https://reasonandscience.catsboard.com/t2272-chimps-our-brothers
from Marcos Eberlins excellent book:
Life and the Universe by Intelligent Design
https://www.widbook.com/ebook/read/life-and-the-universe-by-intelligent-design
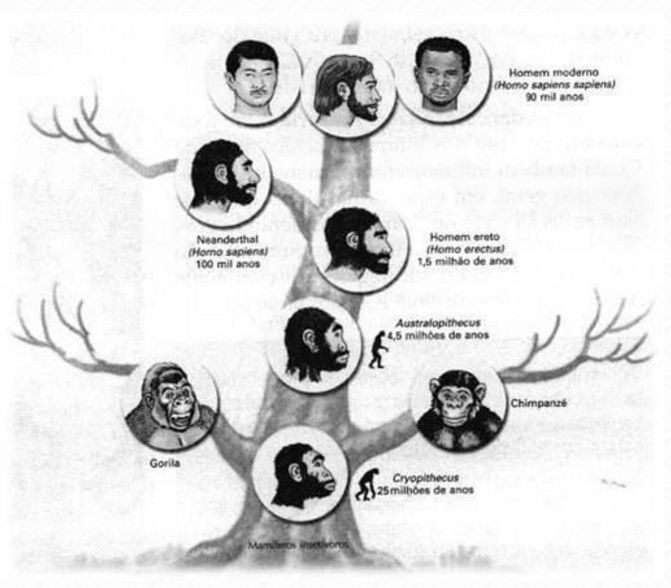
Figure 2. Branch of "legendary Tree of Life" which "illustrates" best monkey-homo sapiens evolution from the "primal hominid", the one of Dryopithecus. Is it?
Could it be that this whole story is based on facts, or are they just rumors? Then we go to them, to the facts, and ten of them, and see which of them can be concluded:
1. fossil record. Is the monkey-man evolution there documented in the fossil record - the "Museum of Life"? As we have discussed in this book, in Chapter 48, it has passed more than 150 years of serious and intensive paleontological investigation. And that scour today shows an abundant fossil record and well established, and many monkeys fossil specimens - chimpanzees, gorillas and orangutans - and humans. But how are these fossils of apes and men? The answer is clear and unequivocal: the fossils are very, very similar to apes and men we know today, and vary as the same range today. They are numerous fossils of man and ape the "way" that apes and men are today well diversified, but there in the fossil record man is man and monkey is monkey. Ie King Kong King Kong, Tarzan is Tarzan, Jane is Jane, and Chita Chita is (Figure 3).
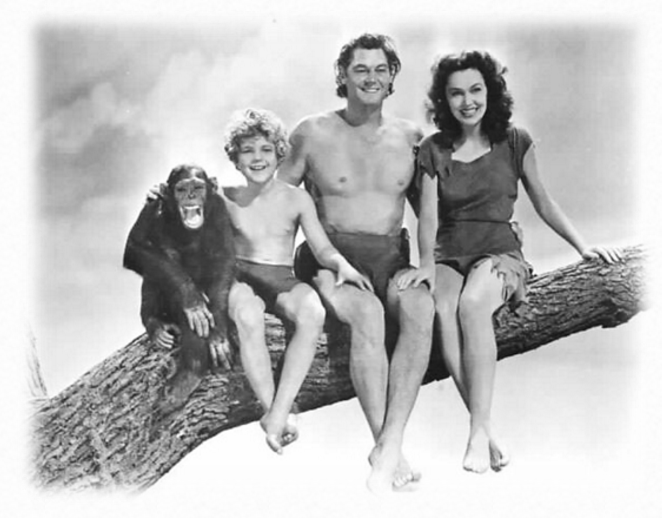
Figure 3. Tarzan, Jane and Cheetah: In the fossil record and in Hollywood, identical.
But then where are the "missing links" of transition? Where were the various links of the evolution of icons like those in Figure 2 - connecting apes and modern humans with our common ancestor ape - the Dryopithecus? Or was this a 'tabajaropithecus "? There were a number of alleged "missing links" like Lucy, as Java Man, and the Piltdown. What's more, Homo Erectus and Neanderthal man, and the latest soon to join these, Homo Nadeli. But they all, without exception, proved over time and further investigation as a farce or a big mistake. Or are even fossils of a man, or even a monkey. Ie, where inhabit these missing links ape-man? Where are they found? The answer of this "where" is again vexatious for "evolution": you will find such "missing links" only on the web, Google, blogs and fertile imagination of materialized evolutionary biologists - shamelessly - in biology textbooks via caricatures of evolution as that of Figures 1 and 2
Darwin once said: "The amount of intermediate species should be huge, and the fossil record should be full of them, and this is perhaps the most serious and most obvious objection to my theory" 1 Darwin was right because the lack of intermediate species was - and still is, and it indicates that it will keep being - the most serious objection to Darwinian theory After 150 years, the shameful absence of transitional forms in the fossil record seems to annihilate Darwin's theory completely.The total, broad and unrestricted absence of intermediate species, not just monkey-man, but rat-bat, the "dino-bird", fish mammal, and many others, or rather literally all there in the fossil record - the "Museum of Life "- wide open for those who have eyes and want to see, seems to leave no doubt of the failure of a theory that once revolutionized the world.
