12. Chromatin Dynamics
Chromatin dynamics refer to the dynamic changes that occur in the structure and organization of chromatin within a cell's nucleus. Chromatin is the complex of DNA, RNA, and proteins that make up the genetic material in a cell. It can undergo various modifications, including changes in its packaging, accessibility, and interactions with regulatory factors. These changes play a crucial role in gene expression regulation, cellular differentiation, and development.
Importance in Biological Systems
Chromatin dynamics are essential for a wide range of biological processes:
Gene Regulation: Chromatin structure can influence whether genes are turned on (active) or off (inactive). Modifications to chromatin can make specific regions of the genome more or less accessible to transcription factors, thereby affecting gene expression.
Cell Differentiation: During the development of multicellular organisms, cells differentiate into various cell types with distinct functions. Chromatin dynamics play a pivotal role in determining which genes are activated or suppressed in different cell types, leading to cellular specialization.
DNA Replication and Repair: Chromatin structure needs to be temporarily modified during DNA replication and repair processes to allow access to the DNA strands. These modifications ensure accurate DNA replication and efficient repair of damaged DNA.
Epigenetic Inheritance: Epigenetic modifications, such as DNA methylation and histone modifications, can be passed on from one generation of cells to the next. These modifications can affect gene expression patterns in progeny cells and can play a role in hereditary traits.
Developmental Processes Shaping Organismal Form and Function
Chromatin dynamics contribute significantly to the development of organisms, influencing their form and function:
Embryonic Development: During embryogenesis, the precise orchestration of gene expression is crucial for the formation of tissues, organs, and body structures. Chromatin dynamics guide the activation and repression of genes involved in this process.
Tissue Differentiation: As tissues develop, specific patterns of chromatin modifications guide the differentiation of stem cells into various cell types, determining their unique functions and properties.
Morphogenesis: The process by which organisms develop their shape involves intricate regulation of gene expression. Chromatin dynamics play a role in controlling the timing and spatial patterns of gene expression that underlie morphogenesis.
Homeostasis and Disease: Throughout an organism's life, chromatin dynamics help maintain cellular homeostasis. Dysregulation of chromatin structure and gene expression can contribute to various diseases, including cancer and developmental disorders.
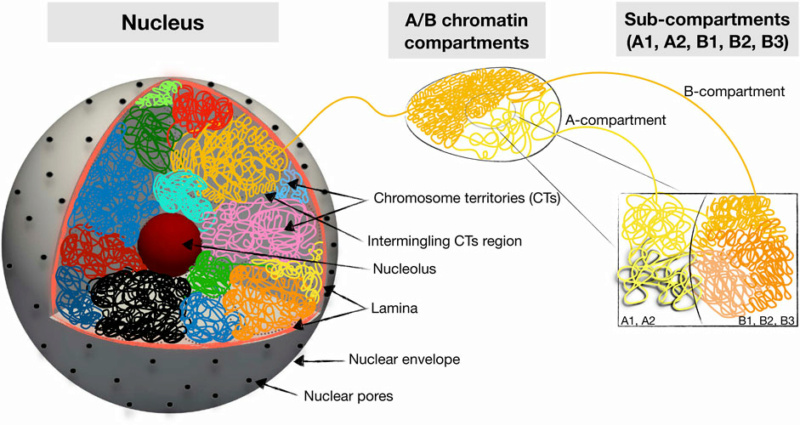
Schematic summary of hierarchical 3D folding of chromatin into compartments and domains. Shown are various keywords relevant for describing nuclear chromatin architecture along with length scales relevant for modeling and imaging studies. 1
How do chromatin dynamics influence gene expression and epigenetic regulation during development?
Chromatin dynamics play a critical role in influencing gene expression and epigenetic regulation during development. Epigenetic regulation refers to heritable changes in gene expression that do not involve alterations in the DNA sequence itself, but rather modifications to the chromatin structure and associated molecules. These modifications can be influenced by environmental factors and developmental cues. Here's how chromatin dynamics influence gene expression and epigenetic regulation during development:
Chromatin Accessibility and Gene Expression
Chromatin can exist in two main states: closed and condensed (heterochromatin) or open and accessible (euchromatin). The accessibility of chromatin directly impacts the binding of transcription factors, which are proteins that regulate gene expression. During development, specific genes need to be turned on or off at different stages to drive differentiation and tissue formation.
Chromatin dynamics, including histone modifications and DNA methylation, influence the level of chromatin accessibility. Histone modifications can lead to changes in the overall chromatin structure, making certain genomic regions more or less accessible to transcription factors and other regulatory molecules. DNA methylation can also affect gene expression by modulating chromatin accessibility.
Histone Modifications
Histones are proteins around which DNA is wrapped to form nucleosomes. Post-translational modifications (such as acetylation, methylation, phosphorylation, and more) to histones can either promote or inhibit the binding of transcription factors and other regulatory proteins. For example, histone acetylation is associated with open chromatin and active gene expression, while histone methylation can have activating or repressing effects depending on the specific histone and site of modification.
DNA Methylation
DNA methylation involves the addition of a methyl group to the DNA molecule, often occurring at cytosine residues within CpG dinucleotides. Methylation at promoter regions can block the binding of transcription factors and other regulatory proteins, leading to gene silencing. During development, DNA methylation patterns are established and can be passed on to daughter cells. Aberrant DNA methylation patterns can lead to developmental disorders and diseases.
Epigenetic Memory and Cellular Differentiation
Chromatin modifications can contribute to the establishment of epigenetic memory. As cells differentiate during development, specific chromatin modifications can be inherited by daughter cells, helping to maintain their identity and gene expression profiles. This is particularly important for ensuring that cells remain committed to their specific lineages as tissues and organs develop.
Environmental Influence
Developmental cues and environmental factors can influence chromatin dynamics and epigenetic regulation. For example, exposure to specific signals during development can trigger changes in chromatin structure that promote or inhibit gene expression in response to external stimuli.
What are the molecular mechanisms that remodel chromatin structure and accessibility?
The molecular mechanisms that remodel chromatin structure and accessibility involve a complex interplay of various enzymes, protein complexes, and regulatory factors. These mechanisms can lead to changes in chromatin packaging and modifications that affect gene expression. Here are some of the key molecular processes involved:
Histone Modifications
Histone modifications are one of the primary ways chromatin structure is remodeled. Enzymes such as histone acetyltransferases (HATs) add acetyl groups to histone tails, neutralizing their positive charge and leading to a more relaxed chromatin structure (euchromatin) that is conducive to gene expression. Conversely, histone deacetylases (HDACs) remove acetyl groups, promoting a condensed chromatin state (heterochromatin) that represses gene expression. Other histone modifications, such as methylation, phosphorylation, ubiquitination, and SUMOylation, can also influence chromatin structure and accessibility. For example, histone methylation can be associated with both gene activation and repression, depending on the specific histone residue and degree of methylation.
Chromatin Remodeling Complexes
Chromatin remodeling complexes are multi-subunit complexes that use the energy of ATP hydrolysis to alter the position of nucleosomes along the DNA. These complexes can slide nucleosomes to expose or hide specific DNA sequences, making them more or less accessible to transcription factors and other regulatory proteins. SWI/SNF, ISWI, and INO80 are examples of chromatin remodeling complexes that play roles in various chromatin-related processes.
DNA Methylation
DNA methylation involves the addition of a methyl group to cytosine residues in CpG dinucleotides. DNA methyltransferases (DNMTs) are responsible for adding methyl groups, which can lead to gene silencing by preventing the binding of transcription factors and other regulatory proteins. DNA demethylation mechanisms, such as active demethylation via ten-eleven translocation (TET) enzymes and passive demethylation during DNA replication, can also contribute to chromatin remodeling and gene expression changes.
Histone Variants
Variants of canonical histones can replace the standard histones in nucleosomes, leading to altered chromatin properties. For instance, the incorporation of histone variants like H2A.Z and H3.3 can influence chromatin accessibility and transcriptional activity.
Non-Coding RNAs
Non-coding RNAs, including microRNAs and long non-coding RNAs, can interact with chromatin to regulate its structure and accessibility. Some non-coding RNAs guide chromatin-modifying complexes to specific genomic locations, influencing gene expression.
Polycomb and Trithorax Group Proteins
Polycomb group (PcG) and trithorax group (TrxG) proteins are involved in maintaining epigenetic states of gene repression and activation, respectively. They help establish and maintain specific chromatin modifications that influence gene expression patterns during development and differentiation.
These mechanisms are interconnected and can have cascading effects on gene expression. The interplay between these factors is highly complex and tightly regulated to ensure proper control of gene expression in response to developmental cues and environmental signals.
