https://reasonandscience.catsboard.com/t2579-the-tongue-evidence-of-intelligent-design
https://evolutionnews.org/2022/09/a-closer-look-at-hummingbird-tongue-design/
Some interesting facts about taste and tongues:
Of all the types of microbes living in your mouth, bacteria are the most numerous. It has been estimated that there are over 100 million in every millilitre of saliva from more than 600 different species. The mouth is one the more dangerous places in the human body in terms of what comes out, what goes in, and what lives there. Fungi, micro-organisms, and viruses constantly come in with every bite of food and frequently just from breathing. In spite of all that, the tongue rarely gets infected, and when it does, it usually heals quickly. One reason is that the tongue secretes an antibiotic called “lingual antimicrobial peptide.” Studies done at the Magainin Research Institute show that the tongue secretes more antibiotic when there is a sore or injury. Our bodies are designed to survive in a world that has all kinds of things that could do us harm. The tongue is only one small, but important example of how design protects us from the dangers around us.
The woodpecker’s tongue is even more amazing than the headache-proof crash helmet it wears. Darwinists call it an “adaptation” which means it just happened that way because woodpeckers wanted to eat beetle larvae way inside trees–and were willing to wait a million years or so for their tongues to grow long enough to reach them. They say what proves it is an adaptation is that woodpecker tongues are just like other bird tongues, only longer (at least three to five times the length of its beak in some species)–as if two objects that are similar in some way have to be descended from a common ancestor rather than having been molded by a common To get a meal, the woodpecker buttresses its tail against a tree trunk, forming a tripod with its toes (two in front, two in back) and listens. Once it has determined that beetles are scuttling around inside and has drilled a hole with its beak, the base of its tongue disengages from under the jaw and re-attaches between its eyes as it plays out. The tongue is sensitive enough to feel the softness of a larval insect, yet stiff and sharp enough to pierce it (other studies indicate it is flexible and actually wraps around its prey) and sticky, with reverse barbs to keep the grub on the tongue as it retracts.
Hummingbird tongues are elastic micropumps
Pumping is a vital natural process, imitated by humans for thousands of years. We demonstrate that a hitherto undocumented mechanism of fluid transport pumps nectar onto the hummingbird tongue. Hummingbird tongues pick up a liquid, calorie-dense food that cannot be grasped, a physical challenge that has long inspired the study of nectar-transport mechanics. Existing biophysical models predict optimal hummingbird foraging on the basis of equations that assume that fluid rises through the tongue in the same way as through capillary tubes. We demonstrate that the hummingbird tongue does not function like a pair of tiny, static tubes drawing up floral nectar via capillary action. Instead, we show that the tongue tip is a dynamic liquid-trapping device that changes configuration and shape dramatically as it moves in and out of fluids. We also show that the tongue–fluid interactions are identical in both living and dead birds, demonstrating that this mechanism is a function of the tongue structure itself, and therefore highly efficient because no energy expenditure by the bird is required to drive the opening and closing of the trap. Our results rule out previous conclusions from capillarity-based models of nectar feeding and highlight the necessity of developing a new biophysical model for nectar intake in hummingbirds. Our findings have ramifications for the study of feeding mechanics in other nectarivorous birds, and for the understanding of the evolution of nectarivory in general. We propose a conceptual mechanical explanation for this unique fluid-trapping capacity, with far-reaching practical applications (e.g., biomimetics).
Blue whale tongues weigh almost 6,000 pounds. That's as much as African forest elephants weigh! They probably use their giant tongues to pick some of the thousands of pounds of krill out of their toothbrush bristle-looking baleen plates. Some snakes use folds of skin in their mouths to soak up water like a sponge through a mechanism called capillary action, a 2012 study found. Pangolins, a relative of anteaters, have long, sticky tongues supplied by an overactive salivary gland that they use to eat thousands of ants. The mammals are hunted in parts of Asia for their meat and scales, and are critically endangered. The okapi, which is related to giraffes and looks kind of like a zebra, has a tongue so long it can lick its own eyes and ears. The animal uses its lengthy tongue to strip leaves off of trees and into its mouth. Dogs make little cups out of their tongues when they drink water, and they are much sloppier than cats. Cat tongues make cups, too. Felines create a column of water under their tongue when they elegantly place it on the surface of the liquid. Mesmerizing, isn't it? Mallee fowl don’t sit on their eggs, like most birds do to let their body heat incubate the eggs. They build a large mound and monitor its temperature with their bill and tongue. The hen usually begins laying in late September — the southern hemisphere spring. From that time until about April, the male uses his beak and tongue to ensure that the temperature of the mound stays constant.
Chameleon Tongues
The chameleon also has one of the fastest tongues on the planet. It is so fast, in fact, that it is difficult for the human eye to see it when the lizard shoots it at potential prey (which may be more than a body length away). Just how fast is this super-hero-like tongue? Scientists have discovered that the chameleon’s tongue can accelerate five times faster than a fighter jet. Now that is fast! The design we see in nature is powerful evidence for the existence of God. Indeed, it is so powerful that it forced world-renowned atheist Antony Flew to admit his lifetime of scholarship was wrong and that God must exist. The more we learn about this planet and the life on it, the more we stand in awe of that amazing design. The chameleon is an excellent example of this trend. For a long time, scientists have known about the amazing design features of the chameleon. The more we learn, however, the more amazing chameleons become! Chameleons’ long, elastic tongues are one of the fastest muscles in the animal kingdom, extending more than twice their body length and packing 14,000 watts of power per kilo. But it is the smallest species that strike fastest, according to a new study. Researchers filmed tongue strikes of chameleons attacking a suspended cricket, at 3000 frames per second. They found that the animals’ tongues are capable of impressive acceleration, doing 0 kilometers to 100 kilometers per hour in one-hundredth of a second, twice as fast as the fastest car. And, like sports cars, the smallest chameleons are the most powerful. Across 20 species, ranging from the tiny 1-cm rosette-nosed chameleon (Rhampholeon spinosus) to the half-meter Malagasy giant chameleon (Furcifer oustaleti), the team found that smaller species’ tongues could accelerate at more than 250 g, five times faster than that of the largest chameleon. This is because small chameleons have evolved larger tongues relative to their body size, handy since they also need to consume proportionally more food to survive. As well as raw muscle power, chameleons spring-load the elastic tissue in their tongue, catapulting it toward prey when they strike, and giving them the highest acceleration and power output of any reptile, bird, or mammal. Previous studies underestimated their power because they failed to consider the little guy.