2. Haldane and biochemistry mathematics . Haldane was a famous British geneticist, atheist and evolutionist. Note here that since he is a geneticist, academic fellows, authors manifests will not be able to rotulate Haldane a "professional from other fields of knowledge." And for being a atheist and evolutionist, they can not classify him as a "pseudoscientist," but i can imagine they will try. Haldane became famous for developing a deadly dilemma for evolution, as calculated it would take 300,000 generations to fix a favorable mutation in a population.1 Several criticisms have been made against his calculations, but the "Haldane's Dilemma" has remained firm and strong.2,3 In 6 million years then, according to the calculations of Haldane, since supposedly the man separated from their "cousins", monkeys, only about 1,000 or about 2.000 3 of these mutations would have been fixed. But - we now know - we are about 33% genetically different from chimps.4 That is, with a 3.2 billion "bits" in the genome, we have nearly 1 billion different bits. Even using those estimates, "conservative" 3%, would still be at least 100 million.5 That is, the "wonder trio" of evolution simply had no time, even at 6 million years - because they are "slow" too - to change almost nothing in US. But we would have changed, and, 100-1000 times more than we could, according to evolution. So how do we change? Well, the calculations of Haldane were made for changes of "good" mutations, but we know today that deleterious mutations - the "evil" ones- are accumulated in us at an alarming rate, in what was called the genetic entropy, and more 100 of them at each generation.6 In other words, your child is 100 mutations worse than you, on average - despite all the effort that life does, with repair mechanisms to avoid such deleterious mutations. Perhaps, then, we have found the answer: our least 125 million bits differences were mainly caused by evil mutations. That is, yes we are "cousins" of chimpanzees, and descendants of the common ancestor ape, but we and chimpanzees really are mutant beings, and "worsened" by mutations. We "unevolved" and we were losing, for example, the hair, which warmed in the winter, and the ability to jump from branch to branch, these acrobatic skills that only apes have, and we were accumulating fat (Figure 4), and we now spend energy with talking nonsense - women, especially - and so on. But is it so?
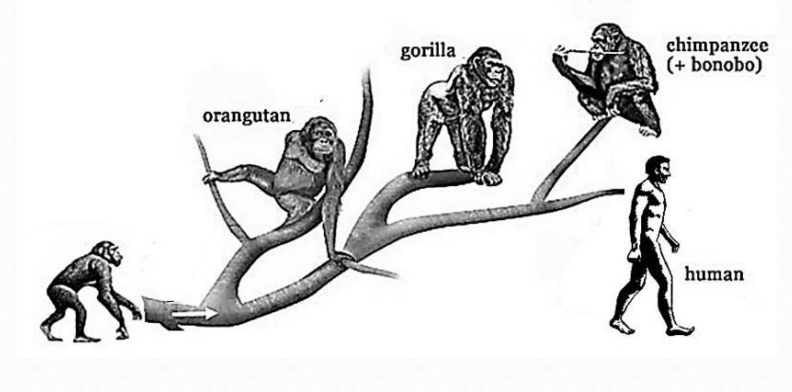
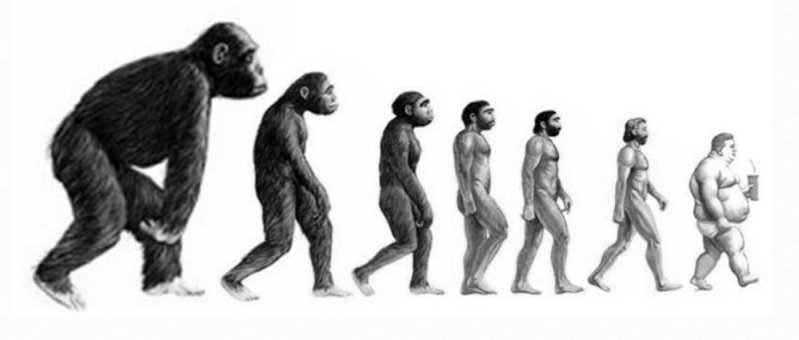
Figure 4. "involution" of common ape ancestor to the present men according to Haldane.