Appearance of chromatin dynamics in the evolutionary timeline
The appearance of chromatin dynamics in the evolutionary timeline is a complex topic that involves understanding the emergence of eukaryotic cells and the development of increasingly sophisticated mechanisms for gene regulation. While the exact timeline and sequence of events are still subjects of ongoing research and debate, here is a general overview of the hypothesized appearance of chromatin dynamics in the evolutionary timeline:
Prokaryotic Stage (3.5 - 2 billion years ago): During the early stages of life on Earth, simple prokaryotic cells lacking a nucleus or complex internal structures would have been predominant. Chromatin dynamics as observed in eukaryotic cells were supposed to be absent at this stage due to the lack of organized nuclear compartments and the presence of only limited DNA-associated proteins.
Eukaryotic Evolution (2 - 1.5 billion years ago): The emergence of eukaryotic cells would have marked a significant step in the evolution of chromatin dynamics. Eukaryotes possess a distinct nucleus, allowing for more complex gene regulation. The evolution of histones and the formation of nucleosomes would have occurred during this time, enabling the packaging of DNA into chromatin and the potential for modifications to influence gene expression.
Early Chromatin Modifications (1 - 0.5 billion years ago): As eukaryotic cells diversified, the acquisition of various chromatin-modifying enzymes would have begun. Simple histone modifications like acetylation and methylation would have evolved to regulate gene expression and chromatin structure. These modifications would have played a role in early developmental processes and responses to environmental cues.
Emergence of Multicellularity (1 billion years ago - 600 million years ago): The evolution of multicellularity would have introduced a new level of complexity in gene regulation. Different cell types within multicellular organisms would have required distinct gene expression patterns. Chromatin dynamics would have become more intricate to establish and maintain cellular differentiation.
Evolution of Developmental Pathways (600 million years ago - present): With the diversification of animal phyla, more elaborate mechanisms of chromatin remodeling, including the development of chromatin remodeling complexes and epigenetic marks, would have evolved. The evolution of these mechanisms would have facilitated the emergence of complex developmental processes that shape organisms' forms and functions.
Evolution of Epigenetic Regulation (300 million years ago - present): The evolution of more advanced epigenetic mechanisms, such as DNA methylation and sophisticated histone modifications, would have allowed for greater control over gene expression patterns. These mechanisms would have played crucial roles in defining cell lineages during development, tissue specialization, and the adaptation of organisms to various environmental conditions.
Recent Evolutionary Advances (Recent history to present): In more recent evolutionary timescales, organisms would have developed highly specialized mechanisms of gene regulation and epigenetic inheritance. The evolution of non-coding RNAs, epigenetic memory, and the coordination of complex gene networks would have further contributed to the sophistication of chromatin dynamics.
It's important to note that this overview is a simplified representation of the evolutionary timeline of chromatin dynamics, and the actual events were likely more complex and intertwined.
De Novo Genetic Information necessary to instantiate chromatin dynamics
To envision the hypothetical process of generating chromatin dynamics mechanisms from scratch, we'll consider a scenario where genetic information is introduced de novo. In this scenario, several key informational components would need to originate to establish the mechanisms of chromatin dynamics during the instantiation of chromatin dynamics:
Genetic Code Encoding Regulatory Proteins: A set of genes would need to emerge that encode proteins responsible for recognizing specific DNA sequences and modifying chromatin structure. These regulatory proteins would include histone-modifying enzymes, chromatin remodeling complexes, and epigenetic writers/readers.
Histone Protein Synthesis and Modification Codes: Genetic information for histone proteins and their post-translational modification codes would need to arise. These codes would dictate how histones are modified to regulate chromatin structure and accessibility, thereby influencing gene expression.
DNA Methylation Machinery: Genes encoding enzymes responsible for DNA methylation and demethylation would need to develop. These enzymes would establish DNA methylation patterns that contribute to the epigenetic landscape and play a role in gene silencing and activation.
Non-Coding RNA Genes: The emergence of genes encoding non-coding RNAs, such as microRNAs and long non-coding RNAs, would be necessary. These RNAs could guide chromatin-modifying complexes to specific genomic loci, influencing chromatin dynamics and gene expression.
Epigenetic Memory Mechanisms: Genetic elements that facilitate the inheritance of epigenetic modifications through cell divisions would have to emerge. This could involve the creation of protein complexes that recognize and maintain specific histone modifications or DNA methylation patterns.
Chromatin Remodeling Complexes: Genes encoding chromatin remodeling complexes would need to evolve. These complexes utilize ATP to reposition nucleosomes along DNA, impacting chromatin accessibility and gene expression.
Cis-Regulatory Elements: The emergence of DNA sequences acting as enhancers, promoters, and other regulatory elements would be essential. These sequences would provide binding sites for transcription factors and other regulatory proteins, enabling precise control of gene expression.
Signal Transduction Pathways: The development of genetic pathways that translate external signals into changes in chromatin structure would be crucial. This could involve the emergence of genes encoding signal transduction components that convey environmental cues to the chromatin-modifying machinery.
Feedback Mechanisms: The establishment of genetic loops and feedback mechanisms that fine-tune chromatin dynamics would be needed. These mechanisms could sense the state of chromatin modifications and regulate the expression of chromatin-modifying factors accordingly.
Maintenance and Repair Systems: Genes coding for systems that maintain chromatin integrity and repair DNA damage caused by chromatin modifications would have to arise. These systems would ensure the stability of the epigenetic landscape over generations.
In this scenario, the generation of these genetic components and their precise integration would collectively instantiate the mechanisms of chromatin dynamics.
Manufacturing codes and languages that would have to emerge and be employed to instantiate chromatin dynamics
To transition from an organism without chromatin dynamics to one with fully developed chromatin dynamics, a complex set of manufacturing codes and languages would need to be established and instantiated. These codes would govern the creation, modification, and interpretation of various molecular components and interactions that collectively constitute the machinery of chromatin dynamics:
Histone Modification Codes: Specific chemical modifications on histone proteins (e.g., acetylation, methylation, phosphorylation) would require distinct codes that dictate where and how these modifications occur. These codes would be "read" by proteins recognizing modified histones, influencing chromatin structure and gene expression.
Epigenetic Marking Instructions: Codes would specify how and where epigenetic marks like DNA methylation are placed or removed. These instructions would guide enzymes to add or erase these marks, affecting gene regulation and chromatin accessibility.
Chromatin Remodeling Commands: Codes would direct the ATP-dependent chromatin remodeling complexes to slide or reposition nucleosomes along DNA. Different commands would dictate whether to compact or open chromatin regions, impacting gene accessibility.
Non-Coding RNA Binding Instructions: Codes would guide non-coding RNAs to their target regions on chromatin. These instructions would ensure that non-coding RNAs interact with the appropriate chromatin-modifying complexes, influencing gene expression.
Interaction Sequences for Regulatory Proteins: Regulatory proteins responsible for modifying chromatin would require interaction sequences that allow them to bind to specific chromatin regions. These sequences would ensure precise targeting of chromatin-modifying activities.
Signal Integration Algorithms: Codes would integrate external signals into chromatin dynamics. These algorithms would interpret various cues, such as environmental changes, and translate them into modifications that alter chromatin structure and gene expression.
Feedback Loop Instructions: Feedback mechanisms would involve codes that initiate when specific chromatin modifications are established. These codes would activate regulatory processes that maintain or counteract the established chromatin state.
Epigenetic Memory Signals: Codes would be needed to maintain epigenetic memory over cell divisions. These signals would ensure that daughter cells inherit and replicate the chromatin modifications present in the parent cell.
Stress Response Programs: Codes would initiate stress response pathways that modulate chromatin dynamics in response to external stressors. These codes would be activated when the organism encounters challenges that require rapid and adaptive changes in gene expression.
Cell Differentiation Signals: Instructions would specify how certain chromatin modifications guide cell differentiation processes. These signals would play a role in the formation of distinct cell types during development.
In this scenario, the establishment of these manufacturing codes and languages would involve intricate molecular interactions, biochemical pathways, and coordination among various cellular components. The precise sequencing and orchestration of these codes would lead to the development of fully functional chromatin dynamics, enabling sophisticated gene regulation and epigenetic control within the organism.
Epigenetic Regulatory Mechanisms necessary to be instantiated for chromatin dynamics
Epigenetic regulation plays a crucial role in the development of chromatin dynamics. To instantiate this regulation from scratch, several systems would need to be employed, and these systems would collaborate to maintain a balanced and functional operation:
Epigenetic Regulation Systems
Histone Modification System: A system of enzymes and proteins responsible for adding and removing various histone modifications would need to be established. Enzymes such as histone acetyltransferases (HATs), histone deacetylases (HDACs), histone methyltransferases, and demethylases would play roles in modifying histone tails and influencing chromatin structure.
DNA Methylation System: An enzymatic machinery involving DNA methyltransferases (DNMTs) would be required to add methyl groups to specific cytosine residues in DNA. DNA methylation can influence gene expression and chromatin structure.
Chromatin Remodeling System: Chromatin remodeling complexes that utilize ATP to reposition nucleosomes along DNA would need to be established. These complexes, such as SWI/SNF and ISWI, play a role in altering chromatin accessibility and structure.
Non-Coding RNA Regulation System: A system involving non-coding RNAs, such as microRNAs and long non-coding RNAs, would be necessary. These RNAs could guide chromatin-modifying complexes to specific genomic locations, modulating chromatin dynamics.