Salamander Tongue Is World's Most Explosive Muscle
The greatest burst of power from any animal muscle comes from the tongue of a tropical salamander, scientists have announced. The giant palm salamander of Central America (Bolitoglossa dofleini) captures fast-moving bugs with an explosive tongue thrust that releases over 18,000 watts of power per kilogram of muscle. 8 The salamander's ballistic firing permits the tongue's sticky-padded tip to reach prey in just a few thousandths of a second. Deban noted that the greatest power output from muscle acting alone has been measured in quail as they flap their wings in vertical takeoff.
The sticky, elastic tongues of amphibians have fascinated researchers for decades—the first study of frog and toad tongues was done in 1849. However, the underlying physics of this adhesive feat remained unclear. Previous studies compared frog tongues to scotch tape, but that’s not the full picture, researchers report today in the Journal of the Royal Society Interface. Yes, frog tongues are uniquely sticky (and their saliva makeup is crucial in bug capture), but they’re also very soft—10 times softer than human tongues and one of the softest known biological materials. That softness makes frog tongues more like adhesive shock absorbers than scotch tape, the researchers suggest. Here’s what happens: Say a bug is buzzing by and a frog releases its tongue. When the bug hits the frog’s tongue, the tongue wraps around the bug like a sticky bubble gum blanket and absorbs the bug’s inertia. Upon impact, the bug gets coated in saliva. Because the tongue is so soft, it can stretch out more than twice its thickness to cover more of the bug’s surface area and get its saliva into the grooves of a bug’s bumpy exoskeleton. Frog saliva is a non-Newtonian fluid, the team found, meaning it defies Newton’s law of viscosity and its flow changes under stress. So the saliva flows normally until the bug hits it, and then the saliva grips the bug as the tongue is retracted back into the frog’s mouth. But if a frog’s tongue is so sticky, how does it get its meal off its tongue and into its stomach? In a much studied phenomenon, frogs swallow with their eyes. The researchers found that when the eyes push against the bug in a motion parallel to the tongue, the saliva starts to flow easily again and down goes dinner. And although the researchers no longer think frog tongues are all that similar to scotch tape, this new finding might help them design new adhesives that stick at high speeds.
Flamingo Tongues Are Weirdly Phallic
There are a couple things that make flamingo tongues way weirder than average. First are the spiny bristles that coat their tongues, an adaptation that allows them to filter their food much like baleen whales do. On top of these unusual spines, scientists have discovered erectile tissue. These are a crucial part in how flamingo tongues work. When feeding, this tissue will fill with blood and become rigid. Since flamingos feed by dipping their heads upside down in water, researchers hypothesize this swollen tissue assists in the stabilization of their heads.
How vertebrates switched from feeding via suction to evolve a tongue remains unclear. 14
Taste perception: from the tongue to the testis 15
In mammals, the sense of taste helps in the evaluation and consumption of nutrients, and in avoiding toxic substances and indigestible materials. Distinct cell types expressing unique receptors detect each of the five basic tastes: salty, sour, bitter, sweet and umami. The latter three tastes are detected by two distinct families of G protein-coupled receptors: T2Rs and T1Rs. Interestingly, these taste receptors have been found in tissues other than the tongue, such as the digestive system, respiratory system, brain, testis and spermatozoa. The functional implications of taste receptors distributed throughout the body are unknown. We therefore reviewed the remarkable advances in our understanding of the molecular basis of taste perception in ‘taste’ and ‘non-taste’ tissues. We also present our speculations on the direction of further research in the field of male reproduction.
The anatomical substrates and units of taste detection are the taste receptor cells (TRCs), which are assembled into the taste buds distributed on the epithelium of the tongue and palate. Theoretically, the basic taste sensations are each recognized by different cells that express specialized receptors and distinct transduction pathways . Sour and salty tastes modulate the function of TRCs by the direct activation of specialized membrane channels. In contrast, sweet, bitter and umami taste transduction is mediated by a common G protein-coupled receptor (GPCR) signalling pathway.
