Mechanobiology - how it points to design
At the molecular level, mechanobiology specifies how mechano-molecular players are recruited and interconnected together to activate a specific biological function.These phenomena are the consequence of two main events, referred to as (i) mechanosensing, or the capacity of cells to sense physical cues and mechanical forces from the surrounding microenvironment and (ii) mechanotransduction, or the capacity of the cells to transduce either external forces into biochemical signals to elicit selected cell functions or to intracellular molecular interaction into forces that influence the architecture and properties of the microenvironment.
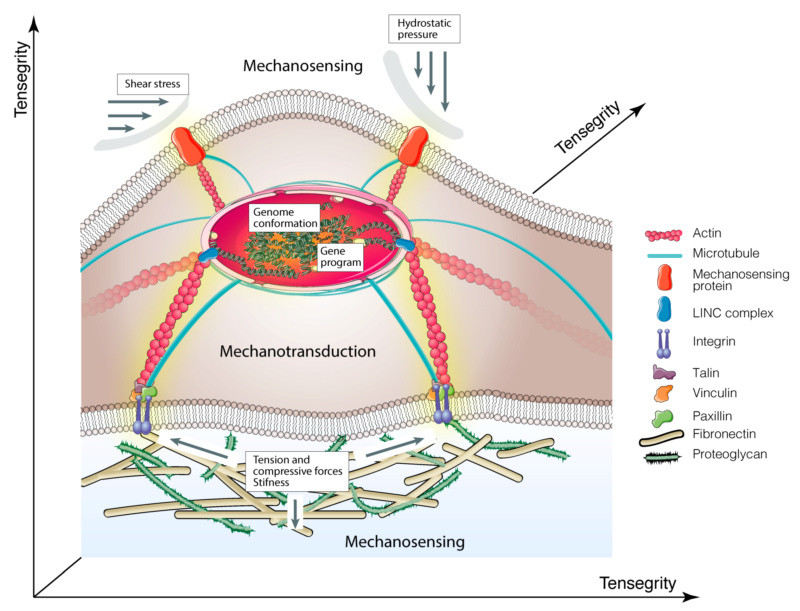
Schematic illustration of molecular basis of mechanobiology.
Cartoon shows how mechanical cues are transmitted to the nucleus via integrins > focal adhesion complex > cytoskeletal components > nucleoskeleton. The yellow shadow indicates mechanotransduction signals.
Tensegrity
Currently, the theoretical explanation of mechanobiology is based on the discovery that, in all cells, the cytoskeleton acts as a dynamic machine that collects the external forces applied to the cell from the microenvironment and responds by generating traction/compression forces transmitted to other molecular components inside or outside the cells. This model is based on the concept of “tensegrity” (tensional integrity), by which living cells organize their cytoskeleton as a hard-wired that immediately responds to external mechanical stresses stabilizing its form.
Tensegrity is a building principle, being originally described by the architect R.B. Fuller and pictured by the sculptor K. Snelson. While Fuller defined a tensegrity system “as structures that stabilize their shape by continuous tension or ‘tensional integrity’, rather than by continuous compression”, Snelson demonstrated that network structures may mechanically stabilize themselves through the use of tensile pre-stress forces. In 1993, D. Ingber applied the term “tensegrity” to living organisms, suggesting a mechanical model where the cytoskeleton structure acts as a dynamic load-bearing pillar. This model, which is capable of recapitulating the events leading to cytoskeletal mechanics, cell shape, and movement, allowed for explaining how cells sense and respond to mechanical forces and, above all, how these two events are connected. Hence, tensegrity predicts that cells respond straightaway to external mechanical stresses applied to the cells’ surface, through proteins that are physically connected to the cytoskeleton. Additionally, in this mechanical model, molecules that are activated by changes in cytoskeletal architecture function in the “solid-state” and transduce mechanical stresses into biochemical signals and gene expression program within single living cells. Therefore, all living organisms use “tensegrity” to mechanically stabilize their shape and integrate and balance their structure and function at all size scales, from the molecular level to organs. This is a consequence of cytoskeleton tension that is transduced into an equilibrium of opposing forces that are dispersed through the network of cytoskeletal filaments. Generally, tension is generated within the actomyosin contractile microfilaments and is counteracted by microtubules, which are able to resist the compression forces. The cytoskeletal components are nonlinear cell mechanical supports, introduced the concept of “initial imperfections” in the original tensegrity model. This scheme provided a new intuitive method for understanding the load-bearing capacity and distribution of force into the cytoskeleton.
