which first make scientific research, and based on the findings and inventions, experience and collected data, elaborate the relevant blueprints of the whole production process of every single machine, interconnections, and conceptualization as how the functional whole will provide the desired outcome. Functional parts are only meaningful within a whole, in other words, it is the whole that gives meaning to its parts. The information is based on a language system which must be pre-established. To define a specific subpart of a machine that requires a specific shape, size, material etc. the initial requirement is the language or code system, and the information based on that language to specify the part in question. In that way, the workers in the factory know how to decipher and understand the blueprint, its meaning, to produce the panels. Intelligent agents think with an "end goal" in mind, allowing them to solve complex problems by taking many parts and arranging them in intricate patterns that perform a specific function. They need to be able to organize parts availability, synchronization, manufacturing and assembly coordination and interface compatibility of the single parts and subunits. The individual parts must precisely fit together and be correctly interconnected. The production process usually takes place in complex factories which also must be fully built, and ready for production. The raw materials are sourced, transported to the factory, selected and prepared for use at the right facility. Without solar panels, no energy could be captured, a solar power system would be non-functional.
What humans invented a few decades ago, was implemented much before through photosynthesis.
Chlorophyll, by evolution?
The evolutionary model is day by day…step by step…we are getting better and better slowly with time, adding to get more complexity. But that suggested manufacturing process raises serious questions in face of the challenge to produce Chlorophyll molecules and photosynthesis.
Evolution has no foresight. So let's suppose, hundreds of millions of years would produce chlorophylls. SO WHAT ???! - on their own, there is no function for them, and if they don't have a function, natural selection would not select them. Chlorophyll by its own, without the other proteins used in the photosynthesis pathway, would have no use.
There relies on the BIG flaw in Darwin's idea: ‘Why would evolution produce a series of enzymes that only generate useless intermediates until all of the enzymes needed for the end product exist, fully functional, and interlinked in a useful manner ?’ A minimal amount of precise instructional information is required for a gene regulatory network, and genes to instruct for the orderly setup of the whole system. If a gene and epigenetic information do not contain sufficient instructions for an end function, there would be no selective advantage, and no end goal would be achieved only useless intermediary products. Thus, before a region of DNA and epigenetic codes and signalling network contain the required information to produce functional multi-part protein complexes, natural selection plays no role in guiding its making. The step by step selective mechanism is not a sufficient nor adequate and too unspecific to explain for the complex molecular proceedings observed in living cells and organisms. Intelligent pre-programming is the most adequate explanation for the origin of Chlorophyll, and photosynthesis.
What good would it be to make complex manufacturing machines to produce intermediate products for solar panel production, without all the other machines and a functional factory fully setup and in place?
What good would there be for natural selection to select and produce enzymes, used in this complex manufacturing process, without all the other enzymes in place, and the whole process coordinated to get a useful end product?
What good would it be, if solar panels would be produced, but all other parts would be missing to transform solar energy into useful energy?
What good would there be, if the chlorophyll pathway would go all the way through the 17th step? Chlorophyll would be produced, BUT:
What good for survival would there be for chlorophyll on its own, if not fully embedded in the photosynthesis process? none.
What good would there be for photosynthesis without chlorophyll in place, capturing light, and transmitting it to the photosystem? none, since capturing light is essential for the whole process.
The thing is, there's no driver for any of the pieces to emerge individually because single parts confer no advantage in and of themselves. The necessity for the parts of the system to be in place all at once is simply evidence of a planning organizing creative intelligence.
Natural selection would not select for components of a complex system that would be useful only in the completion of the much larger system.
If chlorophyll evolved before antenna proteins, whose function is to bind chlorophyll, then chlorophyll would be toxic to cells. Yet the binding function explains the selective value of antenana proteins. Why would such proteins evolve prior to chlorophyll and if they did not how would cells survive chlorophyll until they did? 11
Biological systems are functionally organized, integrated into an interdependent network, and complex, like human-made machines and factories. The wiring of an electrical device equals to the biosynthesis pathway of chlorophyll. For the assembly of a biological system of multiple parts, not only the origin of the genome information to produce all proteins/enzymes with their respective subunits and assembly cofactors must be explained, but also parts availability ( The right materials must be transported to the building site. Often these materials in their raw form are unusable. Other complex machines come into play to transform the raw materials into a usable form. All this requires specific information. ) synchronization, ( these parts must be read on hand at the building site ) manufacturing and assembly coordination ( which required the information of how to assemble each single part correctly, at the right place, at the right moment, and in the right position ) , and interface compatibility ( the parts must fit together correctly, like lock and key ) . Unless the origin of all these steps is properly explained, functional complexity as existing in biological systems has not been addressed adequately.
How could the whole process have started " off the hooks " from zero without a planning intelligence?
