The irreducible complex system of the eye, and eye-brain interdependence
https://reasonandscience.catsboard.com/t2631-the-irreducible-complex-system-of-the-eye-and-eye-brain-interdependence
The signal transduction pathway is irreducible complex 4
http://www.detectingdesign.com/humaneye.html
The first step in vision is the detection of photons. 5 In order to detect a photon, specialized cells use a molecule called 11-cis-retinal. When a photon of light interacts with this molecule, it changes its shape almost instantly. It is now called trans-retinal. This change in shape causes a change in shape of another molecule called rhodopsin. The new shape of rhodopsin is called metarhodopsin II. Metarhodopsin II now sticks to another protein called transducin forcing it to drop an attached molecule called GDP and pick up another molecule called GTP. The GTP-transducin-metarhodopsin II molecule now attaches to another protein called phosphodiesterase. When this happens, phosphodiesterase cleaves molecules called cGMPs. This cleavage of cGMPs reduces their relative numbers in the cell. This reduction in cGMP is sensed by an ion channel. This ion channel shuts off the ability of the sodium ion to enter the cell. This blockage of sodium entrance into the cell causes an imbalance of charge across the cell's membrane. This imbalance of charge sends an electrical current to the brain. The brain then interprets this signal and the result is called vision.
Many other proteins are now needed to convert the proteins and other molecules just mentioned back to their original forms so that they can detect another photon of light and signal the brain. If any one of these proteins or molecules is missing, even in the simplest eye system, vision will not occur.
The question now of course is, how could such a system evolve gradually? All the pieces must be in place simultaneously. For example, what good would it be for an earthworm that has no eyes to suddenly evolve the protein 11-cis-retinal in a small group or "spot" of cells on its head? These cells now have the ability to detect photons, but so what? What benefit is that to the earthworm? Now, lets say that somehow these cells develop all the needed proteins to activate an electrical charge across their membranes in response to a photon of light striking them. So what?! What good is it for them to be able to establish an electrical gradient across their membranes if there is no nervous pathway to the worm's minute brain? Now, what if this pathway did happen to suddenly evolve and such a signal could be sent to the worm's brain. So what?! How is the worm going to know what to do with this signal? It will have to learn what this signal means. Learning and interpretation are very complicated processes involving a great many other proteins in other unique systems. Now the earthworm, in one lifetime, must evolve the ability to pass on this ability to interpret vision to its offspring. If it does not pass on this ability, the offspring must learn as well or vision offers no advantage to them. All of these wonderful processes need regulation. No function is beneficial unless it can be regulated (turned off and on). If the light sensitive cells cannot be turned off once they are turned on, vision does not occur. This regulatory ability is also very complicated involving a great many proteins and other molecules - all of which must be in place initially for vision to be beneficial.
Photoreceptor cells point to intelligent design
https://reasonandscience.catsboard.com/t1696-photoreceptor-cells-point-to-intelligent-design
How the origin of the human eye is best explained through intelligent design
https://reasonandscience.catsboard.com/t2411-how-the-origin-of-the-human-eye-is-best-explained-through-intelligent-design
Signal transduction pathway
The absorption of light leads to an isomeric change in the retinal molecule.
The signal transduction pathway is the mechanism by which the energy of a photon signals a mechanism in the cell that leads to its electrical polarization. This polarization ultimately leads to either the transmittance or inhibition of a neural signal that will be fed to the brain via the optic nerve. The steps, or signal transduction pathway, in the vertebrate eye's rod and cone photoreceptors are then:
1.The rhodopsin or iodopsin in the disc membrane of the outer segment absorbs a photon, changing the configuration of a retinal Schiff base cofactor inside the protein from the cis-form to the trans-form, causing the retinal to change shape.
2.This results in a series of unstable intermediates, the last of which binds stronger to the G protein in the membrane and activates transducin, a protein inside the cell. This is the first amplification step – each photoactivated rhodopsin triggers activation of about 100 transducins. (The shape change in the opsin activates a G protein called transducin.)
3.Each transducin then activates the enzyme cGMP-specific phosphodiesterase (PDE).
4.PDE then catalyzes the hydrolysis of cGMP to 5' GMP. This is the second amplification step, where a single PDE hydrolyses about 1000 cGMP molecules.
5.The net concentration of intracellular cGMP is reduced (due to its conversion to 5' GMP via PDE), resulting in the closure of cyclic nucleotide-gated Na+ ion channels located in the photoreceptor outer segment membrane.
6.As a result, sodium ions can no longer enter the cell, and the photoreceptor outer segment membrane becomes hyperpolarized, due to the charge inside the membrane becoming more negative.
7.This change in the cell's membrane potential causes voltage-gated calcium channels to close. This leads to a decrease in the influx of calcium ions into the cell and thus the intracellular calcium ion concentration falls.
8.A decrease in the intracellular calcium concentration means that less glutamate is released via calcium-induced exocytosis to the bipolar cell (see below). (The decreased calcium level slows the release of the neurotransmitter glutamate, which can either excite or inhibit the postsynaptic bipolar cells.)
9.Reduction in the release of glutamate means one population of bipolar cells will be depolarized and a separate population of bipolar cells will be hyperpolarized, depending on the nature of receptors (ionotropic or metabotropic) in the postsynaptic terminal (see receptive field).
The eyespot apparatus/flagella are interdependent
Eyespots are the simplest and most common "eyes" found in nature, composed of photoreceptors and areas of bright orange-red pigment granules.
Euglena possess a red eyespot, an organelle composed of carotenoid pigment granules. The red spot itself is not thought to be photosensitive. Rather, it filters the sunlight that falls on a light-detecting structure at the base of the flagellum (a swelling, known as the paraflagellar body), allowing only certain wavelengths of light to reach it. As the cell rotates with respect to the light source, the eyespot partially blocks the source, permitting the Euglena to find the light and move toward it (a process known as phototaxis
Phototaxis is a kind of taxis, or locomotory movement, that occurs when a whole organism moves towards or away from stimulus of light.[1] This is advantageous for phototrophic organisms as they can orient themselves most efficiently to receive light for photosynthesis. Phototaxis is called positive if the movement is in the direction of increasing light intensity and negative if the direction is opposite.
so their light sensitiveness has actually nothing to do with seeing. It has a entirely different purpose, namely moves towards or away from stimulus of light . It does not need a brain for doing so.