Epigenetic Memory System: Mechanisms for maintaining epigenetic memory across cell divisions would need to be set up. This could involve proteins that recognize and maintain specific epigenetic marks, ensuring their faithful inheritance.
Collaborative Systems
Signal Transduction Pathways: Signaling pathways would interpret external cues and transmit information to the epigenetic regulation systems. These pathways would be responsible for integrating developmental signals, environmental cues, and stress responses into the regulation of chromatin dynamics.
Feedback Mechanisms: Collaborative feedback loops would involve regulatory proteins that sense the state of chromatin modifications and adjust the activities of epigenetic regulators accordingly. These feedback systems would help maintain the balance of chromatin dynamics.
Cell Differentiation Networks: Networks of transcription factors and signaling pathways would collaborate with the epigenetic regulation systems to guide cell differentiation and the establishment of distinct chromatin states in different cell types.
DNA Repair and Maintenance Systems: DNA repair mechanisms would collaborate with epigenetic regulation systems to ensure the stability and fidelity of epigenetic marks. These systems would help prevent errors and maintain the integrity of chromatin dynamics.
Metabolic and Nutrient Sensing Networks: Cellular metabolic and nutrient sensing pathways could interact with epigenetic regulators to integrate metabolic status with chromatin dynamics. This collaboration would enable cells to respond to changing energy and nutrient conditions.
The development of chromatin dynamics from scratch would require the establishment of multiple epigenetic regulation systems, each with specific functions. These systems would collaborate with other cellular networks, such as signal transduction, feedback, differentiation, and maintenance systems, to ensure the precise orchestration and balanced operation of chromatin dynamics throughout various cellular processes and developmental stages.
Signaling Pathways necessary to create, and maintain chromatin dynamics
The emergence of chromatin dynamics from scratch would involve the creation and interplay of various signaling pathways that transmit information and cues within cells. These pathways would be interconnected, interdependent, and crosstalk with each other, as well as with other biological systems:
Developmental Signaling Pathways: Pathways such as Wnt, Notch, and Hedgehog would contribute to the establishment of cell identities and differentiation processes. They would communicate cues that guide the activation of specific chromatin dynamics mechanisms for different cell types.
Environmental Sensing Pathways: Signaling pathways that sense environmental factors, such as nutrient availability, oxidative stress, and temperature, would play a role. These pathways would transmit signals that modulate chromatin dynamics to adapt to changing conditions.
Stress Response Pathways: Pathways like the p38 MAPK and JNK pathways would be involved in responding to cellular stressors. These pathways could influence chromatin dynamics by altering the activity of chromatin-modifying enzymes and epigenetic regulators.
Hormone Signaling Pathways: Hormonal signals, mediated by pathways like the insulin signaling pathway, would communicate information about growth, metabolism, and development. These signals could impact chromatin dynamics and gene expression to coordinate physiological responses.
Cell Cycle Checkpoint Pathways: Signaling pathways that regulate the cell cycle, such as the p53 pathway, would interact with chromatin dynamics. They could influence the timing and coordination of chromatin modifications during cell division and growth.
DNA Damage Response Pathways: Pathways like the ATM/ATR pathway would detect and respond to DNA damage. These pathways could communicate with chromatin dynamics systems to repair damaged chromatin and prevent the propagation of mutations.
Metabolic Signaling Networks: Metabolic pathways, including mTOR and AMPK pathways, would cross-talk with chromatin dynamics. They could impact epigenetic modifications in response to changes in energy availability and nutrient status.
Inflammatory Signaling Pathways: Pathways like NF-κB and cytokine signaling pathways would connect inflammation with chromatin dynamics. Inflammatory cues could trigger changes in chromatin structure to regulate immune responses and gene expression.
Feedback Loops and Crosstalk: Signaling pathways would often form feedback loops, where chromatin modifications influence the activation or inhibition of specific signaling components. Additionally, crosstalk between pathways would allow integration of multiple signals to fine-tune chromatin dynamics responses.
Interplay with Other Biological Systems: Signaling pathways would interconnect with other biological systems such as epigenetic regulation, transcriptional machinery, and cell signaling networks. For instance, they would influence the recruitment of chromatin-modifying enzymes to specific genomic loci.
The interconnectedness and interdependence of these signaling pathways would facilitate the integration of diverse signals that guide the establishment, maintenance, and adaptation of chromatin dynamics. The crosstalk between signaling pathways and other biological systems would ensure a coordinated and context-specific response to internal and external cues, contributing to the intricate regulation of gene expression and cellular processes.
Regulatory codes necessary for maintenance and operation of chromatin dynamics
To maintain and operate chromatin dynamics, a complex set of regulatory codes and languages would need to be instantiated. These codes would govern the interactions and activities of various molecular components involved in chromatin structure and gene regulation:
Histone Modification Codes: Specific codes would dictate the addition, removal, and interpretation of histone modifications. These codes would guide the binding of reader proteins to modified histones, influencing chromatin structure and gene expression.
DNA Methylation Marks: Codes would specify the locations of DNA methylation marks, which are critical epigenetic modifications. These marks would be recognized by proteins that mediate gene silencing or activation.
Chromatin Remodeling Commands: Codes would direct the action of chromatin remodeling complexes. These commands would determine whether nucleosomes should be repositioned to open or close chromatin regions, impacting gene accessibility.
Non-Coding RNA Binding Sequences: Codes would guide non-coding RNAs to their target regions on chromatin. These sequences would enable non-coding RNAs to interact with chromatin-modifying complexes and influence gene expression.
Epigenetic Memory Signals: Codes would ensure the inheritance of epigenetic marks across cell divisions. These signals would direct the maintenance of specific chromatin modifications over time.
Feedback Loop Codes: Regulatory codes would establish feedback loops that sense the state of chromatin modifications and adjust the activities of chromatin-modifying factors accordingly. These loops would maintain the balance of chromatin dynamics.
Signal Integration Algorithms: Codes would integrate signals from various pathways into chromatin dynamics. These algorithms would interpret cues from developmental, environmental, and stress-related pathways.
Stress Response Instructions: Codes would coordinate chromatin dynamics in response to stressors. These instructions would ensure that chromatin modifications adapt to changes in cellular conditions.
Cell Differentiation Signals: Codes would define how chromatin modifications guide cell differentiation. These signals would help establish and maintain distinct chromatin states in different cell types.
Interaction Sequences for Regulatory Proteins: Regulatory proteins responsible for chromatin modifications would require specific interaction sequences. These sequences would allow these proteins to bind to chromatin and carry out their functions.
Incorporating these regulatory codes and languages would involve complex interactions, feedback loops, and coordination among various molecular players. Together, they would ensure the precise orchestration of chromatin dynamics, allowing for the dynamic regulation of gene expression and the maintenance of cellular identity and function.
How would the intricate chromatin regulatory mechanisms have to evolve to shape cell identity and developmental processes?
The intricate chromatin regulatory mechanisms play a central role in shaping cell identity and developmental processes. These mechanisms enable cells to adopt distinct fates, respond to developmental cues, and ensure proper tissue formation. Here's how these mechanisms would have had to evolve to achieve these outcomes:
Genetic Innovation and Duplication: New genes, including those encoding chromatin-modifying enzymes, transcription factors, and regulatory RNAs, could arise through gene duplication, divergence, and mutation. This genetic innovation provides the raw material for the evolution of novel chromatin regulatory functions.
Diversification of Regulatory Elements Regulatory elements, such as enhancers and promoters, could evolve or be duplicated to acquire new functions. Changes in the sequence of these elements might lead to altered binding sites for transcription factors and other regulatory proteins, enabling the fine-tuning of gene expression patterns.
Recruitment of Transcription Factors: Transcription factors, initially with general functions, could evolve to recognize specific DNA sequences associated with particular genes or cellular functions. Over time, these factors would become key players in establishing cell-specific gene expression patterns.
Coevolution of Epigenetic Marks: Epigenetic modifications and their binding proteins could coevolve to create specific interactions between histones, DNA, and regulatory proteins. This would enable the establishment of unique chromatin landscapes in different cell types, contributing to cell identity.
Epigenetic Memory Mechanisms: Epigenetic memory systems could evolve to maintain stable chromatin states during cell division. This would ensure that cells maintain their identities and gene expression profiles as they replicate and differentiate.
Integration of Signaling Pathways: Signaling pathways, which evolved to respond to environmental and developmental cues, could be integrated with chromatin regulatory networks. These pathways would instruct chromatin-modifying enzymes to modify chromatin in response to specific signals, guiding cell fate decisions.
Emergence of Long Non-Coding RNAs: Long non-coding RNAs (lncRNAs) could evolve as key regulators of chromatin dynamics. LncRNAs might interact with chromatin-modifying complexes and guide them to specific genomic regions, contributing to the establishment of distinct chromatin states.
Collaborative Gene Networks: Gene networks involving multiple regulatory elements and transcription factors could evolve to work in concert to define cell identity. These networks would enable coordinated gene expression patterns that drive cell differentiation and tissue formation.