Why would natural, unguided mechanisms produce a series of enzymes that only generate useless intermediates until all of the enzymes needed for the end product exist, are in place and do their job?
Furthermore, the process is highly toxic for cell membranes and would destroy the surrounding molecules, if not duly protected right from the beginning.
Conclusion
The invention of Chlorophyll biosynthesis can only be explained by the acting agency of an intelligent mind. An intelligent designer is a capable agent, able of planning, with the foresight of the end result, and the requirement of machines and pathways and manufacturing processes for an end goal and useful product. Mindless, unguided, random, evolutionary processes are NOT. We are granted to infer, based on positive scientific evidence and knowledge ( no ignorance ! ) that intelligent design is the best explanation for the origin of the biosynthesis pathway of chlorophyll and photosynthesis.
More readings: Photosynthesis
https://reasonandscience.catsboard.com/t2653-maintopics-on-photosynthesis
Evolution of Chlorophyll Biosynthesis—The Challenge to Survive Photooxidation
https://www.cell.com/fulltext/S0092-8674(00)80144-0
=========================================================================================================================================
Uroporphyrinogen decarboxylase
Without enzymes, biological reaction essential to life takes 2.3 billion years: UNC study
In 1995, Wolfenden reported that without a particular enzyme, a biological transformation he deemed “absolutely essential” in creating the building blocks of DNA and RNA would take 78 million years.
“Now we’ve found a reaction that – again, in the absence of an enzyme – is almost 30 times slower than that,” Wolfenden said. “Its half-life – the time it takes for half the substance to be consumed – is 2.3 billion years, about half the age of the Earth. Enzymes can make that reaction happen in milliseconds.”
“This enzyme is essential for both plant and animal life on the planet,” Wolfenden said. “What we’re defining here is what evolution had to overcome, that the enzyme is surmounting a tremendous obstacle, a reaction half-life of 2.3 billion years.”
It takes a reaction which occurs only once every 500 million years or so in the absence of an enzyme. and brings the rate up to ~10 reactions per second This makes the speed up factor approximately 1.5 x 10^17
[ltr]17[/ltr]
“Without catalysts, there would be no life at all, from microbes to humans,” he said. “It makes you wonder how natural selection operated in such a way as to produce a protein that got off the ground as a primitive catalyst for such an extraordinarily slow reaction.” 11
============================================================================================================================================

Without blood, there would be no advanced life. Nor so without chlorophylls. What do both have in common?
The amazing similarity between blood and chlorophyll
Scientist already discovered that life comes from the sun. Chlorophyll contains oxygen, carbon, nitrogen, hydrogen and magnesium, whilst hemoglobin from the blood contains iron at the place of magnesium, see figure below. Both iron and magnesium are metallic atoms.
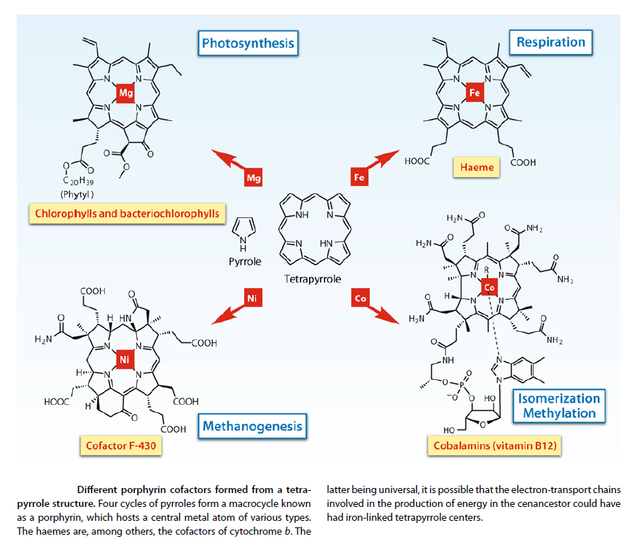
Organisms spend a lot of time acquiring energy (capacity to do work) and nutrients ( a to convey substance that an organism needs for growth and survival, but cannot make of itself). Despite the close similarity between blood and chlorophyll, plants are producers (they make their own food using energy and materials from their environment) and animals are consumers (cannot make their own food). As stressed by Jeremy Rifkin: “the first economy of the world is photosynthesis”.
Hemacyanin is another molecule which is reform conveying oxygen in hemolymph, the blood of arthropods. An arthropod are members of the phylum Arthropoda (invertebrate animal with an exoskeleton, segmented body, and jointed appendages). Insects, arachnids, crustaceans are among this group. Hemacyanin has copper at his center. It is the metallic element at the center of these molecules that give their characteristic color.