In order to function, the euglena needs a red eyespot, and a phototaxis receptor. Thats already a interdependent and irreducible complex system system.
the photoreceptors in euglena have the goal to trigger movement in the flagella in order the bacterias to move closer to the light source for photsynthesis. Several parts in the cell are required to accomplish this task, namely : Excitation of this receptor protein results in the formation of cyclic adenosine monophosphate (cAMP) as a second messenger. Chemical signal transduction ultimately triggers changes in flagellar beat patterns and cell movement. No messenger, no movement, no task accomplished...
At its simplest, the eye incorporates three functions:
Light detection
Shading, in the form of dark pigment, for sensing the direction light is coming from
Connection to motor structures, for movement in response to light
Euglena need
1. a eye spot,
2. receptor proteins, and their exitations results in cyclic adenosine monophosphate (cAMP) . that will result in beat patterns of
3. the flagella for cell movement. That IS already a irreducible/interdependent complex system.
In some organisms, all three of these functions are carried out by just one cell—the single-celled euglena is one example. It has a light-sensitive spot, pigment granules for shading, and motor cilia. This structure, however, isn't considered a true eye.
The most-basic structure that is widely accepted as an eye has just two cells: a photoreceptor that detects light, and a pigment cell that provides shading. The photoreceptor connects to ciliated cells, which engage to move the animal in response to light. The marine ragworm embryo (right) has a two-celled eye.
if they are not there all at once right from the beginning, even the simplest eye will not work. That is already a irreducible complex system.
Photoreception is a dominant sensory tool found in the majority of taxa across the Metazoa. Photoreceptive organs range in complexity from a single photoreceptor cell, pigmented eyespots and cups, to complicated organs that focus, reflect and absorb light in order to resolve images. Very little is known about how these structures develop and how a simple photoreceptive organ can evolve and elaborate into a more complicated form. 6
96% of animal species have eyes.
http://learn.genetics.utah.edu/content/selection/eye/
Despite much progress, we are at the early stages of fully describing the organization of the human visual system, and we know little about the visual systems of apes.
How did evolution “know” that two eyes were required for 3D vision, or that there was 3D vision in the first place?

cornea
aqueous humour
pupil
retina
iris
vitreous humour
ganglion cells
conjunctive
cilary muscles
muscle
ocular vein
sclera
choroid
central retina artery
optic nerve sheath
central retinal vein
http://samedical.blogspot.com.br/2010/08/nervous-system-eye.html
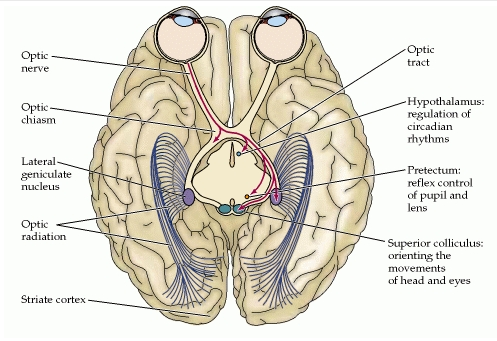
Axons which form the optic nerve
Crossed fibers
Uncrossed fibers
optic tract
commissure of gudden
Pulvinar
Lateral geniculate body
superior colliculus
medial geniculate body
nucleus of oculomotor nerve
nucleus of trochlar nerve
nucleus of abducent nerve
striate cortex
Optic radiation
Axons
An axon is a long, branching cell structure that is unique to nerve cells. Like all animal cells, nerve cells — also known as neurons — are covered with a semi-permeable membrane, and it is this membrane that makes up the axons. Axons are responsible for carrying information from the nerve cells to all the other cells of the body. Interference with signals as they travel through the axons has been identified as a cause of certain degenerative neurological disorders.
The neuron itself is composed of three basic structures: the cell body, the axon, and numerous branching dendrites. The cell body houses the nucleus and other organelles. The dendrites collect information from other parts of the body and carry it into the neuron. The axon carries electrical impulses from the neuron to all the other cells of the body. A fatty sheath that covers the structure for the entirety of its length serves to insulate the electrical signals from interference. Known as the myelin sheath, this protective covering is composed primarily of fat cells, and is responsible for the characteristic whitish color of neural tissue.
Optic nerve
The optic nerve, also known as cranial nerve II, transmits visual information from the retina to the brain. 1,2 million neurons make up the optic nerve, where incredibly complex electro-chemical codes are formed. These electro-chemical codes are transported to the thalamus of the brain via the optic nerve.
Click on the lower left arrow to see how evolutionists think eyes evolved. Notice how the optic nerve, the immensely complex visual code, and visual cortex are ignored as part of the evolutionary sequence. They are just there in the video, but not even mentioned.
The mixed fibers from the two nerves are continued in the optic tracts, the primary visual centers of the brain. From its mode of development, and from its structure, the optic nerve must be regarded as a prolongation of the brain substance, rather than as an ordinary cerebrospinal nerve.
The optic nerve is a thick bundle of about 1.2 million individual nerve fibers. It travels from the retina to the brain’s vision center, carrying electrical data that the brain will interpret as images.
The individual fibers come from the retina’s photosensitive cells – the rods and cones. Please see our page on The Retina for more information. These cells receive the light that travels into the eye through the cornea, the pupil, the lens and then the vitreous gel. They convert the light data to electrical data so that the nerve fibers can pick it up.
The optic nerve fibers travel across the retina and converge near its center. Their convergence forms the optic nerve. At this location there are no light-sensitive cells, since the nerve fibers are taking up the space. This is the eye’s blind spot. It is near the macula, the retinal area giving us our sharpest, bright-light vision. We use it for reading or any close work where small details are important.
Crossed fibers
The crossed fibers of the optic nerve tend to occupy the medial side of the nerve and the uncrossed fibers the lateral side. In the optic tract, however, the fibers are much more intermingled.
Pulvinar
To effectively interact with our environment, body movements must be coordinated with the perception of visual movement. We will present evidence that regions of the pulvinar nucleus that receive input from the superior colliculus (tectum) may be involved in this process.