Taste signal transduction
In general, the following molecular model has been suggested for signal transduction by taste receptors. When a tastant binds to T1Rs or T2Rs, taste GPCRs activate the heterotrimeric G protein α-gustducin. Ligand binding results in the release of the Gβγ13 subunits and the subsequent stimulation of phospholipase C-β2 (PLCβ2). Activation of PLCβ2 hydrolyses phosphatidylinositol-4,5-bisphosphate to produce two intracellular messengers, inositol-1,4,5-trisphosphate and diacylglycerol, and ultimately leads to calcium release from internal stores and activation of the taste transduction channel (the transient receptor potential protein, TRPM5;
The receptors and cells for mammalian taste 16

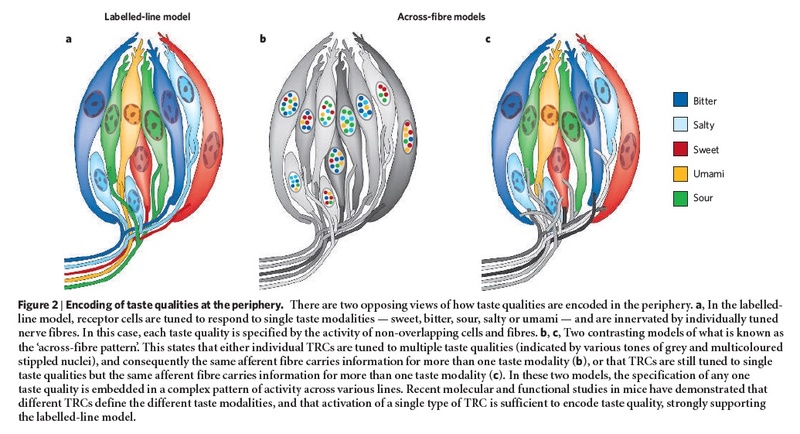
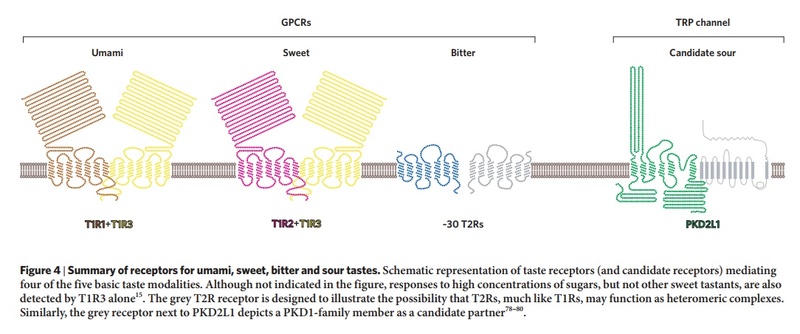
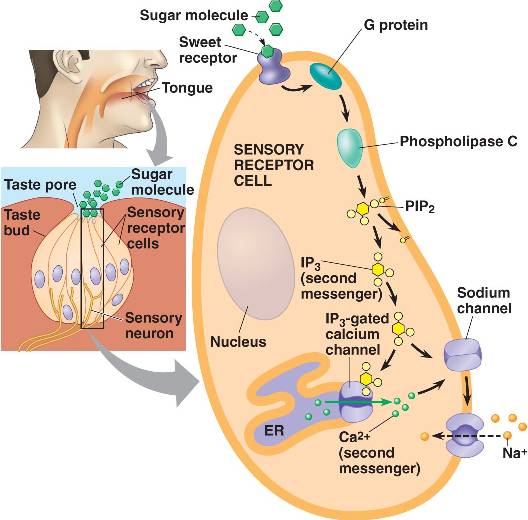
The receptor cells for taste are modified epithelial cells organized into taste buds, which are scattered on the tongue.
Binding of a sugar molecule to a receptor cell initiates a signal transduction pathway.
Sodium channels open, Na+ ions diffuse into the cell, and the membrane depolarizes.
Nerve signals are then sent to the parietal lobe of the cerebrum. 17
Mouth Bacteria 12
Of all the types of microbes living in your mouth, bacteria are the most numerous. It has been estimated that there are over 100 million in every millilitre of saliva from more than 600 different species.
Antibiotic Tongue 1
The mouth is one the more dangerous places in the human body in terms of what comes out, what goes in, and what lives there. Fungi, micro-organisms, and viruses constantly come in with every bite of food and frequently just from breathing. In spite of all that, the tongue rarely gets infected, and when it does, it usually heals quickly.
One reason is that the tongue secretes an antibiotic called “lingual antimicrobial peptide.” Studies done at the Magainin Research Institute show that the tongue secretes more antibiotic when there is a sore or injury.
Our bodies are designed to survive in a world that has all kinds of things that could do us harm. The tongue is only one small, but important example of how design protects us from the dangers around us.
1. The tongue is the only muscle in human body that works without any support from the skeleton 13
2. Our tongue is the home of our taste buds. When looked under a magnifying glass, hundreds and thousands of small bumps will become visible on the tongue. These bumps are known as papillae and are the actual home of our taste buds.
3. Tongue is not the only place where taste buds live. Taste buds can also be found on the inside of our cheeks, on lips, on the roof of our mouth and even under the tongue.
4. Approximately, there are 10,000 taste buds in our mouth of which 8,000 live on our tongue and the remaining 2,000 are found in the places we mentioned in the previous point.
5. There are specific segments on tongue for sensing different tastes. The notion that different parts of the tongue is responsible for sensing different types of tastes (in other words, there are taste belts) is actually a myth. Our tongue can taste sour, sweet, bitter, salty and umami. Umami is actually a very new variant of taste discovered by a Japanese scientist who found that the chemical that is responsible for this taste is monosodium glutamate.
6. Our tongue is the only muscle in our body that is capable of sensing taste and sending taste signals to the brain. Each individual taste bud has around 15 receptacles that are responsible for carrying taste signals to our brain.
7. The tongue is THE STRONGEST muscle in entire body. However, it is at the same time, one of THE MOST sensitive muscles as well.
8. In terms of flexibility, tongue beats every other muscle in our body! Because of this flexibility, the tongue is capable of easily manipulating food inside the mouth and is also capable of acting as a natural cleanser for our teeth after a meal.