Regulatory Robustness and Flexibility: Through evolution, chromatin regulatory mechanisms would balance robustness (maintaining cell identity) and flexibility (responding to cues). Genetic redundancy, compensatory mechanisms, and cis-regulatory element variations would contribute to this balance.
Natural Selection and Adaptation: Cells with chromatin regulatory mechanisms that confer fitness advantages—such as optimized tissue functions, better adaptation to environments, or enhanced reproductive success—would be selected over time, leading to the refinement and optimization of chromatin regulatory networks.
In summary, the evolution of intricate chromatin regulatory mechanisms involves a combination of genetic innovation, coevolution of regulatory elements and proteins, integration of signaling pathways, and the emergence of specialized regulatory RNAs. These mechanisms enable cells to establish and maintain unique chromatin states that define their identities and guide their developmental trajectories. Over time, natural selection acts on these mechanisms, leading to the diversification and specialization of cell types essential for complex organisms.
Is there scientific evidence supporting the idea that chromatin dynamics systems were brought about by the process of evolution?
The intricate choreography of chromatin dynamics presents a formidable challenge to the notion of gradual evolution. The complexity of this process, replete with its demands for the orchestration of various codes, languages, signaling systems, and proteins, leaves no room for stepwise, incremental development. The very essence of chromatin dynamics hinges on an interdependence so profound that any attempt to isolate one mechanism, language, or code system from the others renders it bereft of function. Consider, for instance, the intricate dance between histone modifications and DNA methylation. The seamless interaction between these two facets is indispensable for proper gene expression regulation. A gradualist perspective falters here, as the functional outcome only emerges when both systems are fully operational. Any intermediary stages, lacking the intricate symphony of histone modifications and DNA methylation, would not merely offer no advantage to natural selection but would pose a burden, incurring the cost of energy and resources without conferring fitness. The intricate ballet of transcription factors, promoters, enhancers, and silencers presents another enigma. For these components to exert their regulatory influence coherently, they must be instantiated simultaneously. The proposition of a stepwise evolution falls flat in the face of such a demand. Without the concerted interplay of these elements, the regulatory network remains dormant, devoid of function. It defies reason to imagine that these components, operating in isolation, could have been honed over time into a functional ensemble. A closer look at the very foundation of chromatin dynamics reveals an undeniable coalescence of intricately woven threads. The establishment of a genetic code is inextricably linked with the initiation of translation machinery. The emergence of DNA polymerase finds purpose only when in concert with the advent of DNA repair mechanisms. To envision the sequential unfolding of these components belies the very essence of their symbiotic necessity. The panorama of chromatin dynamics eludes a gradualistic genesis. The exquisite harmony between diverse elements, each with its distinct role, defies the notion of an incremental march towards functionality. The intricacy, interdependence, and instant functionality of these systems can only be attributed to an encompassing intelligence that orchestrated their simultaneous inception.
Irreducibility and Interdependence of the systems to instantiate and operate chromatin dynamics
The orchestration of chromatin dynamics is a marvel of complexity, wherein manufacturing, signaling, and regulatory codes and languages converge to shape the intricate dance of cellular function. Within this intricate tapestry, certain codes and languages are inherently irreducible, and their interdependence is palpably evident. Consider the epigenetic modifications that festoon chromatin – they are a regulatory code that profoundly influences gene expression. These modifications, such as DNA methylation and histone acetylation, are interwoven with signaling languages that transmit molecular cues, like those conveyed by methyltransferases and acetyltransferases. This interplay is critical; the manufacturing of specific epigenetic marks relies on the presence of these modifying enzymes, and their actions are precisely guided by the signaling cues. The code for epigenetic marks is meaningless without the signaling language that directs their placement. Furthermore, the regulatory codes embedded in transcription factors are intricately linked to the signaling networks they engage. Transcription factors bind to specific DNA sequences to control gene expression, but their functionality hinges on recognition motifs and cooperative interactions. These motifs, often recognized through protein-protein interactions, employ a language of shape recognition and charge complementarity. Without the precise matching of motifs and interactions, the regulatory code falls into disarray, rendering transcription factors ineffective. Crucially, these various codes and languages do not exist in isolation. They communicate, crosstalk, and collaborate to sustain functional cellular operation. The regulatory codes of transcription factors, for instance, interface with the manufacturing codes of RNA polymerase and the signaling languages of cellular responses. The cross-talk between these components is orchestrated through complex communication systems involving proteins, protein-protein interactions, and molecular gradients. The complexity of this interdependence defies a stepwise evolutionary progression. Each component's functionality emerges only in the context of the others, and any intermediary stages would lack the required synergy, rendering them non-functional and potentially detrimental to the cell's fitness. The intricate balance of signaling, manufacturing, and regulatory codes, intertwined as they are, bespeaks an intelligent design that foresaw the need for simultaneous instantiation.
The irreducible interplay of manufacturing, signaling, and regulatory codes within chromatin dynamics precludes a gradualist evolution. The intricate communication systems and interdependencies among these codes affirm the need for a holistic, fully operational foundation right from the outset, underscoring the presence of an intelligent designer.
Once chromatin dynamics are instantiated and operational, what other intra and extracellular systems is it interdependent with?
Once chromatin dynamics is instantiated and operational, it becomes intricately interdependent with a multitude of intra- and extracellular systems, each contributing to the orchestration of cellular function.
Intracellular Interdependencies
Gene Expression Machinery: The transcriptional and translational machinery collaborates closely with chromatin dynamics to ensure accurate gene expression. RNA polymerases, splicing factors, and ribosomes rely on proper chromatin structure and epigenetic marks to locate and process genes.
Epigenetic Maintenance Systems: Enzymes responsible for maintaining epigenetic marks, such as DNA methyltransferases and histone modifiers, work hand-in-hand with chromatin dynamics to perpetuate epigenetic information across cell divisions.
DNA Repair Mechanisms: DNA repair pathways are intertwined with chromatin remodeling processes. Repair enzymes need access to damaged DNA sites, and chromatin dynamics enable their interaction with the damaged regions.
Cell Cycle Regulation: Chromatin changes are pivotal during different phases of the cell cycle. Proper progression through the cell cycle depends on accurate gene expression, which is shaped by chromatin dynamics.
Cell Signaling Networks: Signaling pathways, such as those involving growth factors, hormones, and cytokines, interact with chromatin dynamics to trigger specific gene expression patterns in response to external cues.
Mitochondrial Function: The functional status of mitochondria and energy metabolism can influence chromatin structure and gene expression, showcasing the interplay between cellular energy status and chromatin dynamics.
Extracellular Interdependencies
Cell-Cell Communication: The response of a cell to its environment often involves changes in chromatin dynamics. Signaling molecules from neighboring cells can trigger epigenetic modifications and chromatin remodeling events.
Immune Responses: Immune cells can alter chromatin dynamics in response to infection or inflammation. This facilitates the activation or suppression of immune-related genes.
Developmental Pathways: During embryonic development, chromatin dynamics are tightly coordinated with signaling pathways to guide cell differentiation and tissue formation.
Stem Cell Maintenance and Differentiation: The transition of stem cells to specialized cell types relies on precise chromatin changes that dictate gene expression patterns.
Tissue Homeostasis: Proper tissue function is maintained by a balance of cell proliferation, differentiation, and apoptosis, all of which are under the influence of chromatin dynamics.
Environmental Adaptation: Chromatin changes can be responsive to environmental factors, allowing cells to adapt to changing conditions.
These intricate interdependencies further underscore the complexity and integrated nature of chromatin dynamics within the broader cellular context. The simultaneous operation of these systems and their precise coordination necessitates a comprehensive and purposeful design that goes beyond the scope of stepwise, gradual evolution.
Premise 1: Systems that rely on intricate semiotic codes and languages, and exhibit irreducible interdependencies, are unlikely to have emerged gradually through evolutionary processes due to the functional requirement of multiple components operating together.
Premise 2: Intracellular and extracellular systems, including gene expression machinery, epigenetic maintenance, DNA repair, cell cycle regulation, cell signaling networks, mitochondrial function, cell-cell communication, immune responses, developmental pathways, stem cell maintenance and differentiation, tissue homeostasis, and environmental adaptation, rely on precise semiotic codes, languages, and interdependencies.
Conclusion: Therefore, the existence and simultaneous interlocking operation of these systems strongly suggest a comprehensive and purposeful design, as their intricate, interdependent nature and requirement for coordinated operation from the outset align more with the attributes of an intelligently designed system rather than a product of stepwise, gradual evolution.
This syllogism highlights the compelling connection between the complexity of these systems, their interdependence, and the need for their simultaneous instantiation, thereby pointing towards an intelligent design as the plausible explanation for their existence and functionality.