Lateral geniculate body
The lateral geniculate nucleus (LGN) is the primary relay center for visual information received from the retina of the eye.The functions of the LGN are multiple. Its unique folding contributes to its utility by performing a range of anatomical calculations without requiring mathematical computations. These include both temporal correlations/decorrelations as well as spatial correlations. The resulting outputs include time correlated and spatially correlated signals resulting from summing the signals received from the left and right semifields of view captured by each of the two eyes. These signals are correlated in order to achieve a three-dimensional representation of object space as well as obtain information for controlling the precision (previously auxiliary) optical system (POS) of the visual modality.
The distribution of the LGN's neurons into various layers suggests that some distinct aspects of the visual information from the retina may be processed separately in this synaptic relay. And that is exactly what has been demonstrated experimentally.
http://thebrain.mcgill.ca/flash/i/i_02/i_02_cr/i_02_cr_vis/i_02_cr_vis.html
superior colliculus
The general function of the tectal system is to direct behavioral responses toward specific points in egocentric ("body-centered") space. Each layer of the tectum contains a topographic map of the surrounding world in retinotopic coordinates, and activation of neurons at a particular point in the map evokes a response directed toward the corresponding point in space.
pretectum
The pretectal area, or pretectum, is a midbrain structure composed of seven nuclei and comprises part of the subcortical visual system. Through reciprocal bilateral projections from the retina, it is involved primarily in mediating behavioral responses to acute changes in ambient light such as the pupillary light reflex, the optokinetic reflex, and temporary changes to the circadian rhythm.
superior colliculus
The superior colliculus is a set of two bumps on the dorsal side of the midbrain. A larger region, the optic tectum, is formed by this structure and the inferior colliculus. Sometimes, the superior colliculus is referred to simply as the tectum. Unlike the inferior colliculus, which is involved in hearing, the tectum plays a role in processing vision.
This structure is involved with visual reflexes. Both the visual cortex and the retina of the eye itself project information to the outer layers of the tectum. Intermediate layers also receive sensory input from both visual and auditory neurons, as well as input from motor centers. The deepest layers receive mainly motor input, and can even direct eye movement and other motor actions. This wide variety of input types helps this structure to move the head and eyes toward sensory stimuli.
In each layer, neurons of the superior colliculus are arranged in a map. This representational map is aligned with retinal cells. Functionally, this allows activation of different retinal cells, triggering a corresponding response on the map. The tectum can then orient the eyes and head in the same direction the stimuli appeared.
Thalamocortical radiations
The optic radiation (also known as the geniculo-calcarine tract or as the geniculostriate pathway or recently posterior thalamic radiation) is a collection of axons from relay neurons in the lateral geniculate nucleus of the thalamus carrying visual information through two divisions (called Upper and Lower division) to the visual cortex (also called striate cortex) along the calcarine fissure.
Striate or visual cortex
The visual cortex of the brain is the part of the cerebral cortex responsible for processing visual information. It is located in the occipital lobe, in the back of the brain.
All visual information that the human mind receives is processed by a part of the brain known as visual cortex. The visual cortex is part of the outermost layer of the brain, the cortex, and is located at the dorsal pole of the occipital lobe; more simply put, at the lower rear of the brain. The visual cortex obtains its information via projections that extend all the way through the brain from the eyeballs. The projections first pass through a stopover point in the middle of the brain, an almond-like lump known as the Lateral Geniculate Nucleus, or LGN. From there they are projected to the visual cortex for processing.

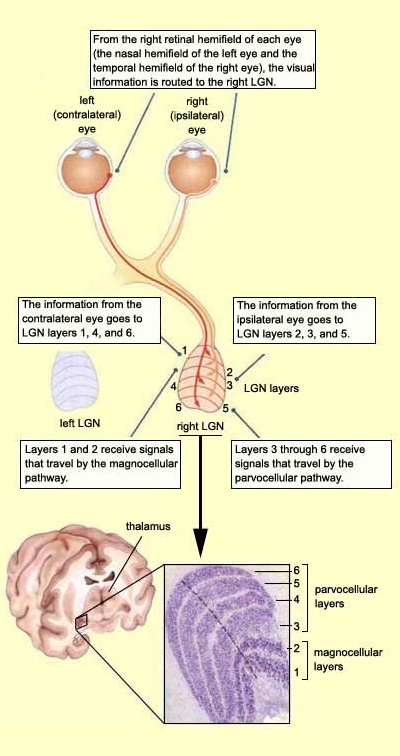
The Purpose of Our Eyes' Strange Wiring Is Unveiled
http://www.scientificamerican.com/article/the-purpose-of-our-eyes-strange-wiring-is-unveiled/
These results mean that the retina of the eye has been optimised so that the sizes and densities of glial cells match the colours to which the eye is sensitive (which is in itself an optimisation process suited to our needs). This optimisation is such that colour vision during the day is enhanced, while night-time vision suffers very little. The effect also works best when the pupils are contracted at high illumination, further adding to the clarity of our colour vision.
We construct a light-guiding model of the retina outside the fovea, in which an array of glial (Muller) cells permeates the depth of the retina down to the photoreceptors. Based on measured refractive indices, we propagate light to obtain a significant increase of the intensity at the photoreceptors. For pupils up to 6 mm width, the coupling between neighboring cells is only a few percent. Low cross talk over the whole visible spectrum also explains the insensitivity to chromatic aberrations of the eye. The retina is revealed as an optimal structure designed for improving the sharpness of images.
Mystery of the reverse-wired eyeball solved
http://medicalxpress.com/news/2015-02-mystery-reverse-wired-eyeball.html
From a practical standpoint, the wiring of the human eye doesn't make a lot of sense. In vertebrates, photoreceptors are located behind the neurons in the back of the eye - resulting in light scattering by the nervous fibers and blurring of our vision. Recently, researchers at the Technion - Israel Institute of Technology have confirmed the biological purpose for this seemingly counterintuitive setup. "The retina is not just the simple detector and neural image processor, as believed until today," said Erez Ribak, a professor at the Technion - Israel Institute of Technology. "Its optical structure is optimized for our vision purposes." Ribak and his co-authors will describe their work during the 2015 American Physical Society March Meeting, on Thursday, March 5 in San Antonio, Texas. Ribak's interest in the optical structure of the retina stems from his previous work applying astrophysics and astronomy techniques to improve the ability of scientists and ophthalmologists to view the retina at high detail. Previous experiments with mice had suggested that Müller glia cells, a type of metabolic cell that crosses the retina, play an essential role in guiding and focusing light scattered throughout the retina. To test this, Ribak and his colleagues ran computer simulations and in-vitro experiments in a mouse model to determine whether colors would be concentrated in these metabolic cells. They then used confocal microscopy to produce three-dimensional views of the retinal tissue, and found that the cells were indeed concentrating light into the photoreceptors."For the first time, we've explained why the retina is built backwards, with the neurons in front of the photoreceptors, rather than behind them," Ribak said. Future research for Ribak and his colleagues includes using water-filled goggles to reduce corneal aberrations, allowing observers to gain a finer view of the retina at depth.
