Ontogeny, Phylogeny and the Origin of Biological Information 1
http://reasonandscience.heavenforum.org/t2308-origin-of-development-and-ontogeny#4757
1. IntroductionIn 1984 I presented the semi-meiotic hypothesis of organic evolution. This was followed by three other papers which exposed the
complete failure of virtually every aspect of the Darwinian model (Davison 1984 1987 1993 1998). Incorporating the conclusions of Otto Schindewolf (1896-1971), Richard B. Goldschmidt (1878-1958), William Bateson (1861-1926), Robert Broom (1866-1951), Pierre Grassé (1895-1985) and Leo S. Berg (1876-1950), the semi-meiotic hypothesis can be summarized as follows. Macroevolution is largely finished. Sexual reproduction is incapable of supporting trans-specific (macroevolutionary) change. Accordingly, all significant change was produced presexually involving the first meiotic division. The essential feature of these changes was due not to micromutations in the genes themselves, but rather to the way in which those genes express their effects which is dependent upon their arrangement within the structure of the chromosome (position effect).
So how did the right program arise to permit the cells to find their right position, and assigning the right kind of cells at the right place , and the right quantity, and replacing cells during development, and cells with no use to be destroid ? The first meiotic division is ideally suited to this form of reproduction as it can preserve the original karyotype and at the same time produce structural novelties instantaneously in homozygous form (Davison 1998). Thus, this model provides a rational explanation for the absence of intermediates both in contemporary and in fossil species.
Such a mechanism does not depend on the production of new information but rather on information already present in the genome i.e. preformed. This perspective remains in complete accord with the remarkable resemblances we find underlying disparate species, from the ubiquity of ATP to the universal 9 plus 2 nature of the structure of cilia and flagella wherever they are found in the living world. It should also be noted that Schindewolf (1993), Goldschmidt (1940), Berg (1969) and Grassé (1973) all subscribed to preadaptation (preformation) during evolutionary change.
These considerations bring me to the subject of this paper.
First, what is the nature and source of the preformed information? Second, how was it made manifest? In considering these two questions I came, as have others, to the realization that there is an intimate relationship between ontogeny and evolution (phylogeny).
The Russian ichthyologist and zoogeographer Leo S. Berg was perhaps the first to indicate that evolution and the development of the individual were closely related. In a clear demonstration of his rejection of Darwinism, he wrote:
The laws of the organic world are the same whether we are
dealing with the development of an individual (ontogeny) or
that of a paleontological series (phylogeny). Neither in
the one nor in the other is there room for chance.
Nomogenesis (1969), page 134
Note that Berg insisted that chance plays no role in either process. Whatever an organism becomes is determined entirely by the orderly unfolding of events that take place during its embryonic development. I accept Berg's judgment and elaborate on it to see where that perspective takes us with respect to our understanding of the two as
yet unresolved problems of ontogeny and phylogeny.2. Epigenesis and preformationThese two terms originated from observations on the nature of the developmental process. It is obvious that all of the information necessary to produce a unique human being is contained (preformed) in a single cell, the fertilized egg, a mere few microns in diameter. On the other hand,
the development of the individual is largely epigenetic. I will deal first with epigenesis and then with preformation. Epigenesis refers to the necessity for development to proceed in a definite serial fashion. For example,
neurulation or the formation of the central nervous system cannot proceed until gastrulation or the formation of the primitive digestive system is complete. Following that, the optic cup can be formed. Only after that can lens induction take place. These ontogenetic events have interesting phylogenetic (evolutionary) parallels.One of the most significant events in vertebrate evolution was the invention of bone. Since bone is significantly more dense than the cartilage it replaced, bony vertebrates would have been relegated to the bottom of the sea if it were not for the early development of the swim bladder, an outgrowth of the primitive gut. This hydrostatic organ made it possible for the animal to maintain neutral buoyancy. The swim bladder is homologous with the vertebrate lung which in turn made possible the invasion of the land and the evolution of the higher vertebrate taxa (amphibians, reptiles, birds and mammals). Accordingly,
the invention of bone and the swim bladder both served to preadapt subsequent vertebrates for life on land. It is significant that the Chondrichthyes (sharks skates, rays and chimeras) have remained cartilaginous and accordingly less specialized which may account for the ongoing successful presence of this very primitive group.