1. Laghmach, R., Di Pierro, M., & Potoyan, D. (2021). A liquid state perspective on dynamics of chromatin compartments. Frontiers in Molecular Biology, 8, 781981. doi:10.3389/fmolb.2021.781981
Chromatin dynamics refer to the dynamic changes that occur in the structure and organization of chromatin within a cell's nucleus. Chromatin is the complex of DNA, RNA, and proteins that make up the genetic material in a cell. It can undergo various modifications, including changes in its packaging, accessibility, and interactions with regulatory factors. These changes play a crucial role in gene expression regulation, cellular differentiation, and development.
Importance in Biological Systems
Chromatin dynamics are essential for a wide range of biological processes:
Gene Regulation: Chromatin structure can influence whether genes are turned on (active) or off (inactive). Modifications to chromatin can make specific regions of the genome more or less accessible to transcription factors, thereby affecting gene expression.
Cell Differentiation: During the development of multicellular organisms, cells differentiate into various cell types with distinct functions. Chromatin dynamics play a pivotal role in determining which genes are activated or suppressed in different cell types, leading to cellular specialization.
DNA Replication and Repair: Chromatin structure needs to be temporarily modified during DNA replication and repair processes to allow access to the DNA strands. These modifications ensure accurate DNA replication and efficient repair of damaged DNA.
Epigenetic Inheritance: Epigenetic modifications, such as DNA methylation and histone modifications, can be passed on from one generation of cells to the next. These modifications can affect gene expression patterns in progeny cells and can play a role in hereditary traits.
Developmental Processes Shaping Organismal Form and Function
Chromatin dynamics contribute significantly to the development of organisms, influencing their form and function:
Embryonic Development: During embryogenesis, the precise orchestration of gene expression is crucial for the formation of tissues, organs, and body structures. Chromatin dynamics guide the activation and repression of genes involved in this process.
Tissue Differentiation: As tissues develop, specific patterns of chromatin modifications guide the differentiation of stem cells into various cell types, determining their unique functions and properties.
Morphogenesis: The process by which organisms develop their shape involves intricate regulation of gene expression. Chromatin dynamics play a role in controlling the timing and spatial patterns of gene expression that underlie morphogenesis.
Homeostasis and Disease: Throughout an organism's life, chromatin dynamics help maintain cellular homeostasis. Dysregulation of chromatin structure and gene expression can contribute to various diseases, including cancer and developmental disorders.
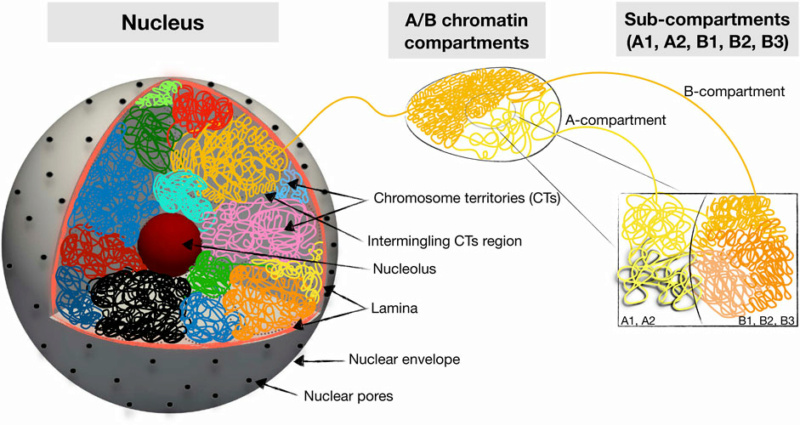
Schematic summary of hierarchical 3D folding of chromatin into compartments and domains. Shown are various keywords relevant for describing nuclear chromatin architecture along with length scales relevant for modeling and imaging studies. 1
How do chromatin dynamics influence gene expression and epigenetic regulation during development?
Chromatin dynamics play a critical role in influencing gene expression and epigenetic regulation during development. Epigenetic regulation refers to heritable changes in gene expression that do not involve alterations in the DNA sequence itself, but rather modifications to the chromatin structure and associated molecules. These modifications can be influenced by environmental factors and developmental cues. Here's how chromatin dynamics influence gene expression and epigenetic regulation during development:
Chromatin Accessibility and Gene Expression
Chromatin can exist in two main states: closed and condensed (heterochromatin) or open and accessible (euchromatin). The accessibility of chromatin directly impacts the binding of transcription factors, which are proteins that regulate gene expression. During development, specific genes need to be turned on or off at different stages to drive differentiation and tissue formation.
Chromatin dynamics, including histone modifications and DNA methylation, influence the level of chromatin accessibility. Histone modifications can lead to changes in the overall chromatin structure, making certain genomic regions more or less accessible to transcription factors and other regulatory molecules. DNA methylation can also affect gene expression by modulating chromatin accessibility.
Histone Modifications
Histones are proteins around which DNA is wrapped to form nucleosomes. Post-translational modifications (such as acetylation, methylation, phosphorylation, and more) to histones can either promote or inhibit the binding of transcription factors and other regulatory proteins. For example, histone acetylation is associated with open chromatin and active gene expression, while histone methylation can have activating or repressing effects depending on the specific histone and site of modification.
DNA Methylation
DNA methylation involves the addition of a methyl group to the DNA molecule, often occurring at cytosine residues within CpG dinucleotides. Methylation at promoter regions can block the binding of transcription factors and other regulatory proteins, leading to gene silencing. During development, DNA methylation patterns are established and can be passed on to daughter cells. Aberrant DNA methylation patterns can lead to developmental disorders and diseases.
Epigenetic Memory and Cellular Differentiation
Chromatin modifications can contribute to the establishment of epigenetic memory. As cells differentiate during development, specific chromatin modifications can be inherited by daughter cells, helping to maintain their identity and gene expression profiles. This is particularly important for ensuring that cells remain committed to their specific lineages as tissues and organs develop.
Environmental Influence
Developmental cues and environmental factors can influence chromatin dynamics and epigenetic regulation. For example, exposure to specific signals during development can trigger changes in chromatin structure that promote or inhibit gene expression in response to external stimuli.
What are the molecular mechanisms that remodel chromatin structure and accessibility?
The molecular mechanisms that remodel chromatin structure and accessibility involve a complex interplay of various enzymes, protein complexes, and regulatory factors. These mechanisms can lead to changes in chromatin packaging and modifications that affect gene expression. Here are some of the key molecular processes involved:
Histone Modifications
Histone modifications are one of the primary ways chromatin structure is remodeled. Enzymes such as histone acetyltransferases (HATs) add acetyl groups to histone tails, neutralizing their positive charge and leading to a more relaxed chromatin structure (euchromatin) that is conducive to gene expression. Conversely, histone deacetylases (HDACs) remove acetyl groups, promoting a condensed chromatin state (heterochromatin) that represses gene expression. Other histone modifications, such as methylation, phosphorylation, ubiquitination, and SUMOylation, can also influence chromatin structure and accessibility. For example, histone methylation can be associated with both gene activation and repression, depending on the specific histone residue and degree of methylation.
Chromatin Remodeling Complexes
Chromatin remodeling complexes are multi-subunit complexes that use the energy of ATP hydrolysis to alter the position of nucleosomes along the DNA. These complexes can slide nucleosomes to expose or hide specific DNA sequences, making them more or less accessible to transcription factors and other regulatory proteins. SWI/SNF, ISWI, and INO80 are examples of chromatin remodeling complexes that play roles in various chromatin-related processes.
DNA Methylation
DNA methylation involves the addition of a methyl group to cytosine residues in CpG dinucleotides. DNA methyltransferases (DNMTs) are responsible for adding methyl groups, which can lead to gene silencing by preventing the binding of transcription factors and other regulatory proteins. DNA demethylation mechanisms, such as active demethylation via ten-eleven translocation (TET) enzymes and passive demethylation during DNA replication, can also contribute to chromatin remodeling and gene expression changes.
Histone Variants
Variants of canonical histones can replace the standard histones in nucleosomes, leading to altered chromatin properties. For instance, the incorporation of histone variants like H2A.Z and H3.3 can influence chromatin accessibility and transcriptional activity.
Non-Coding RNAs
Non-coding RNAs, including microRNAs and long non-coding RNAs, can interact with chromatin to regulate its structure and accessibility. Some non-coding RNAs guide chromatin-modifying complexes to specific genomic locations, influencing gene expression.
Polycomb and Trithorax Group Proteins
Polycomb group (PcG) and trithorax group (TrxG) proteins are involved in maintaining epigenetic states of gene repression and activation, respectively. They help establish and maintain specific chromatin modifications that influence gene expression patterns during development and differentiation.
These mechanisms are interconnected and can have cascading effects on gene expression. The interplay between these factors is highly complex and tightly regulated to ensure proper control of gene expression in response to developmental cues and environmental signals.
Appearance of chromatin dynamics in the evolutionary timeline
The appearance of chromatin dynamics in the evolutionary timeline is a complex topic that involves understanding the emergence of eukaryotic cells and the development of increasingly sophisticated mechanisms for gene regulation. While the exact timeline and sequence of events are still subjects of ongoing research and debate, here is a general overview of the hypothesized appearance of chromatin dynamics in the evolutionary timeline:
Prokaryotic Stage (3.5 - 2 billion years ago): During the early stages of life on Earth, simple prokaryotic cells lacking a nucleus or complex internal structures would have been predominant. Chromatin dynamics as observed in eukaryotic cells were supposed to be absent at this stage due to the lack of organized nuclear compartments and the presence of only limited DNA-associated proteins.