9. Our tongue has a very unique property. It is incapable of detecting taste if it is dry. This means that if you place a piece of lemon on a dry tongue, you will not be able to tell that it is sour. The tongue gets its ability to sense taste only in the presence of saliva that keeps it moist.
10. The color of the tongue can tell a lot about a person’s health. Here are some color indications about health: Pink Tongue = Good Health; White Tongue = Fungal Infection and Yellow Tongue = Stomach Problem or Fever.
11. Tongueprints (actually tongue imprints) of humans are unique (very much same as the fingerprints). Tongues of different humans are of different shapes and will have different number of taste buds, thus making the tongue imprints unique.
12. Tongue has a really really rough texture. Did you ever notice that while kissing someone?
13. Women have shorter tongues compared to males.
14. We mentioned in point 9 that a dry tongue is incapable of detecting taste. That’s because taste buds are capable of sensing taste only when molecules of the food (or whatever you put in your mouth) dissolve in water (our saliva consists of water). This essentially means that you cannot sense taste of anything whose molecules do not dissolve in water even if you have a moist tongue. Ever tried tasting glass?
15. Here is an interesting tongue fact – you don’t keep your tongue clean and you will get bad breath. Why so? That’s because our mouth is the home of 600 different types of bacteria and a single saliva drop contains 1 million of those bacteria. Our entire tongue remains moist due to saliva. So, can you ever imagine the number of bacteria present on our tongue?
16. Every taste bud on our tongue has somewhere between 50 and 100 taste sensing cells. No individual cell is capable of tasting more than one taste.
17. About 2/3rd of the tongue is visible and the remaining 1/3rd is not visible. The part that is not visible is close to the throat.
18. In Tibet, you can merrily stick your tongue out at others. It will not be considered rude or childish. In Tibet, it is actually a greeting.
19. The tongue is more important than we think. It does not only help to taste food but also helps to talk, to spit, to swallow and even to kiss.
20. The longest human tongue to be ever recorded was 3.86 inches from back to tip. The widest tongue measured 3.1 inches. The longest female tongue to be ever recorded was 2.76 inches.
10
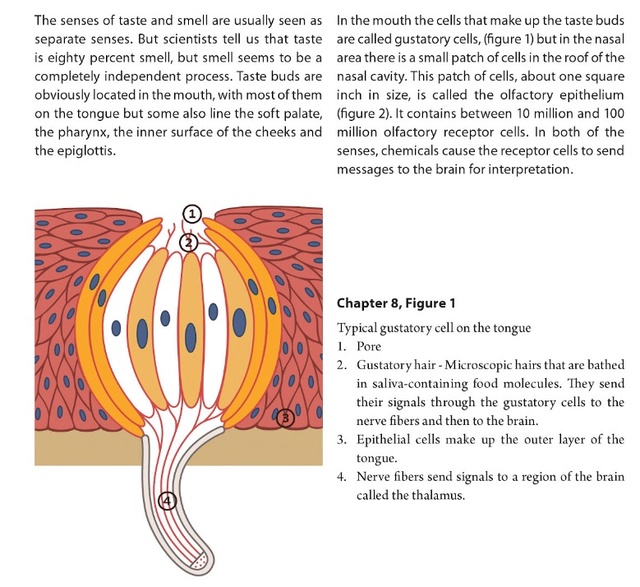


Woodpecker tongue 2
The woodpecker’s tongue is even more amazing than the headache-proof crash helmet it wears.
Darwinists call it an “adaptation” which means it just happened that way because woodpeckers wanted to eat beetle larvae way inside trees–and were willing to wait a million years or so for their tongues to grow long enough to reach them. They say what proves it is an adaptation is that woodpecker tongues are just like other bird tongues, only longer (at least three to five times the length of its beak in some species)–as if two objects that are similar in some way have to be descended from a common ancestor rather than having been molded by a common Artist. Actually, unlike other birds, the tongue of a woodpecker is not attached to the rear of its mouth. And, unlike any other tongues, it apparently doesn’t stay attached in one place. To get a meal, the woodpecker buttresses its tail against a tree trunk, forming a tripod with its toes (two in front, two in back) and listens. Once it has determined that beetles are scuttling around inside and has drilled a hole with its beak, the base of its tongue disengages from under the jaw and re-attaches between its eyes as it plays out.
The tongue is sensitive enough to feel the softness of a larval insect, yet stiff and sharp enough to pierce it (other studies indicate it is flexible and actually wraps around its prey) and sticky, with reverse barbs to keep the grub on the tongue as it retracts.
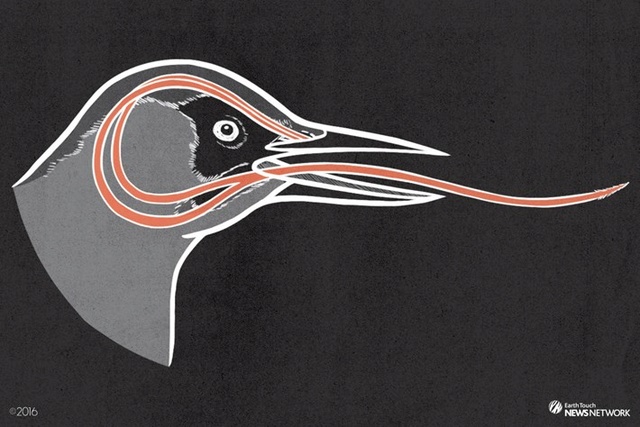
There’s the problem. You’re a woodpecker and you have a tongue three to five times as long as your beak. When you retract it, where does it go? Where can you store it? Can you wrap it around your brain or something? As a matter of fact, yes. When not in use, the European Green Woodpecker’s tongue goes “. . . around the back of the skull beneath the skin, and over the top between the eyes, terminating usually just below the eye socket.” Lane P. Lester and Raymond G. Bohlin, The Natural Limits to Biological Change (Grand Rapids: Zondervan, 1984), p. 25, 3
“In the case of the Red-bellied Woodpecker. . . the tongue forks in the throat, goes below the base of the jaw, and wraps behind and over the top of the head, where the forks rejoin and insert in the bird’s right nostril or around the eye socket.” 4
(Scientifically, “when the woodpecker wants to stick out its tongue, it contracts branchiomandibularis muscles near the base of the hyoid apparatus. This forces the hyoid bones forward within their sheath and propels the tongue out of the bill. Relaxing the muscles allows the tongue to shorten and brings it back inside.” Ibid.)