Alleged dysteleology of the human eye—the ‘backwards’—vascularized retina
http://creation.com/evolutions-witness-how-eyes-evolved-review
The blind spot, which poses no problems whatsoever for human vision.
One common argument against an Intelligent Designer is the one about the ‘mistake’ of the retinal blood vessels situated above the surface of the retina, ‘obstructing’ the incoming light, as well as the existence of the blind spot. This argument, even on its own terms, has no merit.
Although Schwab does not address this issue in terms of ‘bad’ design, he does provide extensive detail on the vascularization of retinas in the Animal Kingdom and includes a very helpful diagram (p. 250) for comparing and contrasting the different forms of vascularization. The fact that so many different designs of vascular systems exist in the Animal Kingdom demonstrates, if nothing else, that there is no ‘right’ or ‘wrong’ way to construct such a system. Consequently, theological issues aside, it is meaningless to begin even to speak of the vascular system, in the human eye, as a form of bad design. Finally, as elaborated in the next section, whatever the anatomical and physiological limitations of the human eye, they are compensated by the extensive interpretive powers of the human brain.
If a change in selective pressures favored a dimpled eyespot with a slight increase in visual acuity, pretty soon the majority of the population would have dimpled eyespots. The problem with this notion is that no population of creatures with flat eyespots shows any sort of intra-population range like this were even a small portion of the population has dimpled eyespots to any selectable degree. This is a common assertion, but it just isn't true.
Now, if these 1,829 gradations really evolutionary steps that are in fact small enough to cross in fairly short order (a few generations each under selective conditions), it seems quite likely that such ranges in morphologic expression would be seen within a single gene pool of a single species. But, they aren't. Species that have simple flat light-sensitive eyespots only have flat light-sensitive eyespots. No individual within that species shows any sort of dimpled eye that would have any selective advantage with regard to increased visual acuity. This fact alone suggests that these seemingly small steps probably aren't that simple when it comes to the coordinated underlying genetic changes that would be needed to get from one step to the next.
A big problem with these morphologic steps is that they do not take into consideration the fact that vision is more involved than what goes on just within the eye. In order to take any advantage of improved visual acuity within the eye, the brain must also change in such a way that it is able to interpret the information the eye is sending it. Otherwise, if the brain is still step up to appreciate only differences in light from dark sent from the eye, without being able to interpret specific patterns of light and dark on the retina, there would be no selective advantage from a dimpled vs. a flat eyespot. Because of this requirement, whatever evolution happens to take place in the eye, must be backed up by equivalent evolution in brain development and interpretive powers.
Another interesting problem with the argument for a selective advantage for a dimpled eye over a flat eyespot is the fact that determining the general direction of a light source can be achieved with a flat eyespot. Dimpling is not needed to determine the relative direction from which a beam of light is coming. All that is needed is an ability to rotate the eyespot relative to the source of light combined with the brain's ability to associate differences in the intensity of light with the change in orientation of the eyespot relative to the source of light. This sort of associative ability could produce essentially the same effect of being able to localize and even follow or move toward a source of light without the need for producing a dimpled or cup-shaped eye. In fact, the species Euglena, with just a flat patch of light-sensitive cells, can swim toward a source of light - - no dimpling needed ( Link ). In fact, some creatures, like starfish and sea urchin have no eyespots at all yet are still sensitive to light to the degree that they can move toward sources of greater light intensity
‘How eyes developed’ is a sweeping generalization. Exactly how the genetic mechanism creates and incorporates neural systems to conduct visual data to various parts of the brain in a coordinated fashion is glossed over, and ignored completely.
The eye is not just a camera. Our vision field is a compilation of multiple retinal layers, dedicated to separate registration of motion in various direction and velocities, and also integrated into memory systems, as well as voluntary and autonomic muscular responses. Ultra-complex hierarchies do not appear as a result of random actions.
Had Darwin been aware of the enormous complexity of only the eye, and its relation to the brain, it would have been a formidable impediment to the generation of his general theory.
There is no such thing as a ‘naturalistic ‘need’ to create an eyes in the first place, and to further complexify its function.
1) http://evillusion.wordpress.com/sight-and-sound-a-daunting-task-for-evolution/
2) http://www.reviewevolution.com/press/pressRelease_EyeEvolution.php
3) http://www.grisda.org/origins/21039.htm
4) http://www.detectingdesign.com/humaneye.html
5) http://www.detectingdesign.com/humaneye.html
6) http://www.sicb.org/meetings/2016/schedule/abstractdetails.php?id=1275
https://reasonandscience.catsboard.com/t2631-the-irreducible-complex-system-of-the-eye-and-eye-brain-interdependence
The signal transduction pathway is irreducible complex 4
http://www.detectingdesign.com/humaneye.html
The first step in vision is the detection of photons. 5 In order to detect a photon, specialized cells use a molecule called 11-cis-retinal. When a photon of light interacts with this molecule, it changes its shape almost instantly. It is now called trans-retinal. This change in shape causes a change in shape of another molecule called rhodopsin. The new shape of rhodopsin is called metarhodopsin II. Metarhodopsin II now sticks to another protein called transducin forcing it to drop an attached molecule called GDP and pick up another molecule called GTP. The GTP-transducin-metarhodopsin II molecule now attaches to another protein called phosphodiesterase. When this happens, phosphodiesterase cleaves molecules called cGMPs. This cleavage of cGMPs reduces their relative numbers in the cell. This reduction in cGMP is sensed by an ion channel. This ion channel shuts off the ability of the sodium ion to enter the cell. This blockage of sodium entrance into the cell causes an imbalance of charge across the cell's membrane. This imbalance of charge sends an electrical current to the brain. The brain then interprets this signal and the result is called vision.