Chlorophyll, hemoglobin, and hemocyanin are three fundamental molecules of life as we know in our planet. These molecules are needed since diffusion or plasma solubility in delivering oxygen is very ineffective, and higher organisms needed to evolve in building specific proteins to transport dioxygen in the blood. These substances are able to transport oxygen in the blood through acceptance of dioxygen from a surface in contact with air (lung) or water (gill), circulate to the tissues, delivering their needed oxygen. Of course, the carrier molecule must not be irreversibly oxidized by dioxygen, and transition metals, characterized by lower oxidation states that were used to accomplish this task. Otherwise, they would be lost for further purpose. However, these metallic elements are carried, involved in a structural protein casing that protects them against irreversible oxidation (in a way not entirely known).
Oxygen may be seen as the elixir of life…and death. The name “oxygen” was bequeathed by Antoine Lavoisier (1743-1794), the revolutionary scientist and political conservative beheaded in May 1794 during the French Revolution. The great mathematician Joseph-Louis Lagrange (1736-1813) regretted the decision of the Revolutionary Tribunal and said: “it took but a moment to cut off that head, though a hundred years perhaps will be required to produce another like it”.
Life on Earth starts with an anaerobic metabolism that still nowadays persist in the form of bacteria living in oxygen-poor environments. In the early earth, nearly all oxygen was bound in compounds, like water and silicate rocks. But nearly 3 billion years ago the “invention” in nature of plant photosynthesis turned the anaerobic world into our present type of environment with aerobic life. It is clear that the introduction of oxygen into the anaerobic world obliged the organisms existing at that time to adapt since a lot of the by-products of oxygen metabolism are toxic compounds. Oxygen was essential to retain on Earth much of the hydrogen, since our planet has a relatively small mass, and can easily lose free hydrogen to the cosmic space. Without binding to oxygen, nearly all hydrogen would be lost forever.
The atomic structure dictates what an element can do, what compounds it can form, and what properties it possess. The nucleus of the common isotope of oxygen contains eight protons and eight neutrons, and is designated by 16O. Quantum mechanics tells us that electrons are not located in the nucleus as “orbitals”, but as a “cloud”, a region of space where electrons are most likely to be.
Chlorophyll biosynthesis is a complex pathway with 17 highly specific steps, of which eight last steps are used by specific enzymes uniquely in this pathway.
The pathway must go all the way through, otherwise, chlorophyll is not synthesized.
Therefore, the Chlorophyll biosynthesis pathway is irreducibly complex.
What good would there be, if the pathway would go only up to the 15th step? none
What good would there be, if the pathway would go all the way through the 17th step? Chlorophyll would be produced, BUT:
What good for survival would there be for chlorophyll on its own, if not fully embedded in the photosynthesis process? none.
What good would there be for photosynthesis without chlorophyll in place, capturing light, and transmitting it to the photosystem? none, since capturing
light is essential for the whole process.
‘Why would evolution produce a series of enzymes that only generate useless intermediates until all of the enzymes needed for the end product have evolved?’
Five following conditions would all have to be met:
the issue about the observed origin of irreducible complexity, similarly, is not to be dismissed by saying that’s Behe’s argument. Have you had an empirically warranted answer to Menuge’s C1 – 5 criteria for exaptation ( a shift in the function of a trait during evolution. For example, a trait can evolve because it served one particular function, but subsequently it may come to serve another.). . . the usually attempted counter? If not, then the issue of irreducible complexity is very definitely still on the table. The criteria:
For a working biological system to be built, the five following conditions would all have to be met:
C1: Availability. Among the parts available for recruitment to form the system, there would need to be ones capable of performing the highly specialized tasks of individual parts, even though all of these items serve some other function or no function.
C2: Synchronization. The availability of these parts would have to be synchronized so that at some point, either individually or in combination, they are all available at the same time.
C3: Localization. The selected parts must all be made available at the same ‘construction site,’ perhaps not simultaneously but certainly at the time they are needed.
C4: Coordination. The parts must be coordinated in just the right way: even if all of the parts of a system are available at the right time, it is clear that the majority of ways of assembling them will be non-functional or irrelevant.
C5: Interface compatibility. The parts must be mutually compatible, that is, ‘well-matched’ and capable of properly ‘interacting’: even if subsystems or parts are put together in the right order, they also need to interface correctly.
( Agents Under Fire: Materialism and the Rationality of Science, pgs. 104-105 (Rowman & Littlefield, 2004). HT: ENV.)