When something is lost during evolution it is rarely replaced. Examples are the loss of limbs in reptiles and amphibians, or digits in many tetrapod vertebrates. Returning to the swim bladder example, the darters, tiny members of the perch family of fishes, have lost the swim bladder, a loss which allowed them to successfully invade swiftly flowing freshwater streams. The Darwinian view would be that this was an adaptation to the stream environment. An alternative view would be that, having lost the swim bladder, the darters sought out or perhaps simply stumbled into the stream environment where they would have a clear advantage over buoyant competitors. Also the Darwinian interpretation would suggest that the swim bladder was gradually reduced, yet there is no evidence whatsoever for that assumption. It would seem that many evolutionary specializations
occur instantly without intermediate forms. Thus, just as ontogeny goes forward in epigenetic fashion, so has evolution. The two processes also share irreversibility as a cardinal feature.
It is when we come to preformation that things really become interesting. I now suggest that just as ontogeny and evolution share epigenetic features, so also may they share the characteristics we associate with preformation. In short,
I propose that the information for virtually all of evolution may have been present from very early in the onset of that process. I realize that this idea may seem ludicrous at first glance, yet it remains compatible with an enormous number of otherwise enigmatic observations from comparative biology. It also avoids postulating Lamarckian devices for which no evidence has been forthcoming.
Before presenting this material it is necessary to grapple with the question of the origin of the information itself. If, as I suggest, the information was preformed, someone or something had to put it
there. I am not the first to confront this problem. I quote Pierre Grassé:
According to Darwinian doctrine and Crick's central dogma, DNA
is not only the depository and distributor of the information
but its sole creator. I do not believe this to be true.
(Grassé's emphasis)
Evolution of Living Organisms
(1973), page 224
Oddly, Grassé does not pursue the problem further. I think we have an obligation to do so. It is my understanding that information does not arise de novo but must have a source. While that source has yet to be identified, its existence is suggested by a host of examples from both the plant and animal kingdoms.
Figure 1. Ris.65. Longitudinal section through the optical infusorian Diplodinium (Epidinium) ecaudatum. (According to Sharp, 1914). ecaudatum. (According to Sharp, 1914). 1 - It has a kind of "brain" (motorium)
; 2 - adoral cirri; 3 - mouth; 4 - conductive filaments of the pharynx; 5 - fibrils pharynx; 6 - skeletal plate; 7 - endoplasm; 8 - "hindgut"; 9 - poroshitsa; 10 - contractile vacuole; 11 - Ma;Mi-12; 13 - dorsal lip; 14 - dorsal cirri area.Works American Protistology dedicated to "neuromotor apparatus" ciliates were subjected to severe criticism by many researchers in a number of countries. Bretschneider (Bretschneider, 1934), detailThe unicellular Amoeba is structurally one of the simplest of the Protozoa, yet in that same phylum can be found creatures exhibiting strikingly advanced features. One such animal is Diplodinium (Epidinium) ecaudatum
(Figure 1). This ciliate exists in huge numbers as symbionts in the stomachs of cattle (Sharp 1914). It has a kind of "brain" (motorium), "circumoesophageal neural connectives" resembling those of annelids and arthropods, clearly differentiated "muscles", a kind of "segmented skeleton" (skeletal laminae), a "mouth", "esophagus", "rectum" and "anus" all elaborated within the confines of a single cell.
Such a creature not only demonstrates that the information is already present for these structures, but also casts considerable doubt on the notion that multicellularity is a prerequisite for the division of labor. It is no wonder that Libby Hyman described the protozoa as the acellular rather than the unicellular animals.
Why this creature should have such an array of advanced features remains a complete mystery. Could it be there to provide us with a clue to the nature of the evolutionary process?
The relict worm-like Peripatus with its curious combination of annelid and arthropod characters could not be placed in either phylum, so it has been placed in a phylum of its own, the Onycophora.
While we associate the placenta with the higher mammals, this structure also is found in certain sharks. Berg (1969) discussed this and related phenomena as examples of what he called physiological acceleration. He noted that Peripatus also nourishes its embryos with a kind of placenta. Thus
Peripatus exhibits characters of three taxa: the annelids, the arthropods and the placental mammals.The primitive chordate Amphioxus (Branchiostoma lanceolatus), while it has all three chordate features (dorsal hollow nervous system, gill slits and notochord), has a kidney consisting of solenocytes of the protonephridial (flame cell) type found in the Platyhelminthes, the Aschelminthes and the polychaete Annelida. On the other hand, certain oligochaete Annelida (earthworms) have a tubular kidney system more like that of vertebrates. The limbless amphibia (Apoda) have large yolk-laden eggs suspended by albuminous chalazae closely resembling the situation in the cleidoic (shelled) eggs of reptiles and birds. They lack only the shell. Such bizarre forms as the egg-laying monotreme mammals (Platypus and Echidna) can suddenly seem acceptable if one simply assumes that those combinations of characters were available when those forms evolved. When it comes to the possible
combinations of features one can almost say -- Anything goes!