Many other proteins are now needed to convert the proteins and other molecules just mentioned back to their original forms so that they can detect another photon of light and signal the brain. If any one of these proteins or molecules is missing, even in the simplest eye system, vision will not occur.
The question now of course is, how could such a system evolve gradually? All the pieces must be in place simultaneously. For example, what good would it be for an earthworm that has no eyes to suddenly evolve the protein 11-cis-retinal in a small group or "spot" of cells on its head? These cells now have the ability to detect photons, but so what? What benefit is that to the earthworm? Now, lets say that somehow these cells develop all the needed proteins to activate an electrical charge across their membranes in response to a photon of light striking them. So what?! What good is it for them to be able to establish an electrical gradient across their membranes if there is no nervous pathway to the worm's minute brain? Now, what if this pathway did happen to suddenly evolve and such a signal could be sent to the worm's brain. So what?! How is the worm going to know what to do with this signal? It will have to learn what this signal means. Learning and interpretation are very complicated processes involving a great many other proteins in other unique systems. Now the earthworm, in one lifetime, must evolve the ability to pass on this ability to interpret vision to its offspring. If it does not pass on this ability, the offspring must learn as well or vision offers no advantage to them. All of these wonderful processes need regulation. No function is beneficial unless it can be regulated (turned off and on). If the light sensitive cells cannot be turned off once they are turned on, vision does not occur. This regulatory ability is also very complicated involving a great many proteins and other molecules - all of which must be in place initially for vision to be beneficial.
Photoreceptor cells point to intelligent design
https://reasonandscience.catsboard.com/t1696-photoreceptor-cells-point-to-intelligent-design
How the origin of the human eye is best explained through intelligent design
https://reasonandscience.catsboard.com/t2411-how-the-origin-of-the-human-eye-is-best-explained-through-intelligent-design
Signal transduction pathway
The absorption of light leads to an isomeric change in the retinal molecule.
The signal transduction pathway is the mechanism by which the energy of a photon signals a mechanism in the cell that leads to its electrical polarization. This polarization ultimately leads to either the transmittance or inhibition of a neural signal that will be fed to the brain via the optic nerve. The steps, or signal transduction pathway, in the vertebrate eye's rod and cone photoreceptors are then:
1.The rhodopsin or iodopsin in the disc membrane of the outer segment absorbs a photon, changing the configuration of a retinal Schiff base cofactor inside the protein from the cis-form to the trans-form, causing the retinal to change shape.
2.This results in a series of unstable intermediates, the last of which binds stronger to the G protein in the membrane and activates transducin, a protein inside the cell. This is the first amplification step – each photoactivated rhodopsin triggers activation of about 100 transducins. (The shape change in the opsin activates a G protein called transducin.)
3.Each transducin then activates the enzyme cGMP-specific phosphodiesterase (PDE).
4.PDE then catalyzes the hydrolysis of cGMP to 5' GMP. This is the second amplification step, where a single PDE hydrolyses about 1000 cGMP molecules.
5.The net concentration of intracellular cGMP is reduced (due to its conversion to 5' GMP via PDE), resulting in the closure of cyclic nucleotide-gated Na+ ion channels located in the photoreceptor outer segment membrane.
6.As a result, sodium ions can no longer enter the cell, and the photoreceptor outer segment membrane becomes hyperpolarized, due to the charge inside the membrane becoming more negative.
7.This change in the cell's membrane potential causes voltage-gated calcium channels to close. This leads to a decrease in the influx of calcium ions into the cell and thus the intracellular calcium ion concentration falls.
8.A decrease in the intracellular calcium concentration means that less glutamate is released via calcium-induced exocytosis to the bipolar cell (see below). (The decreased calcium level slows the release of the neurotransmitter glutamate, which can either excite or inhibit the postsynaptic bipolar cells.)
9.Reduction in the release of glutamate means one population of bipolar cells will be depolarized and a separate population of bipolar cells will be hyperpolarized, depending on the nature of receptors (ionotropic or metabotropic) in the postsynaptic terminal (see receptive field).
The eyespot apparatus/flagella are interdependent
Eyespots are the simplest and most common "eyes" found in nature, composed of photoreceptors and areas of bright orange-red pigment granules.
Euglena possess a red eyespot, an organelle composed of carotenoid pigment granules. The red spot itself is not thought to be photosensitive. Rather, it filters the sunlight that falls on a light-detecting structure at the base of the flagellum (a swelling, known as the paraflagellar body), allowing only certain wavelengths of light to reach it. As the cell rotates with respect to the light source, the eyespot partially blocks the source, permitting the Euglena to find the light and move toward it (a process known as phototaxis
Phototaxis is a kind of taxis, or locomotory movement, that occurs when a whole organism moves towards or away from stimulus of light.[1] This is advantageous for phototrophic organisms as they can orient themselves most efficiently to receive light for photosynthesis. Phototaxis is called positive if the movement is in the direction of increasing light intensity and negative if the direction is opposite.
so their light sensitiveness has actually nothing to do with seeing. It has a entirely different purpose, namely moves towards or away from stimulus of light . It does not need a brain for doing so.
In order to function, the euglena needs a red eyespot, and a phototaxis receptor. Thats already a interdependent and irreducible complex system system.
the photoreceptors in euglena have the goal to trigger movement in the flagella in order the bacterias to move closer to the light source for photsynthesis. Several parts in the cell are required to accomplish this task, namely : Excitation of this receptor protein results in the formation of cyclic adenosine monophosphate (cAMP) as a second messenger. Chemical signal transduction ultimately triggers changes in flagellar beat patterns and cell movement. No messenger, no movement, no task accomplished...
At its simplest, the eye incorporates three functions:
Light detection
Shading, in the form of dark pigment, for sensing the direction light is coming from
Connection to motor structures, for movement in response to light
Euglena need
1. a eye spot,
2. receptor proteins, and their exitations results in cyclic adenosine monophosphate (cAMP) . that will result in beat patterns of
3. the flagella for cell movement. That IS already a irreducible/interdependent complex system.