Eukaryotic Evolution (2 - 1.5 billion years ago): The emergence of eukaryotic cells would have marked a significant step in the evolution of chromatin dynamics. Eukaryotes possess a distinct nucleus, allowing for more complex gene regulation. The evolution of histones and the formation of nucleosomes would have occurred during this time, enabling the packaging of DNA into chromatin and the potential for modifications to influence gene expression.
Early Chromatin Modifications (1 - 0.5 billion years ago): As eukaryotic cells diversified, the acquisition of various chromatin-modifying enzymes would have begun. Simple histone modifications like acetylation and methylation would have evolved to regulate gene expression and chromatin structure. These modifications would have played a role in early developmental processes and responses to environmental cues.
Emergence of Multicellularity (1 billion years ago - 600 million years ago): The evolution of multicellularity would have introduced a new level of complexity in gene regulation. Different cell types within multicellular organisms would have required distinct gene expression patterns. Chromatin dynamics would have become more intricate to establish and maintain cellular differentiation.
Evolution of Developmental Pathways (600 million years ago - present): With the diversification of animal phyla, more elaborate mechanisms of chromatin remodeling, including the development of chromatin remodeling complexes and epigenetic marks, would have evolved. The evolution of these mechanisms would have facilitated the emergence of complex developmental processes that shape organisms' forms and functions.
Evolution of Epigenetic Regulation (300 million years ago - present): The evolution of more advanced epigenetic mechanisms, such as DNA methylation and sophisticated histone modifications, would have allowed for greater control over gene expression patterns. These mechanisms would have played crucial roles in defining cell lineages during development, tissue specialization, and the adaptation of organisms to various environmental conditions.
Recent Evolutionary Advances (Recent history to present): In more recent evolutionary timescales, organisms would have developed highly specialized mechanisms of gene regulation and epigenetic inheritance. The evolution of non-coding RNAs, epigenetic memory, and the coordination of complex gene networks would have further contributed to the sophistication of chromatin dynamics.
It's important to note that this overview is a simplified representation of the evolutionary timeline of chromatin dynamics, and the actual events were likely more complex and intertwined.
De Novo Genetic Information necessary to instantiate chromatin dynamics
To envision the hypothetical process of generating chromatin dynamics mechanisms from scratch, we'll consider a scenario where genetic information is introduced de novo. In this scenario, several key informational components would need to originate to establish the mechanisms of chromatin dynamics during the instantiation of chromatin dynamics:
Genetic Code Encoding Regulatory Proteins: A set of genes would need to emerge that encode proteins responsible for recognizing specific DNA sequences and modifying chromatin structure. These regulatory proteins would include histone-modifying enzymes, chromatin remodeling complexes, and epigenetic writers/readers.
Histone Protein Synthesis and Modification Codes: Genetic information for histone proteins and their post-translational modification codes would need to arise. These codes would dictate how histones are modified to regulate chromatin structure and accessibility, thereby influencing gene expression.
DNA Methylation Machinery: Genes encoding enzymes responsible for DNA methylation and demethylation would need to develop. These enzymes would establish DNA methylation patterns that contribute to the epigenetic landscape and play a role in gene silencing and activation.
Non-Coding RNA Genes: The emergence of genes encoding non-coding RNAs, such as microRNAs and long non-coding RNAs, would be necessary. These RNAs could guide chromatin-modifying complexes to specific genomic loci, influencing chromatin dynamics and gene expression.
Epigenetic Memory Mechanisms: Genetic elements that facilitate the inheritance of epigenetic modifications through cell divisions would have to emerge. This could involve the creation of protein complexes that recognize and maintain specific histone modifications or DNA methylation patterns.
Chromatin Remodeling Complexes: Genes encoding chromatin remodeling complexes would need to evolve. These complexes utilize ATP to reposition nucleosomes along DNA, impacting chromatin accessibility and gene expression.
Cis-Regulatory Elements: The emergence of DNA sequences acting as enhancers, promoters, and other regulatory elements would be essential. These sequences would provide binding sites for transcription factors and other regulatory proteins, enabling precise control of gene expression.
Signal Transduction Pathways: The development of genetic pathways that translate external signals into changes in chromatin structure would be crucial. This could involve the emergence of genes encoding signal transduction components that convey environmental cues to the chromatin-modifying machinery.
Feedback Mechanisms: The establishment of genetic loops and feedback mechanisms that fine-tune chromatin dynamics would be needed. These mechanisms could sense the state of chromatin modifications and regulate the expression of chromatin-modifying factors accordingly.
Maintenance and Repair Systems: Genes coding for systems that maintain chromatin integrity and repair DNA damage caused by chromatin modifications would have to arise. These systems would ensure the stability of the epigenetic landscape over generations.
In this scenario, the generation of these genetic components and their precise integration would collectively instantiate the mechanisms of chromatin dynamics.
Manufacturing codes and languages that would have to emerge and be employed to instantiate chromatin dynamics
To transition from an organism without chromatin dynamics to one with fully developed chromatin dynamics, a complex set of manufacturing codes and languages would need to be established and instantiated. These codes would govern the creation, modification, and interpretation of various molecular components and interactions that collectively constitute the machinery of chromatin dynamics:
Histone Modification Codes: Specific chemical modifications on histone proteins (e.g., acetylation, methylation, phosphorylation) would require distinct codes that dictate where and how these modifications occur. These codes would be "read" by proteins recognizing modified histones, influencing chromatin structure and gene expression.
Epigenetic Marking Instructions: Codes would specify how and where epigenetic marks like DNA methylation are placed or removed. These instructions would guide enzymes to add or erase these marks, affecting gene regulation and chromatin accessibility.
Chromatin Remodeling Commands: Codes would direct the ATP-dependent chromatin remodeling complexes to slide or reposition nucleosomes along DNA. Different commands would dictate whether to compact or open chromatin regions, impacting gene accessibility.
Non-Coding RNA Binding Instructions: Codes would guide non-coding RNAs to their target regions on chromatin. These instructions would ensure that non-coding RNAs interact with the appropriate chromatin-modifying complexes, influencing gene expression.
Interaction Sequences for Regulatory Proteins: Regulatory proteins responsible for modifying chromatin would require interaction sequences that allow them to bind to specific chromatin regions. These sequences would ensure precise targeting of chromatin-modifying activities.
Signal Integration Algorithms: Codes would integrate external signals into chromatin dynamics. These algorithms would interpret various cues, such as environmental changes, and translate them into modifications that alter chromatin structure and gene expression.
Feedback Loop Instructions: Feedback mechanisms would involve codes that initiate when specific chromatin modifications are established. These codes would activate regulatory processes that maintain or counteract the established chromatin state.
Epigenetic Memory Signals: Codes would be needed to maintain epigenetic memory over cell divisions. These signals would ensure that daughter cells inherit and replicate the chromatin modifications present in the parent cell.
Stress Response Programs: Codes would initiate stress response pathways that modulate chromatin dynamics in response to external stressors. These codes would be activated when the organism encounters challenges that require rapid and adaptive changes in gene expression.
Cell Differentiation Signals: Instructions would specify how certain chromatin modifications guide cell differentiation processes. These signals would play a role in the formation of distinct cell types during development.
In this scenario, the establishment of these manufacturing codes and languages would involve intricate molecular interactions, biochemical pathways, and coordination among various cellular components. The precise sequencing and orchestration of these codes would lead to the development of fully functional chromatin dynamics, enabling sophisticated gene regulation and epigenetic control within the organism.
Epigenetic Regulatory Mechanisms necessary to be instantiated for chromatin dynamics
Epigenetic regulation plays a crucial role in the development of chromatin dynamics. To instantiate this regulation from scratch, several systems would need to be employed, and these systems would collaborate to maintain a balanced and functional operation:
Epigenetic Regulation Systems
Histone Modification System: A system of enzymes and proteins responsible for adding and removing various histone modifications would need to be established. Enzymes such as histone acetyltransferases (HATs), histone deacetylases (HDACs), histone methyltransferases, and demethylases would play roles in modifying histone tails and influencing chromatin structure.
DNA Methylation System: An enzymatic machinery involving DNA methyltransferases (DNMTs) would be required to add methyl groups to specific cytosine residues in DNA. DNA methylation can influence gene expression and chromatin structure.
Chromatin Remodeling System: Chromatin remodeling complexes that utilize ATP to reposition nucleosomes along DNA would need to be established. These complexes, such as SWI/SNF and ISWI, play a role in altering chromatin accessibility and structure.
Non-Coding RNA Regulation System: A system involving non-coding RNAs, such as microRNAs and long non-coding RNAs, would be necessary. These RNAs could guide chromatin-modifying complexes to specific genomic locations, modulating chromatin dynamics.