While we associate imaginal discs with the complete metamorphosis of the higher insects (Diptera, Hymenoptera, Homoptera and Lepidoptera), imaginal discs are also involved in the metamorphosis of certain marine worms (Nemertea), with the formation of the adult or imago taking place within the body of the Pilidium larva which then splits open to release the adult form much as does the pupa of a moth or butterfly. Considerations like these and many others prompted Leo Berg (1969) to conclude: "Evolution is in a great measure an unfolding of preexisting rudiments."
The same view was independently offered by Pierre Grassé (1973): "The existence of internal factors affecting evolution has to be accepted by any objective mind."
The very word evolution derives from the Latin evolvo meaning to unfold as the pages of a book. Needless to say, a book has, by definition, already been written. The plant kingdom abounds with examples in support of preformation. Distantly related plant species often produce similar, if not identical, chemical scents of anise, lemon, orange, pineapple, cinnamon and a host of other flavorings so valuable to our cuisine. There is even a chocolate scented member of the mint family!
The behavioral and structural reciprocities that often exist between plants and their insect pollinators indicate that they may have been sharing the same informational pool when those evolutionary events took place.What does that mean ? What " informational pool " is the author writing about ? How about we put the intelligent designer as the source of that pool ? makes sense ? One of the most remarkable of such interactions was described by Berg (1969). Certain stick and leaf insects (Phasmodea) resemble the plants that they inhabit
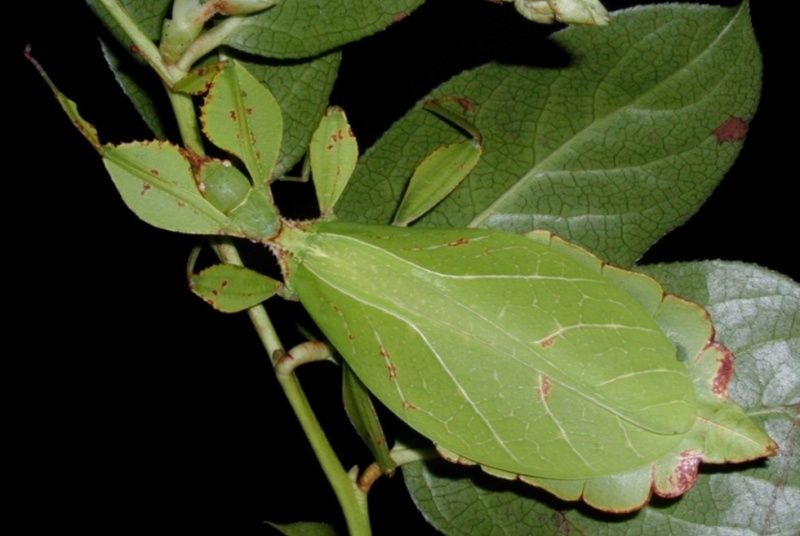
even to the extent that the eggs of the insects closely resemble both internally and externally the seeds of the plants (Umbelliferae) they inhabit and, like the seeds, are scattered on the ground where they may remain for up to two years. The tunic of the Urochordata (sea squirts) is composed of cellulose, otherwise a polymer associated with the plant kingdom.
Rather than assuming independent inventions of these remarkable parallels, it seems to me more reasonable to postulate these events resulted from the activation (derepression) of an enormous yet clearly limited stockpile of potentialities which were available when those events took place.
These considerations cast a whole new perspective on what has been called convergent evolution. The morphological similarities that exist, especially in the skeletal systems, between placental wolves and bears and their marsupial counterparts, to my mind, defy any explanation based on the accidental evolution of these similarities through chance events. Thus, what has been described as convergent evolution is not that at all but the expression from preformed sources of virtually identical morphologies. In this connection it is useful to recall the words of R.C. Punnett:
Natural selection is a real factor in connection with mimicry,
but its function is to conserve and render preponderant an
already existing likeness, not to build up that likeness
through the accumulation of small variations, as is so
generally assumed.
(my emphasis)
Mimicry in Butterflies
(1915), page 152
One is compelled to ask -- What is the source of the already existing likeness?
How such transformations were effected remains, of course, a complete mystery. Unfortunately, since macroevolution seems no longer to be in progress, we may never be able to resolve that issue.
However intangible, the issue remains -- What is the origin of the preexisting information?3. Has evolution been guided?Questions such as this can probably never be resolved. Nevertheless, one can still offer observations which relate to such metaphysical considerations.
Some of our greatest minds have been physicists. Galileo, Newton, Pascal, Faraday and, in particular, Einstein all have referred to God one way or another. Richard P. Feynman (1998) has compared scientific discovery to a religious experience. I think it is fair to say that we are still ignorant of the source of bright ideas, insights and acts of creative genius.