In some organisms, all three of these functions are carried out by just one cell—the single-celled euglena is one example. It has a light-sensitive spot, pigment granules for shading, and motor cilia. This structure, however, isn't considered a true eye.
The most-basic structure that is widely accepted as an eye has just two cells: a photoreceptor that detects light, and a pigment cell that provides shading. The photoreceptor connects to ciliated cells, which engage to move the animal in response to light. The marine ragworm embryo (right) has a two-celled eye.
if they are not there all at once right from the beginning, even the simplest eye will not work. That is already a irreducible complex system.
Photoreception is a dominant sensory tool found in the majority of taxa across the Metazoa. Photoreceptive organs range in complexity from a single photoreceptor cell, pigmented eyespots and cups, to complicated organs that focus, reflect and absorb light in order to resolve images. Very little is known about how these structures develop and how a simple photoreceptive organ can evolve and elaborate into a more complicated form. 6
96% of animal species have eyes.
http://learn.genetics.utah.edu/content/selection/eye/
Despite much progress, we are at the early stages of fully describing the organization of the human visual system, and we know little about the visual systems of apes.
How did evolution “know” that two eyes were required for 3D vision, or that there was 3D vision in the first place?
In order to take any advantage of improved visual acuity within the eye, the brain must also change in such a way that it is able to interpret the information the eye is sending it. Otherwise, if the brain is still step up to appreciate only differences in light from dark sent from the eye, without being able to interpret specific patterns of light and dark on the retina, there would be no selective advantage from a dimpled vs. a flat eyespot for example Because of this requirement, whatever evolution happens to take place in the eye, must be backed up by equivalent evolution in brain development and interpretive powers.
The main optical components of the eye are as follows. First comes the cornea, the transparent, slightly convex outer surface at the centre of the eye. The cornea does not have any blood vessels, so its takes its nutrients from the fluid behind it, known as the aqueous humour, as well as from the fluid in front of it, the tears, which are spread across your cornea when you blink your eyelid.
Next comes the pupil, the opening that lets light enter the eye and ultimately reach the retina. The pupil appears black because of the layer of black pigmented cells that line the back of the eye and absorb the light.
The diameter of the pupil is controlled by the iris, a circular muscle whose pigmentation gives the eye its colour and whose contraction lets the eye adapt continuously to changing light conditions. On a dark night, your pupils are big and black, because your irises open wide to let in as much as possible of the little light available. This reaction is called the pupillary reflex. You can observe it easily yourself, by watching your eyes in a mirror while you turn a nearby light on and off.
After passing through the pupil, the light goes on through the lens, which is suspended between the aqueous humour and the vitreous humour, the fluid that fills the inside of the eye.
The lens in turn focuses the light rays onto the retina, lining the back of the eye. The retina converts the image formed by the light rays into nerve impulses. The optic nerve, composed of the axons of the retina's ganglion cells, then transmits these impulses from the eye to the first visual relay in the brain.

cornea
aqueous humour
pupil
retina
iris
vitreous humour
ganglion cells
conjunctive
cilary muscles
muscle
ocular vein
sclera
choroid
central retina artery
optic nerve sheath
central retinal vein
http://samedical.blogspot.com.br/2010/08/nervous-system-eye.html
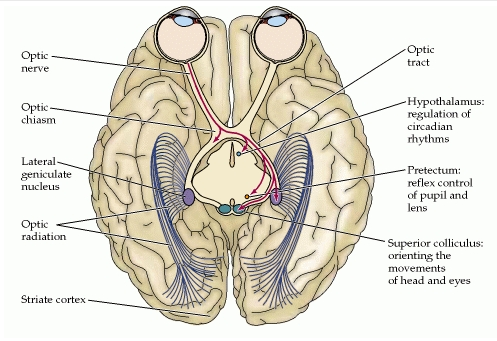
Axons which form the optic nerve
Crossed fibers
Uncrossed fibers
optic tract
commissure of gudden
Pulvinar
Lateral geniculate body
superior colliculus
medial geniculate body
nucleus of oculomotor nerve
nucleus of trochlar nerve
nucleus of abducent nerve
striate cortex
Optic radiation
Axons
An axon is a long, branching cell structure that is unique to nerve cells. Like all animal cells, nerve cells — also known as neurons — are covered with a semi-permeable membrane, and it is this membrane that makes up the axons. Axons are responsible for carrying information from the nerve cells to all the other cells of the body. Interference with signals as they travel through the axons has been identified as a cause of certain degenerative neurological disorders.
The neuron itself is composed of three basic structures: the cell body, the axon, and numerous branching dendrites. The cell body houses the nucleus and other organelles. The dendrites collect information from other parts of the body and carry it into the neuron. The axon carries electrical impulses from the neuron to all the other cells of the body. A fatty sheath that covers the structure for the entirety of its length serves to insulate the electrical signals from interference. Known as the myelin sheath, this protective covering is composed primarily of fat cells, and is responsible for the characteristic whitish color of neural tissue.
Optic nerve
The optic nerve, also known as cranial nerve II, transmits visual information from the retina to the brain. 1,2 million neurons make up the optic nerve, where incredibly complex electro-chemical codes are formed. These electro-chemical codes are transported to the thalamus of the brain via the optic nerve.
Click on the lower left arrow to see how evolutionists think eyes evolved. Notice how the optic nerve, the immensely complex visual code, and visual cortex are ignored as part of the evolutionary sequence. They are just there in the video, but not even mentioned.
The mixed fibers from the two nerves are continued in the optic tracts, the primary visual centers of the brain. From its mode of development, and from its structure, the optic nerve must be regarded as a prolongation of the brain substance, rather than as an ordinary cerebrospinal nerve.
The optic nerve is a thick bundle of about 1.2 million individual nerve fibers. It travels from the retina to the brain’s vision center, carrying electrical data that the brain will interpret as images.
The individual fibers come from the retina’s photosensitive cells – the rods and cones. Please see our page on The Retina for more information. These cells receive the light that travels into the eye through the cornea, the pupil, the lens and then the vitreous gel. They convert the light data to electrical data so that the nerve fibers can pick it up.