Let's have a look about the BEST explanation that proponents of natural mechanisms can come up with:
Blankenship: Molecular mechanisms of photosynthesis pg.208:
It is not conceivable that highly complex molecules such as chlorophylls were synthesized by prebiotic chemistry, given their very specific functional groups and multiple chiral centers. Instead, they are the end product of a progressive evolutionary development, in which simple molecules are the start of the biosynthesis chain and are then progressively elaborated in later steps. In this view, each intermediate in the modern pathway was at some point the end point in the pathway. This requires that each intermediate in the modern pathway be usable in the past as an end product. In the case of chlorophyll biosynthesis, Granick proposed that simple porphyrins or porphyrin precursors were the starting points and that successive steps were added to improve the efficiency of the pigments or to extend light absorption into new spectral ranges. This is an appealing idea and is probably at least partially true. The Granick hypothesis in the context of photosynthesis has been championed by Mauzerall (1992), as well as embraced by others (Olson and Pierson, 1987; Olson, 1999, 2006).
This is about the best explanation that proponents of naturalistic origins can come up with. All it exemplifies is baseless just so assertions in a superficial manner. Pseudo science at its best. It should be clear to any honest thinker that there is a huge gap of explanatory power between proponents of naturalism, and design.
Resumed: For the assembly of a biological system of multiple parts, following steps must be explained: the origin of the genome information to produce all subunits and assembly cofactors. Parts availability, synchronization, manufacturing and assembly coordination through genetic information, and interface compatibility. The individual parts must precisely fit together. All these steps are better explained through a super intelligent and powerful designer, rather than mindless natural processes by chance, or/and evolution since we observe all the time minds capabilities producing machines and factories, producing machines and end products.
everything *has* to be in place at once or else an organism has no survival advantage. The thing is, there's no driver for any of the pieces to evolve individually because single parts confer no advantage in and of themselves. The necessity for the parts of the system to be in place all at once is simply evidence of creation. Photosynthesis missing one piece (like chlorophylls) is like a car missing just one piece of the drive train (such as a differential); it's not that it doesn't function as well - it doesn't function at all!
Outline of chlorophyll a biosynthesis from glutamate. The enzymes that catalyze the individual numbered reactions are
(1) glutamyl-tRNA synthetase;
(2) glutamyl-tRNA reductase;
(3) glutamate 1-semialdehyde aminotransferase;
(4) porphobilinogen synthase;
(5) hydroxymethylbilane synthase;
(6) uroporphyrinogen III synthase;
(7) uroporphyrinogen III decarboxylase;
(8 ) coproporphyrinogen III oxidative decarboxylase;
(9) protoporphyrinogen IX oxidase;
(10) protoporphyrin IX Mg-chelatase;
(11) S-adenosyl-L-methionine:Mg-protoporphyrin IX methyltransferase;
(12)–(14) Mg-protoporphyrin IX monomethyl ester oxidative cyclase;
(15) divinyl (proto)chlorophyllide 4-vinyl reductase;
(16) light-dependent NADPH:protochlorophyllide oxidoreductase or light-independent protochlorophyllide
reductase;
(17) chlorophyll synthase.
Outline of chlorophyll a biosynthesis from glutamate. The enzymes that catalyze the individual numbered reactions are
(1) glutamyl-tRNA synthetase;
(2) glutamyl-tRNA reductase;
(3) glutamate 1-semialdehyde aminotransferase;
(4) porphobilinogen synthase;
(5) hydroxymethylbilane synthase;
(6) uroporphyrinogen III synthase;
(7) uroporphyrinogen III decarboxylase;
(8 )coproporphyrinogen III oxidative decarboxylase;
(9) protoporphyrinogen
IX oxidase;
The assembly of chlorophyll takes fiveteen enzymes. 1
Natural selection could not operate to favor a system with anything less than all seventeen being present and functioning. What evolutionary process could possibly produce complex sophisticated enzymes that generate nothing useful until the whole process is complete? Some proponents of evolution argue that the assumed primeval organic soup had many of the simpler chemicals and that only as they were used up did it become necessary to generate the earlier enzymes in the pathway. InThe Mystery of Life’s Origin: Reassessing Current Theories, the authors set forth the good basic chemistry that demonstrates that there could never have been an organic soup, and present some of the evidence out there in the world indicating that there never was. Denton and Overman also cite a number of experts who suggest that there is no evidence for such a primitive soup but rather considerable evidence against it.
Chlorophyll itself, and many of the intermediates along its pathway of synthesis can form triplet states, which would destroy surrounding lipids by a free radical cascade apart from the context of the enzymes that manufacture them and the apoproteins into which they are inserted at the conclusion of their synthesis. According to Asada ‘triplet excited pigments are physiologically equivalent to the active oxygens’, and according to Sandmann and Scheer, chlorophyll triplets ‘are already highly toxic by themselves … .’The entire process of chlorophyll synthesis from δ-aminolevulinic acid to protoporphyrin IX is apparently tightly coupled to avoid leakage of intermediates. Almost all of the enzymes of chlorophyll biosynthesis are involved in handling phototoxic material. For many of these enzymes, if they are not there when their substrate is manufactured, the cell will be destroyed by their substrate on the loose in the wrong place at the wrong time. Apel has cited four of the enzymes of chlorophyll biosynthesis for which this has been proven to be the case. This is a significant problem for evolutionists, who need time for these enzymes to evolve successively. Each time a new enzyme evolved it would have produced a new phototoxin until the next enzyme evolved.