Epigenetic Memory System: Mechanisms for maintaining epigenetic memory across cell divisions would need to be set up. This could involve proteins that recognize and maintain specific epigenetic marks, ensuring their faithful inheritance.
Collaborative Systems
Signal Transduction Pathways: Signaling pathways would interpret external cues and transmit information to the epigenetic regulation systems. These pathways would be responsible for integrating developmental signals, environmental cues, and stress responses into the regulation of chromatin dynamics.
Feedback Mechanisms: Collaborative feedback loops would involve regulatory proteins that sense the state of chromatin modifications and adjust the activities of epigenetic regulators accordingly. These feedback systems would help maintain the balance of chromatin dynamics.
Cell Differentiation Networks: Networks of transcription factors and signaling pathways would collaborate with the epigenetic regulation systems to guide cell differentiation and the establishment of distinct chromatin states in different cell types.
DNA Repair and Maintenance Systems: DNA repair mechanisms would collaborate with epigenetic regulation systems to ensure the stability and fidelity of epigenetic marks. These systems would help prevent errors and maintain the integrity of chromatin dynamics.
Metabolic and Nutrient Sensing Networks: Cellular metabolic and nutrient sensing pathways could interact with epigenetic regulators to integrate metabolic status with chromatin dynamics. This collaboration would enable cells to respond to changing energy and nutrient conditions.
The development of chromatin dynamics from scratch would require the establishment of multiple epigenetic regulation systems, each with specific functions. These systems would collaborate with other cellular networks, such as signal transduction, feedback, differentiation, and maintenance systems, to ensure the precise orchestration and balanced operation of chromatin dynamics throughout various cellular processes and developmental stages.
Signaling Pathways necessary to create, and maintain chromatin dynamics
The emergence of chromatin dynamics from scratch would involve the creation and interplay of various signaling pathways that transmit information and cues within cells. These pathways would be interconnected, interdependent, and crosstalk with each other, as well as with other biological systems:
Developmental Signaling Pathways: Pathways such as Wnt, Notch, and Hedgehog would contribute to the establishment of cell identities and differentiation processes. They would communicate cues that guide the activation of specific chromatin dynamics mechanisms for different cell types.
Environmental Sensing Pathways: Signaling pathways that sense environmental factors, such as nutrient availability, oxidative stress, and temperature, would play a role. These pathways would transmit signals that modulate chromatin dynamics to adapt to changing conditions.
Stress Response Pathways: Pathways like the p38 MAPK and JNK pathways would be involved in responding to cellular stressors. These pathways could influence chromatin dynamics by altering the activity of chromatin-modifying enzymes and epigenetic regulators.
Hormone Signaling Pathways: Hormonal signals, mediated by pathways like the insulin signaling pathway, would communicate information about growth, metabolism, and development. These signals could impact chromatin dynamics and gene expression to coordinate physiological responses.
Cell Cycle Checkpoint Pathways: Signaling pathways that regulate the cell cycle, such as the p53 pathway, would interact with chromatin dynamics. They could influence the timing and coordination of chromatin modifications during cell division and growth.
DNA Damage Response Pathways: Pathways like the ATM/ATR pathway would detect and respond to DNA damage. These pathways could communicate with chromatin dynamics systems to repair damaged chromatin and prevent the propagation of mutations.
Metabolic Signaling Networks: Metabolic pathways, including mTOR and AMPK pathways, would cross-talk with chromatin dynamics. They could impact epigenetic modifications in response to changes in energy availability and nutrient status.
Inflammatory Signaling Pathways: Pathways like NF-κB and cytokine signaling pathways would connect inflammation with chromatin dynamics. Inflammatory cues could trigger changes in chromatin structure to regulate immune responses and gene expression.
Feedback Loops and Crosstalk: Signaling pathways would often form feedback loops, where chromatin modifications influence the activation or inhibition of specific signaling components. Additionally, crosstalk between pathways would allow integration of multiple signals to fine-tune chromatin dynamics responses.
Interplay with Other Biological Systems: Signaling pathways would interconnect with other biological systems such as epigenetic regulation, transcriptional machinery, and cell signaling networks. For instance, they would influence the recruitment of chromatin-modifying enzymes to specific genomic loci.
The interconnectedness and interdependence of these signaling pathways would facilitate the integration of diverse signals that guide the establishment, maintenance, and adaptation of chromatin dynamics. The crosstalk between signaling pathways and other biological systems would ensure a coordinated and context-specific response to internal and external cues, contributing to the intricate regulation of gene expression and cellular processes.
Regulatory codes necessary for maintenance and operation of chromatin dynamics
To maintain and operate chromatin dynamics, a complex set of regulatory codes and languages would need to be instantiated. These codes would govern the interactions and activities of various molecular components involved in chromatin structure and gene regulation:
Histone Modification Codes: Specific codes would dictate the addition, removal, and interpretation of histone modifications. These codes would guide the binding of reader proteins to modified histones, influencing chromatin structure and gene expression.
DNA Methylation Marks: Codes would specify the locations of DNA methylation marks, which are critical epigenetic modifications. These marks would be recognized by proteins that mediate gene silencing or activation.
Chromatin Remodeling Commands: Codes would direct the action of chromatin remodeling complexes. These commands would determine whether nucleosomes should be repositioned to open or close chromatin regions, impacting gene accessibility.
Non-Coding RNA Binding Sequences: Codes would guide non-coding RNAs to their target regions on chromatin. These sequences would enable non-coding RNAs to interact with chromatin-modifying complexes and influence gene expression.
Epigenetic Memory Signals: Codes would ensure the inheritance of epigenetic marks across cell divisions. These signals would direct the maintenance of specific chromatin modifications over time.
Feedback Loop Codes: Regulatory codes would establish feedback loops that sense the state of chromatin modifications and adjust the activities of chromatin-modifying factors accordingly. These loops would maintain the balance of chromatin dynamics.
Signal Integration Algorithms: Codes would integrate signals from various pathways into chromatin dynamics. These algorithms would interpret cues from developmental, environmental, and stress-related pathways.
Stress Response Instructions: Codes would coordinate chromatin dynamics in response to stressors. These instructions would ensure that chromatin modifications adapt to changes in cellular conditions.
Cell Differentiation Signals: Codes would define how chromatin modifications guide cell differentiation. These signals would help establish and maintain distinct chromatin states in different cell types.
Interaction Sequences for Regulatory Proteins: Regulatory proteins responsible for chromatin modifications would require specific interaction sequences. These sequences would allow these proteins to bind to chromatin and carry out their functions.
Incorporating these regulatory codes and languages would involve complex interactions, feedback loops, and coordination among various molecular players. Together, they would ensure the precise orchestration of chromatin dynamics, allowing for the dynamic regulation of gene expression and the maintenance of cellular identity and function.
How would the intricate chromatin regulatory mechanisms have to evolve to shape cell identity and developmental processes?
The intricate chromatin regulatory mechanisms play a central role in shaping cell identity and developmental processes. These mechanisms enable cells to adopt distinct fates, respond to developmental cues, and ensure proper tissue formation. Here's how these mechanisms would have had to evolve to achieve these outcomes:
Genetic Innovation and Duplication: New genes, including those encoding chromatin-modifying enzymes, transcription factors, and regulatory RNAs, could arise through gene duplication, divergence, and mutation. This genetic innovation provides the raw material for the evolution of novel chromatin regulatory functions.
Diversification of Regulatory Elements Regulatory elements, such as enhancers and promoters, could evolve or be duplicated to acquire new functions. Changes in the sequence of these elements might lead to altered binding sites for transcription factors and other regulatory proteins, enabling the fine-tuning of gene expression patterns.
Recruitment of Transcription Factors: Transcription factors, initially with general functions, could evolve to recognize specific DNA sequences associated with particular genes or cellular functions. Over time, these factors would become key players in establishing cell-specific gene expression patterns.
Coevolution of Epigenetic Marks: Epigenetic modifications and their binding proteins could coevolve to create specific interactions between histones, DNA, and regulatory proteins. This would enable the establishment of unique chromatin landscapes in different cell types, contributing to cell identity.
Epigenetic Memory Mechanisms: Epigenetic memory systems could evolve to maintain stable chromatin states during cell division. This would ensure that cells maintain their identities and gene expression profiles as they replicate and differentiate.
Integration of Signaling Pathways: Signaling pathways, which evolved to respond to environmental and developmental cues, could be integrated with chromatin regulatory networks. These pathways would instruct chromatin-modifying enzymes to modify chromatin in response to specific signals, guiding cell fate decisions.
Emergence of Long Non-Coding RNAs: Long non-coding RNAs (lncRNAs) could evolve as key regulators of chromatin dynamics. LncRNAs might interact with chromatin-modifying complexes and guide them to specific genomic regions, contributing to the establishment of distinct chromatin states.
Collaborative Gene Networks: Gene networks involving multiple regulatory elements and transcription factors could evolve to work in concert to define cell identity. These networks would enable coordinated gene expression patterns that drive cell differentiation and tissue formation.