I would have loved to watch Blind Chance sittin’ there on the ground fumbling with woodpecker tongues (and butterfly wings and weeds and clam shells, whatever, since it can’t tell one from another), dropping one skull and picking up another and poking some things at other things, starting over with every single attempt (because it is too dumb to remember what it did last time and too dumb to know whether it worked or not so it can improve on it), suddenly coming up with something so creative even the biologists have to keep cautioning each other to “constantly keep in mind that what they see was not designed” (Francis Crick, Ph.D.). When Thomas Edison tried to find a filament that would work in a light bulb, he had the advantage of a brain and opposable thumbs and eyesight–not to mention foresight. It took him from 3,000-10,000 tries, depending on who you read. Can you imagine how many tries it took for Natural Selection to cobble together something so intricate and complicated? With maybe a fortunate mutation and a dab of genetic drift along the way, one random stab after another after another after another after another after another. . .
For years scientists have been trying to find out how the woodpecker avoids damaging its brain up against its skull as it slams its head into a tree at up to 20 ft/sec. 5
Unlike other animals like bighorn rams that butt their heads together, the woodpecker does not have a double bone in the front of the skull that acts like a shock absorber. They only have a single layer of bone. This has long intrigued scientists, so a team from the Hong Kong Polytechnic University led by Ming Shang set out to discover the woodpecker’s secrets.
They filmed a woodpecker in slow motion striking against a pressure plate to capture the details of the impact. Upon careful examination of the slow motion footage, they discovered that the woodpecker turns its head just enough to lessen the effects of a direct impact. In addition to the slow motion studies, they also used computer thermal tomography of the skulls of woodpeckers. This study revealed that there is a slight difference in the length of the upper and lower beaks. The amount of force created at the tip of the beak is greater than the force that is transmitted to the bones of the skull. It was determined that the difference in beak length served to reduce the force of impact.
They also used a scanning electron microscope to examine the bones of the skull. Under the details of the microscope it was revealed that the bones in the front of the skull have what they described as a ‘spongy’ structure in key locations. These spongy pockets are strategically placed so as to absorb some of the shock force.
The brain of the woodpecker is also shaped in such a way as to minimize the possibility of brain damage. Instead of being longer front to back like the human brain, the woodpecker’s brain is longer up and down, thus reducing the impact surface and subsequent shock force.
If you have ever watched any kind of crime show or forensic program that involved someone being strangled, you probably heard reference to the hyoid bone. In humans, this is a small horseshoe shaped bone that lies just over the Adam’s apple. It is not connected to any other bone and is fairly fragile. The hyoid is so thin that it is easily broken when someone is manually strangled. In the case of the woodpecker, the hyoid bone is much different. According to the report I read the woodpecker’s hyoid acts like a safety belt and was described as: Starting at the underside of the birds’ beaks, it makes a full loop through their nostrils, under and around the back of their skulls, over the top and meeting again before the forehead. Other scientists are now looking at the design features of the woodpecker’s skull to see if it will help them create more efficient safety helmets for sports, and for bicyclists and motorcyclists. I always have to smile when scientists spend so much effort and intelligence trying to duplicate what they believe happened by chance in nature.

What I found confusing in the report that I read was they attributed the woodpecker’s special features to only three things: the hyoid bone, the difference in length between upper and lower beaks and the spongy structures of the bones of the skull. However, when as I read their report, I counted five design features not three. To their list you have to add the way the woodpecker turns its head at impact and the shape of the brain. When you add all of these features together, you get an extremely complex set of design features that all have to be in place in order for the woodpecker to avoid turning its brain into mush as it pecks against tree after tree. If they evolved one feature at a time, the woodpecker would never have survived long enough to pass its traits on to subsequent generations. When talking about the special design features of the woodpecker’s skull, you also have to consider their tongue and how it sets in their head. The woodpecker has a very long sticky tongue that is far too long to fit in its bill. They use these long sticky tongues to pull insects out of the holes they drill into the trees. So if the tongues are too long to fit into the bill, where does it go? The tongue is pulled into a muscular sheath that extends under the scalp, around the head and then back into the right nostril. I have yet to see an evolutionary explanation for this weird feature. Add the special design features listed above to the weird design of the tongue and you have far too many unique features that all needed to be in place for the woodpecker to survive. So the next time you see or hear a woodpecker hammering away at a nearby tree, take the opportunity to share its unique designs with those with you. Explain to them that the only possible explanation for the woodpecker is our all-knowing Creator God. 6
The Hummingbird Tongue 7
Hummingbird tongues pick up a liquid, calorie-dense food that cannot be grasped, a physical challenge that has long inspired the study of nectar-transport mechanics. Existing biophysical models predict optimal hummingbird foraging on the basis of equations that assume that fluid rises through the tongue in the same way as through capillary tubes. We demonstrate that the hummingbird tongue does not function like a pair of tiny, static tubes drawing up floral nectar via capillary action. Instead, we show that the tongue tip is a dynamic liquid-trapping device that changes configuration and shape dramatically as it moves in and out of fluids. We also show that the tongue–fluid interactions are identical in both living and dead birds, demonstrating that this mechanism is a function of the tongue structure itself, and therefore highly efficient because no energy expenditure by the bird is required to drive the opening and closing of the trap. Our results rule out previous conclusions from capillarity-based models of nectar feeding and highlight the necessity of developing a new biophysical model for nectar intake in hummingbirds. Our findings have ramifications for the study of feeding mechanics in other nectarivorous birds, and for the understanding of the evolution of nectarivory in general. We propose a conceptual mechanical explanation for this unique fluid-trapping capacity, with far-reaching practical applications (e.g., biomimetics).