Triplet state chlorophyll, generated in the reaction centres when singlet (excited state) chlorophyll cannot get rid of its energy quickly enough, as may be the case when excess photon energy is coming in, lasts long enough to generate very damaging singlet oxygen (1O2), which attacks lipids, proteins, chlorophyll and DNA.Evolutionists maintain that ground-state oxygen (3O2, a triplet state biradical) was not around when photosynthesis evolved. There is, however, considerable evidence that there has never been a time in Earth’s history when there was not significant free oxygen in the atmosphere (see Dimroth and Kimberley, Thaxton, Bradley and Olsen, Overman and Pannenberg,34 Denton). The evolutionists’ own analyses suggest that the last common ancestor for the bacteria and archaea already had sophisticated enzyme systems for using O2 and for disarming its reactive by-products. Since these organisms had already evolved by 3.5 Ga, on the evolutionists’ timescale,37 this also suggests something rather ominous for the absence of oxygen theory.
In the system that presently exists, a sophisticated complex of enzymes and pigments quenches the excess energy and scavenges the dangerous oxygen species generated by excess light. CuZn superoxide dismutase (in most higher plants) converts superoxide (O2-), the primary product of photoreduction of dioxygen in PSI, to H2O2 in the highest-known diffusion-controlled rate among enzymatic reactions. It appears that about one molecule of superoxide dismutase attaches to the surface of the membrane in the vicinity of the PSI complex, along with ascorbate peroxidase (APX). Ascorbate reduces the H2O2 generated, in a reaction catalyzed by APX. The product of this reaction, the monodehydroascorbate radical, is reduced again to ascorbate by photoreduced ferredoxin (Fd) in PSI.The enzymes and other reducing species of this system could not evolve gradually and then microcompartmentalize over time because nothing works unless everything is in place. This means that the first appearance of oxygen would have been lethal to the cell, whether the source of oxygen was biological or non-biological. Enzymes such as superoxide dismutase would not have been able to evolve at all. APX, for example, has only about 31–33% homology with cytochrome c peroxidase, from which it is thought to have evolved.Cells without these enzymes exposed to ground-state oxygen would simply have been destroyed before hundreds of base pair changes generated the enzymes from something else.
Natural selection is not evolution’s friend. In answer to the question, ‘Why would evolution produce a series of enzymes that only generate useless intermediates until all of the enzymes needed for the end product have evolved?’
The question, ‘Why and how would evolution go about trying to produce a protein for binding pigment molecules before pigment molecules existed?’ is another major challenge for proponents of evolution.
If chlorophyll evolved before the antenna proteins that bind it, it would in all likelihood destroy the cell, so the proteins had to evolve first. But natural selection could not favor a ‘newly evolved’ protein which could bind chlorophyll and other pigment molecules before those crucial pigments had themselves come into existence! Each binding site must be engineered to bind chlorophyll an or chlorophyll b only or carotene only. The carotene molecules must be present in just the right places for quenching triplet states in the chlorophylls. Even if the pigment molecules were already around, producing just the right protein would be an extremely difficult task. It would not only have to bind pigment molecules only, but it would need to bind just the right pigments in just the right places in just the right orientation so that energy could be transferred perfectly between them, with a little lower energy at each step. Anything else would do nothing or would transfer energy at random, and the complex would accomplish nothing at best and burn up the cell at worst.
And there is another problem for evolution. The insertion of the pigment molecules changes the conformation of the apoprotein from about 20% to about 60% α-helical content. So evolution would have to produce a protein with a wrong shape that would assume just the right shape by the insertion of pigment molecules in just the right positions and orientations when those pigment molecules had not yet evolved.
The energy transfer timeframe between pigment molecules in the antenna complex is between 10-15 and 10-9seconds. The system that God engineered captures 95–99% of the photon energy for photochemistry, even though there are four other ways the energy can be lost during the slightly less than a billionth of a second the system has for capturing it. Humans certainly cannot begin to design systems with such efficiency, but the evolutionists are determined that chance, what Cairns-Smith calls ‘old fumble fingers’, can.
Our understanding of the assembly of apoproteins with their pigments is very poor, but we do know that the chloroplast encoded chlorophyll a binding proteins of PSI and PSII core complexes are inserted cotranslationally into the thylakoid. Protein intermediates of the D1 protein have been observed due to ribosome pausing. It may be that this ribosome pausing permits cotranslational binding of chlorophyll a to the protein. This kind of controlled insertion, with synthesis of otherwise phototoxic material, is precisely what we would expect from intelligent planning and forethought, but how might ‘old fumble fingers’ hit on such a scheme?