It is interesting to compare our interpretation of the laws that govern the inanimate world with those that may be operative in the animate world.
Leo Berg, as the complete title of his book indicates, believed that all of both evolution and development was the result of laws, a perspective with which I have (admittedly reluctantly) been forced to agree. If not chance, it seems to me that the only alternative is to agree with Berg. The existence of laws presumes a law maker or makers. That in turn suggests purpose. Don't representatives in Congress have some purpose in mind when they enact legislation?No one denies the validity of Galileo's equation which relates the distance that a body falls to time, or Newton's laws of motion, or Einstein's equation relating energy and mass. Why then must one reject, as the Darwinians do, the suggestion that comparable laws exist or have existed controlling the living world? Everyone accepts gravitation and the equations associated with it, yet no one yet understands the cause of gravity. Accordingly, neither in religion nor in science does acceptance demand understanding.
Nevertheless, the Darwinians continue to insist that evolution is the result only of chance events. Stephen J. Gould has recently compared evolution to a drunk reeling back and forth between the bar room wall and the gutter (Gould 1996 page 149). He has also described intelligence as an evolutionary accident. I will only comment that it was some accident!
Isn't it conceivable that there may really be no conflict between religion and evolutionary science? Isn't it possible that both might be correct? I have come to believe just that. Blaise Pascal offered the following commentary as well as the solution:
Men despise religion. They hate it and are afraid it may be true. The cure for this is first to show that religion is not contrary to reason, but worthy of reverence and respect.Finally, let me close with the following appropriate quotations from
my favorite intellectual, Albert Einstein:
I want to know God's thoughts ... the rest are details.
The most beautiful thing we can experience is the mysterious.
It is the source of all true Art and Science.
Science without religion is lame. Religion without science
is blind.
I shall never believe that God plays dice with the world.
I rest my case.
4. ConclusionThe greatest impediment to progress in the field of evolution resides in the stubborn refusal of the Darwinians to entertain any possibility that the living world might be subject to laws such as govern virtually every aspect of the inanimate world.
I find it fascinating that it is the physicists who postulate God while biologists typically remain atheists or agnostics. I offer the following explanation for that curious situation.
It is the physicists who have been able to discover the several laws that serve to describe and thereby explain their domain of the inanimate world. Thus they have been forced to come to grips with the reality that laws must have an origin. By way of contrast, most biological scientists have yet to acknowledge those laws that so clearly operate in the living world, and so they reject them as being unnatural or mystical and accordingly unacceptable. I see no reason to make such distinctions. Laws are laws whether or not we like them or understand them. Accordingly,
I have found the acceptance of the existence of preformed laws and informationto be a necessary and liberating asset in the ongoing search for ultimate truth concerning the two greatest mysteries in all of biological science: ontogeny and phylogeny. They are obviously intimately related just as Leo Berg so clearly recognized long ago. I now firmly believe that in solving the one we will solve the other.
References
BERG, L. (1969) Nomogenesis; or, Evolution Determined by Law.
M.I.T. Press, Cambridge. (Original Russian edition 1922.)
DAVISON, J.A. (1984) Semi-meiosis as an evolutionary mechanism.
J. Theor. Biol., 111: 725-735.
DAVISON, J.A. (1987) Semi-meiosis and evolution: a response.
J. Theor. Biol., 126: 379-381.
DAVISON, J.A. (1993) The blind alley: Its significance for
evolutionary theory. Rivista di Biologia (Biology Forum),
86: 101-110.
DAVISON, J.A. (1998) Evolution as a self-limiting process.
Rivista di Biologia (Biology Forum), 91: 199-220.
FEYNMAN, R.P. (1998) The Meaning of It All: Thoughts of a
Citizen Scientist. Addison-Wesley, Reading, Massachusetts.
GOLDSCHMIDT, R.B. (1940) The Material Basis of Evolution.
Yale University Press, New Haven.
GOULD, S.J. (1996) Full House: The Spread of Excellence from Plato
to Darwin. Harmony Books, New York.
GRASSÉ, P. (1977) Evolution of Living Organisms: Evidence
for a New Theory of Transformation. Academic Press, New York.
(Original French edition 1973.)
PUNNETT, R.C. (1915) Mimicry in Butterflies.
University Press, Cambridge.
SCHINDEWOLF, O. (1993) Basic questions in paleontology.
University of Chicago Press, Chicago. (Original German edition 1950.)
SHARP, R.G. (1914) Diplodinium ecaudatum, with an account of its
neuromotor apparatus. University of California Publications in
Zoology, 13: 43-123.
1) http://www.uvm.edu/~jdavison/ontogeny.html