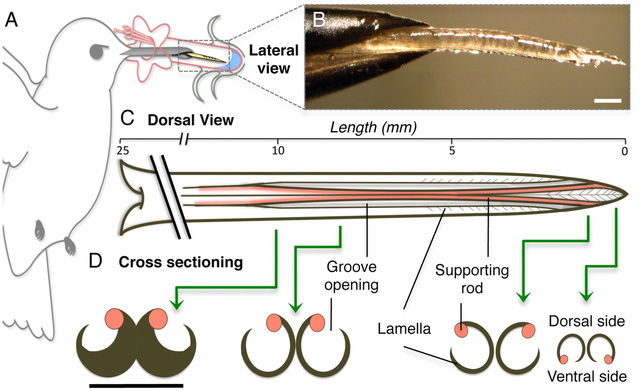
Fig. 1.
Hummingbird tongues. (A) Nectarivores use their tongue (yellow) as their primary food-gathering tool. (B) Lateral picture of a post mortem Ruby-throated Hummingbird (Archilochus colubris) tongue tip protruding from the bill tip. (C) Dorsal view of the morphology of a hummingbird tongue (approximate dimensions for A. colubris) showing length of the entire tongue, open-sided grooves, and the fringed (lamellar) region of the tip (distal approximately 6 mm). Base of the tongue is on the left; tip on the right. (D) Cross-sectioning shows the structural arrangement along the distal region of the tongue; green arrows identify the placement of the cross-sections. Black lines indicate the same structures in dorsal and cross-sectional views. Note the change in position of supporting rods from the base of the grooves to the tongue tip. Unlabeled scale bars, 0.5 mm.
https://www.youtube.com/watch?v=dMw3RO7p9yg
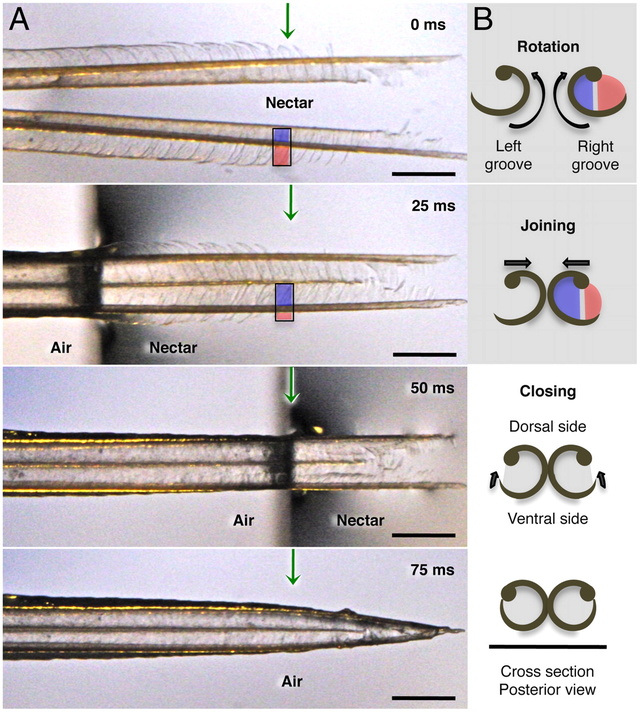
Fig. 2.
Hummingbird tongue trapping nectar. (A) Dorsal view of a post mortem tongue tip (A. colubris) leaving nectar, from totally immersed (Top photograph) at 0 milliseconds (ms), to outside the liquid (Bottom photograph). Green arrows mark the same reference point on the tongue in each image. (B) Cross-sectional diagrams (right margin) indicate the changes in position of lamellae at the reference point over time. From top to bottom: inside rotation of the entire structure (blue and red colors represent portions of visible lamellae along each side of the rod), tongue tips joining, and lamellae closing. In the first two diagrams, lamellae are inside the nectar; in the last two, lamellae have been withdrawn but contain nectar trapped inside the grooves. Scale bars, 0.5 mm.