Chlorophyll biosynthesis is a complex pathway with 17 highly specific steps, of which eight last steps are used by specific enzymes uniquely in this pathway.
Even if we find in the sequence space the right steps to make the enzymes required to permit the synthesis of the products of these intermediate steps, so what ? the intermediate products would have no function, and no survival advantage of the organism would be provided. Natural selection could not operate to favor a system with anything less than all seventeen enzymes being present, functioning and processing all intermediate products to get the final product. What evolutionary process could possibly produce complex sophisticated enzymes that generate nothing useful until the whole process is complete? And even if everything were in place correctly, and chlorophyll were synthesized correctly, so what ? Unless chlorophyll AND all other proteins and protein complexes were fully in place, fully evolved and functional, correctly interlocked and working in an interdependent manner, photosynthesis would not happen. But even if photosynthesis would happen, so what? Why would the organism choose such an extremely complex mechanism, if it was surviving just fine previously? Furthermore, you do not just need the right enzymes. For the assembly of a biological system of multiple parts, following steps must be explained: the origin of the genome information to produce all subunits and assembly cofactors. Parts availability, synchronization, manufacturing and assembly coordination through genetic information, and interface compatibility. The individual parts must precisely fit together. All these steps are better explained through a super intelligent and powerful designer, rather than mindless natural processes by chance, or/and evolution since we observe all the time minds capabilities producing machines and factories, producing machines and end products.
everything *has* to be in place at once or else an organism has no survival advantage. The thing is, there’s no driver for any of the pieces to evolve individually because single parts confer no advantage in and of themselves. The necessity for the parts of the system to be in place all at once is simply evidence of creation. Photosynthesis missing one piece (like chlorophylls) is like a car missing just one piece of the drive train (such as a differential); it’s not that it doesn’t function as well – it doesn’t function at all!

Chlorophylls absorb photons by means of an excitation process analogous to the photoelectric effect. These pigments contain a complex ring structure, called a porphyrin ring, with alternating single and double bonds. At the center of the ring is a magnesium atom. Photons absorbed by the pigment molecule excite electrons in the ring, which are then channeled away through the alternating carbon-bond system. Several small side groups attached to the outside of the ring alter the absorption properties of the molecule in different kinds of chlorophyll . Chlorophyll absorbs in only two narrow bands, but does so with high efficiency. Therefore, plants and most other photosynthetic organisms achieve far higher overall photon capture rates with chlorophyll than with other pigments. 9
When Molecules Absorb or Emit Light,They Change Their Electronic State 10
Chlorophyll appears green to our eyes because it absorbs light mainly in the red and blue parts of the spectrum, so only some of the light enriched in green wavelengths (about 550 nm) is reflected into our eyes (see Figure 7.3).
The absorption of light is represented by Equation 7.3, in which chlorophyll (Chl) in its lowest-energy, or ground, state absorbs a photon (represented by hn) and makes a transition to a higher-energy, or excited, state (Chl*):
Chl + hn → Chl*

The distribution of electrons in the excited molecule is somewhat different from the distribution in the groundstate molecule (Figure 7.5)
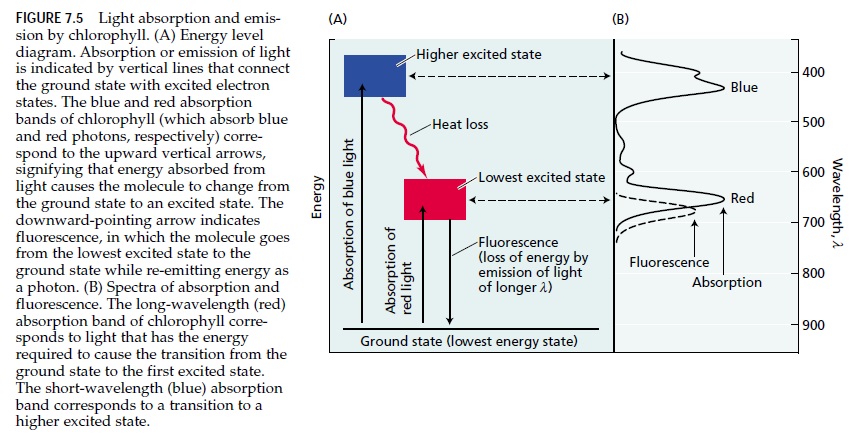
The excited molecule, such as chlorophyll (X1 ), transfers the excitation energy by resonance to an adjacent chlorophyll molecule (X2 ) and returns to its ground state.