The optic nerve fibers travel across the retina and converge near its center. Their convergence forms the optic nerve. At this location there are no light-sensitive cells, since the nerve fibers are taking up the space. This is the eye’s blind spot. It is near the macula, the retinal area giving us our sharpest, bright-light vision. We use it for reading or any close work where small details are important.
Crossed fibers
The crossed fibers of the optic nerve tend to occupy the medial side of the nerve and the uncrossed fibers the lateral side. In the optic tract, however, the fibers are much more intermingled.
Pulvinar
To effectively interact with our environment, body movements must be coordinated with the perception of visual movement. We will present evidence that regions of the pulvinar nucleus that receive input from the superior colliculus (tectum) may be involved in this process.
Lateral geniculate body
The lateral geniculate nucleus (LGN) is the primary relay center for visual information received from the retina of the eye.The functions of the LGN are multiple. Its unique folding contributes to its utility by performing a range of anatomical calculations without requiring mathematical computations. These include both temporal correlations/decorrelations as well as spatial correlations. The resulting outputs include time correlated and spatially correlated signals resulting from summing the signals received from the left and right semifields of view captured by each of the two eyes. These signals are correlated in order to achieve a three-dimensional representation of object space as well as obtain information for controlling the precision (previously auxiliary) optical system (POS) of the visual modality.
The distribution of the LGN's neurons into various layers suggests that some distinct aspects of the visual information from the retina may be processed separately in this synaptic relay. And that is exactly what has been demonstrated experimentally.
http://thebrain.mcgill.ca/flash/i/i_02/i_02_cr/i_02_cr_vis/i_02_cr_vis.html
superior colliculus
The general function of the tectal system is to direct behavioral responses toward specific points in egocentric ("body-centered") space. Each layer of the tectum contains a topographic map of the surrounding world in retinotopic coordinates, and activation of neurons at a particular point in the map evokes a response directed toward the corresponding point in space.
pretectum
The pretectal area, or pretectum, is a midbrain structure composed of seven nuclei and comprises part of the subcortical visual system. Through reciprocal bilateral projections from the retina, it is involved primarily in mediating behavioral responses to acute changes in ambient light such as the pupillary light reflex, the optokinetic reflex, and temporary changes to the circadian rhythm.
superior colliculus
The superior colliculus is a set of two bumps on the dorsal side of the midbrain. A larger region, the optic tectum, is formed by this structure and the inferior colliculus. Sometimes, the superior colliculus is referred to simply as the tectum. Unlike the inferior colliculus, which is involved in hearing, the tectum plays a role in processing vision.
This structure is involved with visual reflexes. Both the visual cortex and the retina of the eye itself project information to the outer layers of the tectum. Intermediate layers also receive sensory input from both visual and auditory neurons, as well as input from motor centers. The deepest layers receive mainly motor input, and can even direct eye movement and other motor actions. This wide variety of input types helps this structure to move the head and eyes toward sensory stimuli.
In each layer, neurons of the superior colliculus are arranged in a map. This representational map is aligned with retinal cells. Functionally, this allows activation of different retinal cells, triggering a corresponding response on the map. The tectum can then orient the eyes and head in the same direction the stimuli appeared.
Thalamocortical radiations
The optic radiation (also known as the geniculo-calcarine tract or as the geniculostriate pathway or recently posterior thalamic radiation) is a collection of axons from relay neurons in the lateral geniculate nucleus of the thalamus carrying visual information through two divisions (called Upper and Lower division) to the visual cortex (also called striate cortex) along the calcarine fissure.
Striate or visual cortex
The visual cortex of the brain is the part of the cerebral cortex responsible for processing visual information. It is located in the occipital lobe, in the back of the brain.
All visual information that the human mind receives is processed by a part of the brain known as visual cortex. The visual cortex is part of the outermost layer of the brain, the cortex, and is located at the dorsal pole of the occipital lobe; more simply put, at the lower rear of the brain. The visual cortex obtains its information via projections that extend all the way through the brain from the eyeballs. The projections first pass through a stopover point in the middle of the brain, an almond-like lump known as the Lateral Geniculate Nucleus, or LGN. From there they are projected to the visual cortex for processing.

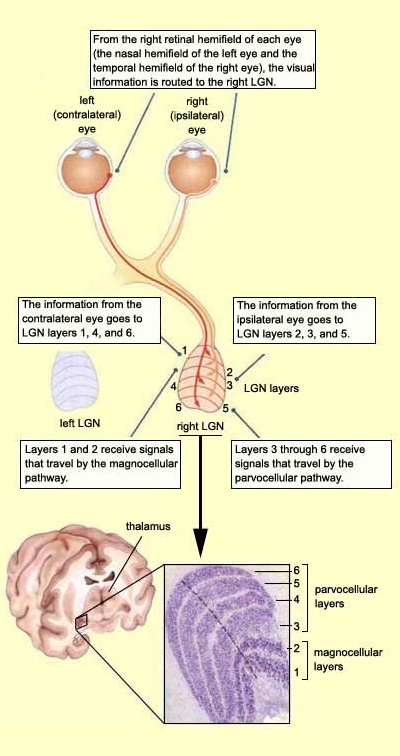
The Purpose of Our Eyes' Strange Wiring Is Unveiled
http://www.scientificamerican.com/article/the-purpose-of-our-eyes-strange-wiring-is-unveiled/
These results mean that the retina of the eye has been optimised so that the sizes and densities of glial cells match the colours to which the eye is sensitive (which is in itself an optimisation process suited to our needs). This optimisation is such that colour vision during the day is enhanced, while night-time vision suffers very little. The effect also works best when the pupils are contracted at high illumination, further adding to the clarity of our colour vision.
Retinal Glial Cells Enhance Human Vision Acuity
http://journals.aps.org/prl/abstract/10.1103/PhysRevLett.104.158102
AbstractWe construct a light-guiding model of the retina outside the fovea, in which an array of glial (Muller) cells permeates the depth of the retina down to the photoreceptors. Based on measured refractive indices, we propagate light to obtain a significant increase of the intensity at the photoreceptors. For pupils up to 6 mm width, the coupling between neighboring cells is only a few percent. Low cross talk over the whole visible spectrum also explains the insensitivity to chromatic aberrations of the eye. The retina is revealed as an optimal structure designed for improving the sharpness of images.