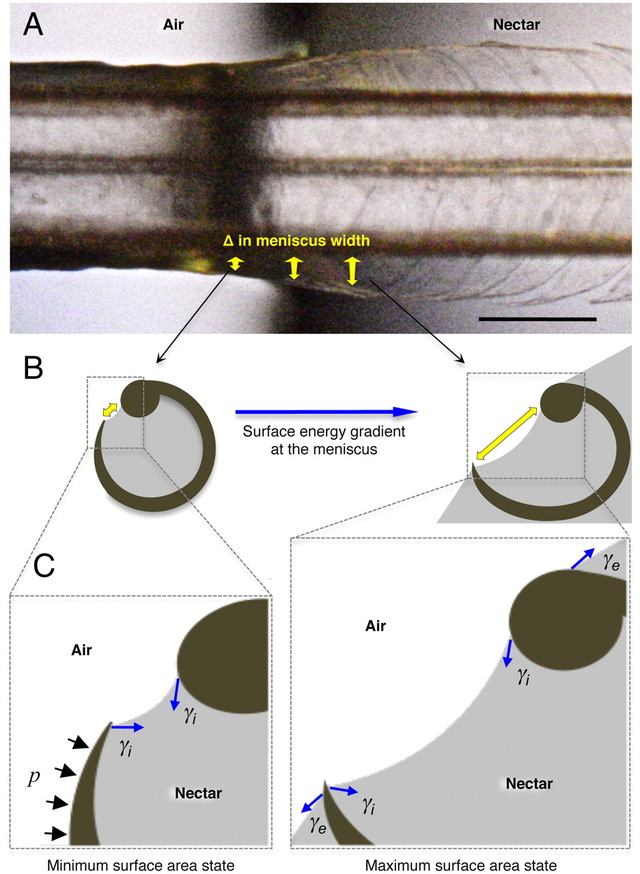
Fig. 3.
Conceptual hypothesis of the forces involved in lamellar closing. Blue arrows indicate the force exerted by surface tension (γ). Black arrows represent the Laplace pressure (p). (A) Dorsal view of a post mortem tongue (A. colubris) interacting with the air–nectar interface, showing the change in lamellar position with respect to the change in meniscal width (sagittally inclined yellow arrows). (B) Cross-section diagrams indicating the surface energy gradient on the internal menisci outside the nectar (Left) and at the beginning of the interface (Right). Yellow arrows depict meniscal width matching the points in the Upper panel. (C) Conceptual representation of the main forces acting on each lamella. Note that the minimum surface area state is achieved outside the nectar (Left) and the maximum surface area state is reached at the beginning of the interface (Right). When the tongue is leaving the nectar and the fluid no longer covers the outer wall of the lamella, the external component of the surface tension (γe) stops operating on the structure, Laplace pressure (p) begins to act and the surface area tends to be reduced by the internal component of surface tension (γi). The net result is the bending of the flexible lamella over the stiffer rod. Scale bar, 0.5 mm.
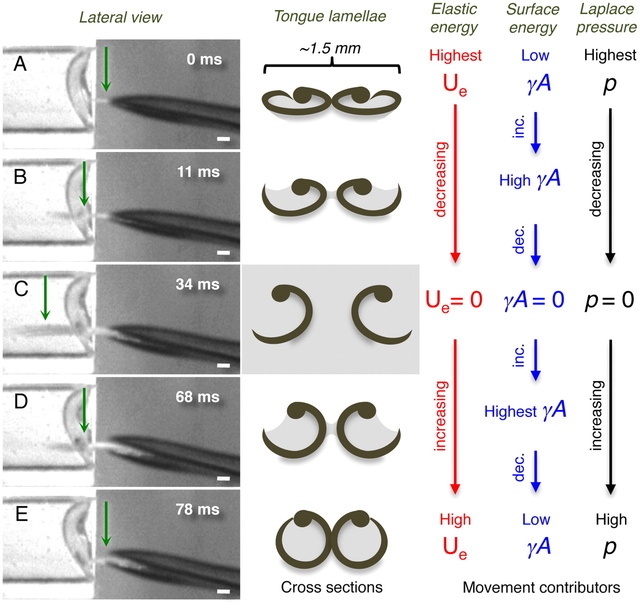
Fig. 4.
Conceptual hypothesis for lamellar movements during the licking (tongue) cycle. (Left column) Frames from the high-speed videos showing a lateral view of the bill tip and the tongue of a living Indigo-capped Hummingbird (Amazilia cyanifrons). Green arrows identify the cross-sections denoted in the middle column. (Center column) Cross-sections of the tongue tip showing the shape of the lamellae on each frame in the Left column. (Right columns) Conceptual depiction of the hypothesized relative contributions of the most important contributors to lamellar movements on each shape of the lamellae. Red stands for elastic potential energy (Ue), blue for surface energy (γA), and black for Laplace pressure (p). (A) The cycle begins when the tongue is protruded through a narrow space left when the bill tips are separating from each other. (B) Tongue penetrating the nectar located in the artificial feeder on the Left. (C) Maximum protrusion distance of the tongue in this licking cycle. (D) Tongue leaving the fluid while being retracted inside the bill. (E) Tongue almost fully retracted inside the bill; when the bill closes the cycle starts again. Scale bars, 1 mm.
Hummingbird tongues are elastic micropumps 8
Pumping is a vital natural process, imitated by humans for thousands of years. We demonstrate that a hitherto undocumented mechanism of fluid transport pumps nectar onto the hummingbird tongue. Using high-speed cameras, we filmed the tongue–fluid interaction in 18 hummingbird species, from seven of the nine main hummingbird clades. During the offloading of the nectar inside the bill, hummingbirds compress their tongues upon extrusion; the compressed tongue remains flattened until it contacts the nectar. After contact with the nectar surface, the tongue reshapes filling entirely with nectar; we did not observe the formation of menisci required for the operation of capillarity during this process. We show that the tongue works as an elastic micropump; fluid at the tip is driven into the tongue's grooves by forces resulting from re-expansion of a collapsed section. This work falsifies the long-standing idea that capillarity is an important force filling hummingbird tongue grooves during nectar feeding. The expansive filling mechanism we report in this paper recruits elastic recovery properties of the groove walls to load nectar into the tongue an order of magnitude faster than capillarity could. Such fast filling allows hummingbirds to extract nectar at higher rates than predicted by capillarity-based foraging models, in agreement with their fast licking rates.