Regulatory Robustness and Flexibility: Through evolution, chromatin regulatory mechanisms would balance robustness (maintaining cell identity) and flexibility (responding to cues). Genetic redundancy, compensatory mechanisms, and cis-regulatory element variations would contribute to this balance.
Natural Selection and Adaptation: Cells with chromatin regulatory mechanisms that confer fitness advantages—such as optimized tissue functions, better adaptation to environments, or enhanced reproductive success—would be selected over time, leading to the refinement and optimization of chromatin regulatory networks.
In summary, the evolution of intricate chromatin regulatory mechanisms involves a combination of genetic innovation, coevolution of regulatory elements and proteins, integration of signaling pathways, and the emergence of specialized regulatory RNAs. These mechanisms enable cells to establish and maintain unique chromatin states that define their identities and guide their developmental trajectories. Over time, natural selection acts on these mechanisms, leading to the diversification and specialization of cell types essential for complex organisms.
Is there scientific evidence supporting the idea that chromatin dynamics systems were brought about by the process of evolution?
The intricate choreography of chromatin dynamics presents a formidable challenge to the notion of gradual evolution. The complexity of this process, replete with its demands for the orchestration of various codes, languages, signaling systems, and proteins, leaves no room for stepwise, incremental development. The very essence of chromatin dynamics hinges on an interdependence so profound that any attempt to isolate one mechanism, language, or code system from the others renders it bereft of function. Consider, for instance, the intricate dance between histone modifications and DNA methylation. The seamless interaction between these two facets is indispensable for proper gene expression regulation. A gradualist perspective falters here, as the functional outcome only emerges when both systems are fully operational. Any intermediary stages, lacking the intricate symphony of histone modifications and DNA methylation, would not merely offer no advantage to natural selection but would pose a burden, incurring the cost of energy and resources without conferring fitness. The intricate ballet of transcription factors, promoters, enhancers, and silencers presents another enigma. For these components to exert their regulatory influence coherently, they must be instantiated simultaneously. The proposition of a stepwise evolution falls flat in the face of such a demand. Without the concerted interplay of these elements, the regulatory network remains dormant, devoid of function. It defies reason to imagine that these components, operating in isolation, could have been honed over time into a functional ensemble. A closer look at the very foundation of chromatin dynamics reveals an undeniable coalescence of intricately woven threads. The establishment of a genetic code is inextricably linked with the initiation of translation machinery. The emergence of DNA polymerase finds purpose only when in concert with the advent of DNA repair mechanisms. To envision the sequential unfolding of these components belies the very essence of their symbiotic necessity. The panorama of chromatin dynamics eludes a gradualistic genesis. The exquisite harmony between diverse elements, each with its distinct role, defies the notion of an incremental march towards functionality. The intricacy, interdependence, and instant functionality of these systems can only be attributed to an encompassing intelligence that orchestrated their simultaneous inception.
Irreducibility and Interdependence of the systems to instantiate and operate chromatin dynamics
The orchestration of chromatin dynamics is a marvel of complexity, wherein manufacturing, signaling, and regulatory codes and languages converge to shape the intricate dance of cellular function. Within this intricate tapestry, certain codes and languages are inherently irreducible, and their interdependence is palpably evident. Consider the epigenetic modifications that festoon chromatin – they are a regulatory code that profoundly influences gene expression. These modifications, such as DNA methylation and histone acetylation, are interwoven with signaling languages that transmit molecular cues, like those conveyed by methyltransferases and acetyltransferases. This interplay is critical; the manufacturing of specific epigenetic marks relies on the presence of these modifying enzymes, and their actions are precisely guided by the signaling cues. The code for epigenetic marks is meaningless without the signaling language that directs their placement. Furthermore, the regulatory codes embedded in transcription factors are intricately linked to the signaling networks they engage. Transcription factors bind to specific DNA sequences to control gene expression, but their functionality hinges on recognition motifs and cooperative interactions. These motifs, often recognized through protein-protein interactions, employ a language of shape recognition and charge complementarity. Without the precise matching of motifs and interactions, the regulatory code falls into disarray, rendering transcription factors ineffective. Crucially, these various codes and languages do not exist in isolation. They communicate, crosstalk, and collaborate to sustain functional cellular operation. The regulatory codes of transcription factors, for instance, interface with the manufacturing codes of RNA polymerase and the signaling languages of cellular responses. The cross-talk between these components is orchestrated through complex communication systems involving proteins, protein-protein interactions, and molecular gradients. The complexity of this interdependence defies a stepwise evolutionary progression. Each component's functionality emerges only in the context of the others, and any intermediary stages would lack the required synergy, rendering them non-functional and potentially detrimental to the cell's fitness. The intricate balance of signaling, manufacturing, and regulatory codes, intertwined as they are, bespeaks an intelligent design that foresaw the need for simultaneous instantiation.
The irreducible interplay of manufacturing, signaling, and regulatory codes within chromatin dynamics precludes a gradualist evolution. The intricate communication systems and interdependencies among these codes affirm the need for a holistic, fully operational foundation right from the outset, underscoring the presence of an intelligent designer.
Once chromatin dynamics are instantiated and operational, what other intra and extracellular systems is it interdependent with?
Once chromatin dynamics is instantiated and operational, it becomes intricately interdependent with a multitude of intra- and extracellular systems, each contributing to the orchestration of cellular function.
Intracellular Interdependencies
Gene Expression Machinery: The transcriptional and translational machinery collaborates closely with chromatin dynamics to ensure accurate gene expression. RNA polymerases, splicing factors, and ribosomes rely on proper chromatin structure and epigenetic marks to locate and process genes.
Epigenetic Maintenance Systems: Enzymes responsible for maintaining epigenetic marks, such as DNA methyltransferases and histone modifiers, work hand-in-hand with chromatin dynamics to perpetuate epigenetic information across cell divisions.
DNA Repair Mechanisms: DNA repair pathways are intertwined with chromatin remodeling processes. Repair enzymes need access to damaged DNA sites, and chromatin dynamics enable their interaction with the damaged regions.
Cell Cycle Regulation: Chromatin changes are pivotal during different phases of the cell cycle. Proper progression through the cell cycle depends on accurate gene expression, which is shaped by chromatin dynamics.
Cell Signaling Networks: Signaling pathways, such as those involving growth factors, hormones, and cytokines, interact with chromatin dynamics to trigger specific gene expression patterns in response to external cues.
Mitochondrial Function: The functional status of mitochondria and energy metabolism can influence chromatin structure and gene expression, showcasing the interplay between cellular energy status and chromatin dynamics.
Extracellular Interdependencies
Cell-Cell Communication: The response of a cell to its environment often involves changes in chromatin dynamics. Signaling molecules from neighboring cells can trigger epigenetic modifications and chromatin remodeling events.
Immune Responses: Immune cells can alter chromatin dynamics in response to infection or inflammation. This facilitates the activation or suppression of immune-related genes.
Developmental Pathways: During embryonic development, chromatin dynamics are tightly coordinated with signaling pathways to guide cell differentiation and tissue formation.
Stem Cell Maintenance and Differentiation: The transition of stem cells to specialized cell types relies on precise chromatin changes that dictate gene expression patterns.
Tissue Homeostasis: Proper tissue function is maintained by a balance of cell proliferation, differentiation, and apoptosis, all of which are under the influence of chromatin dynamics.
Environmental Adaptation: Chromatin changes can be responsive to environmental factors, allowing cells to adapt to changing conditions.
These intricate interdependencies further underscore the complexity and integrated nature of chromatin dynamics within the broader cellular context. The simultaneous operation of these systems and their precise coordination necessitates a comprehensive and purposeful design that goes beyond the scope of stepwise, gradual evolution.
Premise 1: Systems that rely on intricate semiotic codes and languages, and exhibit irreducible interdependencies, are unlikely to have emerged gradually through evolutionary processes due to the functional requirement of multiple components operating together.
Premise 2: Intracellular and extracellular systems, including gene expression machinery, epigenetic maintenance, DNA repair, cell cycle regulation, cell signaling networks, mitochondrial function, cell-cell communication, immune responses, developmental pathways, stem cell maintenance and differentiation, tissue homeostasis, and environmental adaptation, rely on precise semiotic codes, languages, and interdependencies.
Conclusion: Therefore, the existence and simultaneous interlocking operation of these systems strongly suggest a comprehensive and purposeful design, as their intricate, interdependent nature and requirement for coordinated operation from the outset align more with the attributes of an intelligently designed system rather than a product of stepwise, gradual evolution.
This syllogism highlights the compelling connection between the complexity of these systems, their interdependence, and the need for their simultaneous instantiation, thereby pointing towards an intelligent design as the plausible explanation for their existence and functionality.
1. Laghmach, R., Di Pierro, M., & Potoyan, D. (2021). A liquid state perspective on dynamics of chromatin compartments. Frontiers in Molecular Biology, 8, 781981. doi:10.3389/fmolb.2021.781981