Light in the visible range is absorbed by the electrons of the molecule, i.e., the photon energy is used to promote the electrons from their ground state to a higher energy level. 6 The photon promotes one electron to a higher energy level, leaving a defect electron (hole) in the lower level. Often, electron and hole are described as a pair of charged particles that attract each other. This bound electron–hole pair is the exciton that stores the photon energy. As the electron and the hole are located on the same molecule, the distance between the two is confined to roughly the diameter of the molecule. What is transferred is the "excited electron and the hole". We shall call this entity an "exciton". Thus, we can talk about exciton transfer. 8 We shall deal here with energy transfer between different pigments ("heterogeneous" transfer), as well as with transfer between identical molecules ("homogeneous" transfer). The latter can be repeated many times, giving rise to energy migration.
The calculated (and actually observed) "critical distances" over which the transfer probability equals 50%, are of the order of 5 nm. In a chloroplast, the distance between different pigment molecules much less than 5 nm, so that the probability of energy transfer must be quite high. The shorter the distance between pigment molecules involved in the transfer of excitation energy, the greater the probability that it will be transferred.
The crucial question is: How can a Frenkel exciton move in a pigment protein
complexes ( PPC )? To answer this question, we have to consider the electron-electron interaction between two adjacent dye molecules. Let us call one of these molecules the donor D and the other the acceptor A.
Absorption of blue light excites the chlorophyll to a higher energy state than absorption of red light because the energy of photons is higher when their wavelength is shorter. In the higher excited state, chlorophyll is extremely unstable, very rapidly gives up some of its energy to the surroundings as heat, and enters the lowest excited state, where it can be stable for a maximum of several nanoseconds (10–9 s). Because of this inherent instability of the excited state, any process that captures its energy must be extremely rapid. In the lowest excited state, the excited chlorophyll has four alternative pathways for disposing of its available energy.
1. Excited chlorophyll can re-emit a photon and thereby return to its ground state—a process known as fluorescence. When it does so, the wavelength of fluorescence is slightly longer (and of lower energy) than the wavelength of absorption because a portion of the excitation energy is converted into heat before the fluorescent photon is emitted. Chlorophylls fluoresce in the red region of the spectrum.
2. The excited chlorophyll can return to its ground state by directly converting its excitation energy into heat, with no emission of a photon.
3. Chlorophyll may participate in energy transfer, during which an excited chlorophyll transfers its energy to another molecule.
4. A fourth process is photochemistry, in which the energy of the excited state causes chemical reactions to occur. The photochemical reactions of photosynthesis are among the fastest known chemical reactions. This extreme speed is necessary for photochemistry to compete with the three other possible reactions of the excited state just described.
Photosynthetic Pigments Absorb the Light That Powers Photosynthesis
The energy of sunlight is first absorbed by the pigments of the plant. All pigments active in photosynthesis are found in the chloroplast. Structures and absorption spectra of several photosynthetic pigments are shown in Figures 7.6 and 7.7, respectively.

FIGURE 7.6 Molecular structure of some photosynthetic pigments.
(A) The chlorophylls have a porphyrin-like ring structure with a magnesium atom (Mg) coordinated in the center and a long hydrophobic hydrocarbon tail that anchors them in the photosynthetic membrane. The porphyrin-like ring is the site of the electron rearrangements that occur when the chlorophyll is excited and of the unpaired electrons when it is either oxidized or reduced. Various chlorophylls differ chiefly in the substituents around the rings and the pattern of double bonds.
(B) Carotenoids are linear polyenes that serve as both antenna pigments and photoprotective agents.
(C) Bilin pigments are open-chain tetrapyrroles found in antenna structures known as phycobilisomes that occur in cyanobacteria and red algae.

9

Absorption Spectra and Pigments
How does a molecule “capture” the energy of light? A photon can be envisioned as a very fast-moving packet of energy. When it strikes a molecule, its energy is either lost as heat or absorbed by the electrons of the molecule, boosting those electrons into higher energy levels. Whether or not the photon’s energy is absorbed depends on how much energy it carries (defined by its wavelength) and on the chemical nature of the molecule it hits. Electrons occupy discrete energy levels in their orbits around atomic nuclei. To boost an electron into a different energy level requires just the right amount of energy, just as reaching the next rung on a ladder requires you to raise your foot just the right distance. A specific atom can, therefore, absorb only certain photons of light—namely, those that correspond to the atom’s available electron energy levels. As a result, each molecule has a characteristic absorption spectrum, the range and efficiency of photons it is capable of absorbing. Molecules that are good absorbers of light in the visible range are called pigments. Organisms have a variety of different pigments, but there are only two general types used in green plant photosynthesis: carotenoids and chlorophylls. Chlorophylls absorb photons within narrow energy ranges. Two kinds of chlorophyll in plants, chlorophylls a and b, preferentially absorb violet-blue and red light (figure 10.5). Neither of these pigments absorbs photons with wavelengths between about 500 and 600 nanometers, and light of these wavelengths is, therefore, reflected by plants. When these photons are subsequently absorbed by the pigment in our eyes, we perceive them as green. Chlorophyll a is the main photosynthetic pigment and is the only pigment that can act directly to convert light energy to chemical energy. However, chlorophyll b, acting as an accessory or secondary light-absorbing pigment, complements and adds to the light absorption of chlorophyll a. Chlorophyll b has an absorption spectrum shifted toward the green wavelengths. Therefore, chlorophyll b can absorb photons chlorophyll a cannot. Chlorophyll b therefore greatly increases the proportion of the photons in sunlight that plants can harvest. An important group of accessory pigments, the carotenoids, assist in photosynthesis by capturing energy from light of wavelengths that are not efficiently absorbed by either chlorophyll.