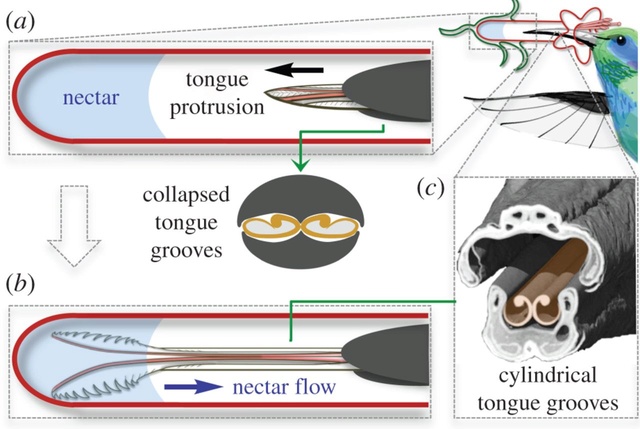
The hummingbird tongue fills with nectar even when only the tip is immersed. (a) Hummingbirds can drink from flowers with corollas longer than their bills by extending their bifurcated, longitudinally grooved tongues to reach the nectar. During protrusion, the tongue is compressed as it passes through the bill tip, which results in a collapsed configuration of the grooves (cross-section). (b) Upon reaching the nectar, the tongue tips fringed with lamellae roll open and spread apart, but some of the grooved portions of the tongue will never contact the nectar pool. For the grooves to fill with nectar, they must return to their uncompressed, cylindrical configuration. (c) Coronal cutaway from a µCT scan showing the bill and tongue architecture. Hummingbird drawn by K. Hurme.
The cylinders are in a flattened shape when they enter the nectar. Having been compressed by the beak, they store elastic energy that makes them rapidly expand in the fluid as they unfurl. This expansion helps to pump the fluid into the cylindrical cavity upward from the lamellae. That way, more nectar can be delivered into the bird’s mouth. 9
https://www.youtube.com/watch?v=QYoYQAbPXbU

Blue whale tongues weigh almost 6,000 pounds. That's as much as African forest elephants weigh! They probably use their giant tongues to pick some of the thousands of pounds of krill out of their toothbrush bristle-looking baleen plates.
Some snakes use folds of skin in their mouths to soak up water like a sponge through a mechanism called capillary action, a 2012 study found.
Pangolins, a relative of anteaters, have long, sticky tongues supplied by an overactive salivary gland that they use to eat thousands of ants. The mammals are hunted in parts of Asia for their meat and scales, and are critically endangered.
The okapi, which is related to giraffes and looks kind of like a zebra, has a tongue so long it can lick its own eyes and ears. The animal uses its lengthy tongue to strip leaves off of trees and into its mouth.
Dogs make little cups out of their tongues when they drink water, and they are much sloppier than cats.
https://www.youtube.com/watch?v=0hb7E7IjrFc
Cat tongues make cups, too. Felines create a column of water under their tongue when they elegantly place it on the surface of the liquid. Mesmerizing, isn't it?
Mallee fowl don’t sit on their eggs, like most birds do to let their body heat incubate the eggs. They build a large mound and monitor its temperature with their bill and tongue. The hen usually begins laying in late September — the southern hemisphere spring. From that time until about April, the male uses his beak and tongue to ensure that the temperature of the mound stays constant.
1. http://www.dandydesigns.org/id54.html
2. https://hiddeninjesus.wordpress.com/2012/10/21/intelligent-design-woodpecker-tongue/
3. http://www.present-truth.org/3-Nature/Evolution%20of%20Creationist/MOGC%2010.htm
4. http://www.hiltonpond.org/thisweek030308.html
5. http://creationrevolution.com/scientists-discover-design-features-of-woodpecker-pecking/#f7tW1TdAfzoOuBTo.99
6. http://www.bbc.co.uk/news/science-environment-15458633
7. https://evolutionnews.org/2015/09/hummingbird_ton/
8. http://rspb.royalsocietypublishing.org/content/282/1813/20151014
9. https://evolutionnews.org/2015/09/hummingbird_ton/
10. https://books.google.com.br/books?id=URxlAgAAQBAJ&pg=PA22&lpg=PA22&dq=tongue,+intelligent+design&source=bl&ots=vlKC_TMAG-&sig=cYc-8Pt_3XUbOukq7sgSp-ua_eM&hl=pt-BR&sa=X&ved=0ahUKEwi-_pu9__bUAhVKfiYKHW8GAzMQ6AEIUzAH#v=onepage&q&f=true
11. http://www.businessinsider.com/craziest-ways-to-drink-in-the-animal-kingdom-2015-10/#dogs-make-little-cups-out-of-their-tongues-when-they-drink-water-and-they-are-much-sloppier-than-cats-11
12. http://www.brighthub.com/science/genetics/articles/45935.aspx
13. http://factslegend.org/20-interesting-human-tongue-facts/
14. https://www.newscientist.com/article/dn27181-tongues-may-have-evolved-from-a-mouthful-of-water/
15. https://academic.oup.com/molehr/article/19/6/349/1061673/Taste-perception-from-the-tongue-to-the-testis
16. http://www.nature.com.sci-hub.cc/nature/journal/v444/n7117/full/nature05401.html
17. http://bio1152.nicerweb.com/Locked/media/ch50/taste.html
More readings:
The Craziest Tongues In Nature
http://www.ranker.com/list/crazy-animal-tongues/eric-vega
Last edited by Otangelo on Thu Sep 08, 2022 11:38 am; edited 18 times in total