Mechanosensing
As mentioned above, all organisms have structures, enabling them to recognize and respond to mechanical forces. This cross-talk takes place at the macroscale level (e.g., in organs and tissues), at the microscale level (e.g., in single cells), and also at the nanoscale level (e.g., in molecular complexes or single proteins). At present, we know that the different types of forces orchestrate the control of all biological functions, including stem cells’ commitment, determination, development, and maintenance of cells and tissues homeostasis. Table 1 summarizes the different mechanical properties and the proteins serving as transmitters in mediating these processes.
Mechanical properties and biological mediators
At the molecular level, mechanobiology specifies how mechano-molecular players are recruited and interconnected together to activate a specific biological function.These phenomena are the consequence of two main events, referred to as (i) mechanosensing, or the capacity of cells to sense physical cues and mechanical forces from the surrounding microenvironment and (ii) mechanotransduction, or the capacity of the cells to transduce either external forces into biochemical signals to elicit selected cell functions or to intracellular molecular interaction into forces that influence the architecture and properties of the microenvironment.
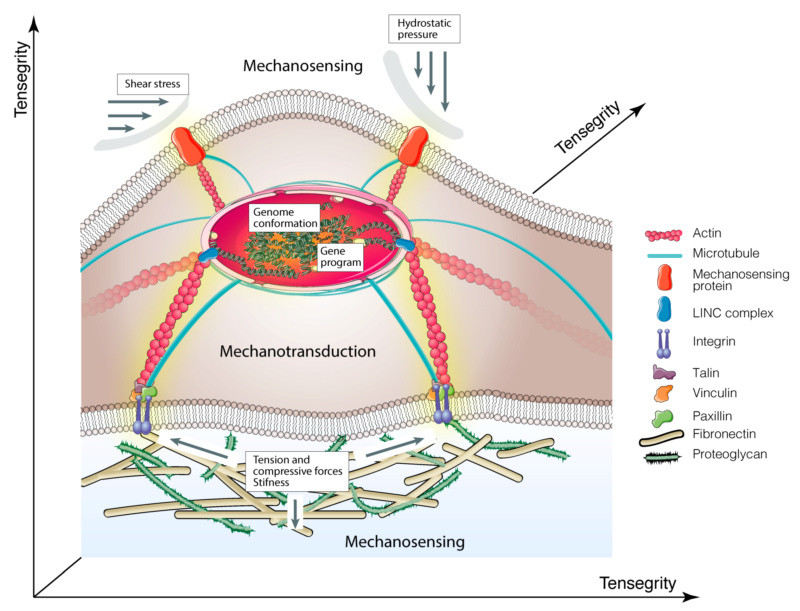
Schematic illustration of molecular basis of mechanobiology.
Cartoon shows how mechanical cues are transmitted to the nucleus via integrins > focal adhesion complex > cytoskeletal components > nucleoskeleton. The yellow shadow indicates mechanotransduction signals.
Tensegrity
Currently, the theoretical explanation of mechanobiology is based on the discovery that, in all cells, the cytoskeleton acts as a dynamic machine that collects the external forces applied to the cell from the microenvironment and responds by generating traction/compression forces transmitted to other molecular components inside or outside the cells. This model is based on the concept of “tensegrity” (tensional integrity), by which living cells organize their cytoskeleton as a hard-wired that immediately responds to external mechanical stresses stabilizing its form.