Mystery of the reverse-wired eyeball solved
http://medicalxpress.com/news/2015-02-mystery-reverse-wired-eyeball.html
From a practical standpoint, the wiring of the human eye doesn't make a lot of sense. In vertebrates, photoreceptors are located behind the neurons in the back of the eye - resulting in light scattering by the nervous fibers and blurring of our vision. Recently, researchers at the Technion - Israel Institute of Technology have confirmed the biological purpose for this seemingly counterintuitive setup. "The retina is not just the simple detector and neural image processor, as believed until today," said Erez Ribak, a professor at the Technion - Israel Institute of Technology. "Its optical structure is optimized for our vision purposes." Ribak and his co-authors will describe their work during the 2015 American Physical Society March Meeting, on Thursday, March 5 in San Antonio, Texas. Ribak's interest in the optical structure of the retina stems from his previous work applying astrophysics and astronomy techniques to improve the ability of scientists and ophthalmologists to view the retina at high detail. Previous experiments with mice had suggested that Müller glia cells, a type of metabolic cell that crosses the retina, play an essential role in guiding and focusing light scattered throughout the retina. To test this, Ribak and his colleagues ran computer simulations and in-vitro experiments in a mouse model to determine whether colors would be concentrated in these metabolic cells. They then used confocal microscopy to produce three-dimensional views of the retinal tissue, and found that the cells were indeed concentrating light into the photoreceptors."For the first time, we've explained why the retina is built backwards, with the neurons in front of the photoreceptors, rather than behind them," Ribak said. Future research for Ribak and his colleagues includes using water-filled goggles to reduce corneal aberrations, allowing observers to gain a finer view of the retina at depth.
Alleged dysteleology of the human eye—the ‘backwards’—vascularized retina
http://creation.com/evolutions-witness-how-eyes-evolved-review
The blind spot, which poses no problems whatsoever for human vision.
One common argument against an Intelligent Designer is the one about the ‘mistake’ of the retinal blood vessels situated above the surface of the retina, ‘obstructing’ the incoming light, as well as the existence of the blind spot. This argument, even on its own terms, has no merit.
Although Schwab does not address this issue in terms of ‘bad’ design, he does provide extensive detail on the vascularization of retinas in the Animal Kingdom and includes a very helpful diagram (p. 250) for comparing and contrasting the different forms of vascularization. The fact that so many different designs of vascular systems exist in the Animal Kingdom demonstrates, if nothing else, that there is no ‘right’ or ‘wrong’ way to construct such a system. Consequently, theological issues aside, it is meaningless to begin even to speak of the vascular system, in the human eye, as a form of bad design. Finally, as elaborated in the next section, whatever the anatomical and physiological limitations of the human eye, they are compensated by the extensive interpretive powers of the human brain.
If a change in selective pressures favored a dimpled eyespot with a slight increase in visual acuity, pretty soon the majority of the population would have dimpled eyespots. The problem with this notion is that no population of creatures with flat eyespots shows any sort of intra-population range like this were even a small portion of the population has dimpled eyespots to any selectable degree. This is a common assertion, but it just isn't true.
Now, if these 1,829 gradations really evolutionary steps that are in fact small enough to cross in fairly short order (a few generations each under selective conditions), it seems quite likely that such ranges in morphologic expression would be seen within a single gene pool of a single species. But, they aren't. Species that have simple flat light-sensitive eyespots only have flat light-sensitive eyespots. No individual within that species shows any sort of dimpled eye that would have any selective advantage with regard to increased visual acuity. This fact alone suggests that these seemingly small steps probably aren't that simple when it comes to the coordinated underlying genetic changes that would be needed to get from one step to the next.
A big problem with these morphologic steps is that they do not take into consideration the fact that vision is more involved than what goes on just within the eye. In order to take any advantage of improved visual acuity within the eye, the brain must also change in such a way that it is able to interpret the information the eye is sending it. Otherwise, if the brain is still step up to appreciate only differences in light from dark sent from the eye, without being able to interpret specific patterns of light and dark on the retina, there would be no selective advantage from a dimpled vs. a flat eyespot. Because of this requirement, whatever evolution happens to take place in the eye, must be backed up by equivalent evolution in brain development and interpretive powers.
Another interesting problem with the argument for a selective advantage for a dimpled eye over a flat eyespot is the fact that determining the general direction of a light source can be achieved with a flat eyespot. Dimpling is not needed to determine the relative direction from which a beam of light is coming. All that is needed is an ability to rotate the eyespot relative to the source of light combined with the brain's ability to associate differences in the intensity of light with the change in orientation of the eyespot relative to the source of light. This sort of associative ability could produce essentially the same effect of being able to localize and even follow or move toward a source of light without the need for producing a dimpled or cup-shaped eye. In fact, the species Euglena, with just a flat patch of light-sensitive cells, can swim toward a source of light - - no dimpling needed ( Link ). In fact, some creatures, like starfish and sea urchin have no eyespots at all yet are still sensitive to light to the degree that they can move toward sources of greater light intensity
‘How eyes developed’ is a sweeping generalization. Exactly how the genetic mechanism creates and incorporates neural systems to conduct visual data to various parts of the brain in a coordinated fashion is glossed over, and ignored completely.
The eye is not just a camera. Our vision field is a compilation of multiple retinal layers, dedicated to separate registration of motion in various direction and velocities, and also integrated into memory systems, as well as voluntary and autonomic muscular responses. Ultra-complex hierarchies do not appear as a result of random actions.
Had Darwin been aware of the enormous complexity of only the eye, and its relation to the brain, it would have been a formidable impediment to the generation of his general theory.
There is no such thing as a ‘naturalistic ‘need’ to create an eyes in the first place, and to further complexify its function.
1) http://evillusion.wordpress.com/sight-and-sound-a-daunting-task-for-evolution/
2) http://www.reviewevolution.com/press/pressRelease_EyeEvolution.php
3) http://www.grisda.org/origins/21039.htm
4) http://www.detectingdesign.com/humaneye.html
5) http://www.detectingdesign.com/humaneye.html
6) http://www.sicb.org/meetings/2016/schedule/abstractdetails.php?id=1275
Last edited by Admin on Sun Dec 30, 2018 8:38 am; edited 4 times in total