Chlorophylls and Carotenoids
Chlorophylls absorb photons by means of an excitation process analogous to the photoelectric effect. These pigments contain a complex ring structure, called a porphyrin ring, with alternating single and double bonds. At the center of the ring is a magnesium atom. Photons absorbed by the pigment molecule excite electrons in the ring, which are then channeled away through the alternating carbon-bond system. Several small side groups attached to the outside of the ring alter the absorption properties of the molecule in different kinds of chlorophyll (figure 10.6).
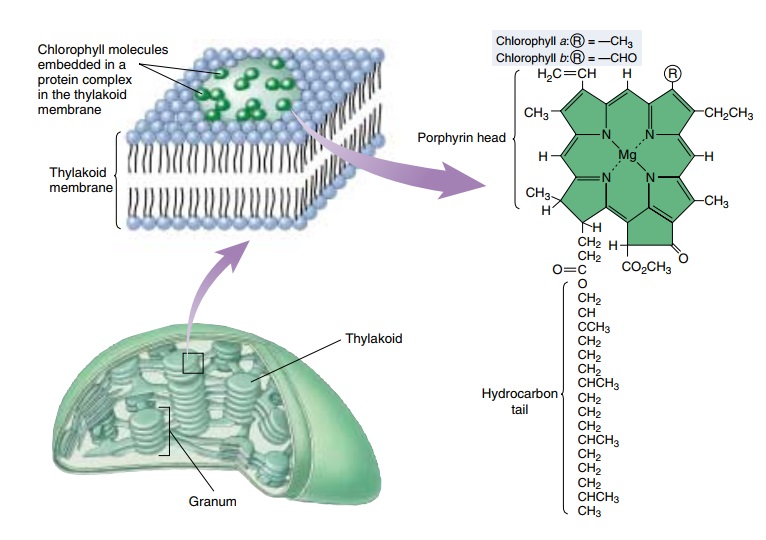
The precise absorption spectrum is also influenced by the local microenvironment created by the association of chlorophyll with specific proteins. It is reasonable to ask why these photosynthetic organisms do not use a pigment like retinal (the pigment in our eyes), which has a broad absorption spectrum that covers the range of 500 to 600 nanometers. The most likely hypothesis involves photoefficiency. Although retinal absorbs a broad range of wavelengths, it does so with relatively low efficiency. Chlorophyll, in contrast, absorbs in only two narrow bands, but does so with high efficiency. Therefore, plants and most other photosynthetic organisms achieve far higher overall photon capture rates with chlorophyll than with other pigments.
1) https://answersingenesis.org/evidence-against-evolution/shining-light-on-the-evolution-of-photosynthesis/
2) http://www.synarchive.com/syn/24
3. http://citeseerx.ist.psu.edu/viewdoc/download?doi=10.1.1.94.171&rep=rep1&type=pdf
4. https://creation.com/shining-light-on-the-evolution-of-photosynthesis
5. https://br.answers.yahoo.com/question/index?qid=20120702140557AAfDaJu
6. The Biophysics of Photosynthesis, page 8
7. http://www.life.illinois.edu/govindjee/biochem494/foerster.htm
8. Chlorophyll Fluorescence Understanding Crop Performance— Basics and Applications, page 8
9. http://www.mhhe.com/biosci/genbio/raven6b/graphics/raven06b/other/raven06b_10.pdf
10. Plant Physiology, 3rd ed by Lincoln Taiz and Eduardo Zeiger, page 11
11. https://uncommondescent.com/intelligent-design/is-photosynthesis-irreducibly-complex/#more-2255
12. https://www.med.unc.edu/biochem/news/2008/without-enzyme-biological-reaction-essential-to-life-takes-2-3-billion-years-unc-study
13. https://www.cell.com/fulltext/S0092-8674(00)80144-0
14. https://journals.plos.org/plosone/article?id=10.1371/journal.pone.0166338
Last edited by Otangelo on Sun Feb 06, 2022 4:14 pm; edited 145 times in total