Tensegrity is a building principle, being originally described by the architect R.B. Fuller and pictured by the sculptor K. Snelson. While Fuller defined a tensegrity system “as structures that stabilize their shape by continuous tension or ‘tensional integrity’, rather than by continuous compression”, Snelson demonstrated that network structures may mechanically stabilize themselves through the use of tensile pre-stress forces. In 1993, D. Ingber applied the term “tensegrity” to living organisms, suggesting a mechanical model where the cytoskeleton structure acts as a dynamic load-bearing pillar. This model, which is capable of recapitulating the events leading to cytoskeletal mechanics, cell shape, and movement, allowed for explaining how cells sense and respond to mechanical forces and, above all, how these two events are connected. Hence, tensegrity predicts that cells respond straightaway to external mechanical stresses applied to the cells’ surface, through proteins that are physically connected to the cytoskeleton. Additionally, in this mechanical model, molecules that are activated by changes in cytoskeletal architecture function in the “solid-state” and transduce mechanical stresses into biochemical signals and gene expression program within single living cells. Therefore, all living organisms use “tensegrity” to mechanically stabilize their shape and integrate and balance their structure and function at all size scales, from the molecular level to organs. This is a consequence of cytoskeleton tension that is transduced into an equilibrium of opposing forces that are dispersed through the network of cytoskeletal filaments. Generally, tension is generated within the actomyosin contractile microfilaments and is counteracted by microtubules, which are able to resist the compression forces. The cytoskeletal components are nonlinear cell mechanical supports, introduced the concept of “initial imperfections” in the original tensegrity model. This scheme provided a new intuitive method for understanding the load-bearing capacity and distribution of force into the cytoskeleton.
Mechanosensing
As mentioned above, all organisms have structures, enabling them to recognize and respond to mechanical forces. This cross-talk takes place at the macroscale level (e.g., in organs and tissues), at the microscale level (e.g., in single cells), and also at the nanoscale level (e.g., in molecular complexes or single proteins). At present, we know that the different types of forces orchestrate the control of all biological functions, including stem cells’ commitment, determination, development, and maintenance of cells and tissues homeostasis. Table 1 summarizes the different mechanical properties and the proteins serving as transmitters in mediating these processes.
Mechanical properties and biological mediators
Tension | Tensile forces refer to the external stimuli that tend to stretch cells, acting in opposite directions, thus causing their elongation. Cellular responses to stretching depend largely on the type and amount of load as well as on the composition of the extracellular matrix. | Myosin II, integrins, FAK, F-actin, Ifs, ZO-1, E-cadherin, Lmn A/C, Arp2/3, formin, coronin 1B, a-catenin, vinculin, collagens, elastin, fibrillin, fibulin, tenascin-C, pacsin-2, F-actin, microtubules | [26,27,28,29,30] |
Compression | Contrary to tension, compressive forces applied from the outside towards the centre of cells result in cells contraction and shortening. | Collagen, vimentin, F-actin ROCK, myosin regulatory light chain, Wnt/β-catenin | [29] |
Shear Stress | When two opposite forces are tangentially applied to cells surface, they generate shear stress, which cause changes in morphology and adhesion properties. | PECAM1, VEGFR, ERK1/2, PGTS2, IER3, EGR1, IGF1, IGFBP1, Integrin, TGF-β, β-catenin, MAPK, laminin-5, F-actin, PI3K | [31,32] |
Hydrostatic Pressure | Hydrostatic pressure is the force exercised by the surrounding fluid to cells membranes. Due to its nondirectional nature, it is mainly non-deforming but has an important thermodynamic effect on the cytoskeleton influencing microtubule stability. | Shc1, integrins, collagen, TGF-β, F-actin | [32] |
Stiffness | The term stiffness, which generally is used to describe the ability of an object to resist deformation after the application of a force, is also a measure of the rigidity of the extracellular matrix or the cells were those forces are applied. | Integrin (α2), fibronectin, collagens, α-actinin, Rho signaling cascade, talin vinculin, FAK, BMP receptor, F-Actins, vimentin Ifs, microtubules, filamin, lamin-A/C, emerin, Yap1 | [28,33,34] |
Elasticity | Elasticity is the property of the object to complement its original shape and size after removal of the applied force. In biology is the resistance of cells to the extracellular matrix deformation. | Collagen VI, tenascins, titin, elastin, fibrillins, integrins, F-Actins, microtubules, Myosin II | [33] |
Viscoelasticity | It indicates the elastic and viscous properties by which an object contrasts the deformation. | Collagens, Elastin, ICAM-1, F-Actins | [35,36] |