3. The "Argument Bill Gates." We are - argue - very similar to the monkeys; not as much morphologically - since the difference is really striking - but genetically similar. But is it so? Genetically, we are indeed a absurd degree of different, about 33% different. But is it that even 1 or 2% or 3% or 5% they calculate, 5 would it be little difference? Or even 2% would be a true evolutionary "Big Bang" ? And the "miracles" of speech and conscience, and that "white part" in our eyes? And let's just consider the genes? And proteins do not count? Are these small differences? To illustrate the enormity of the difference in the genome, we will use what I call "argument Bill Gates" and consider as certain the "miserable" 2% they calculated a day. Imagine then that the "Bill" sends you an email announcing: "Friend, you won at the internal microsoft lottery, and of everything I have, 2% is now yours" How would you react? Would you complain like this ? "But Bill Gates, only 2%?" Or would you jump with joy at the many millions that these 2% represent? Yes, even about 2% who underestimated, applied to the 3.2 billion base pairs (bits), would give about 80 million bits (80,000,000) differences. A tremendous evolutionary "Big Bang" ! A 2% "a la Bill Gates" (Figure 5) which makes Haldane squirm around in his grave.

Figure 5. The effect "Bill Gates" the difference between human and monkey genetically. Only 2% of 3.2 billion?
4. The "Argument Living Water / Watermelon / Clouds". Even if the difference between humans and apes were even those "miserable" 2%, this similarity proves what? See the argument illustrated in Figure 6. Jellyfish, watermelons and clouds are composed of 97% water. This is a fact. But what does this "apearance" shows? It shows almost nothing, just something very trivial: that things beyond different can be formed from the same material. But, through intelligent and distinct designs, completely different things can be done with only 3% difference remaining. This argument is confusing for phylogenetic analysis, and illustrates the fallacy of genomes comparison - to the "Cherry evolution," which is more "pineapple". Something that can only be sustained against an assumption: the "belief" that we evolved and that evolution is a fact - and granted. And we have-because we have - then find a culprit at all costs. And the poor monkey - who happened to be the least "unlike us" looking - was "chosen" much to distaste, I imagine. But make bad assumptions provides and forms bad scientific basis.

Figure 6. Watermelons, clouds and Jelly fish. Very similar in composition - 97% water, but coincidentally, extremely different things have been done with the remaining 3%.
5. The "myth of the 98% genetic similarity." Compare genomes is no easy task and today no one has an accurate idea of how this can be done accurately. And the greatest difficulty comes from our little knowledge of how the information stored there is used. Despite the genomes of chimpanzees and humans are sequenced, no one can agree on what would be the best way to compare them. And not what the best program is to do this. One of the most popular methods is based on an algorithm called BLAST, which cuts the DNA (or proteins) into small segments and then try to compare these segments of both. This appears to be the most "generous" to compare two genomes, and which takes "highest possible similarity", since it does not require a genome is structured similarly to each other. What matters in BLAST is a bit of information in a genome can be found anywhere in the other genome. I have my suspects about this method, and I think the DNA is so mysterious that it will take a lot to discover an accurate comparison method, but even with the "generous" BLAST, the scientific literature shows today that the genetic difference is actually much higher that the alleged 1-2% (Nature 2005, 437, 69). Comparisons of proponents of evolution between chimpanzees and human genomes have very exaggerated the similarities by not consider whole chromosomes, but only parts of them, as specific genes, and "relieve" the matching rules. Worse, comparisons have made use of pre-selection and filter levels before alignment and unaligned regions are usually omitted and gaps (gaps) in the alignments are often dismissed or artificially inserted. But when these "omissions" are properly considered and evaluated all impartially, the similarity has fallen dramatically and come to a measly 66% 4 or even less: 62%, making 15 in 33% or even 38% different from chimps.
In retail, for you to understand well, see one of the comparisons that have been made: the genomes of humans and chimpanzees have only 2.4 billion (76%) of almost 3.2 billion base pairs that align correctly. But to get a good alignment, 3% artificial gaps had to be introduced, there remaining 1.23% of differences represented by pair of bases exchanged. Moreover, there are "variations in the number of copies", which causes an additional difference of 2.7%. All in all, we share a meager similarity of only about 70% or less, or about 1/3, or 33% different (Figure 7). The worst thing is that the human genome was used as a "template" for the sequencing of the chimpanzee genome, thus assuming a priori a resemblance, which can make this "pseudosimiliarity" in fact be even lower. Faced with 33% maximum, or even the "paltry" 3% or 4% if you prefer, or any intermediate value between 33 and 3%, the most recent study i know of showed 12% difference, 14 evolution seems to get "naked with its hand in the pocket" without time and without any capacity to fulfill the "mission impossible" that it has been credited for: making of "Chimpanzees your brothers."
And remember also a fact that everyone in computer science knows very well. Developers are used to use a series of routines in common, and so all its programs tend to be - in terms of routines - very similar. This similarity is as high as about 80 to 90% of totally different programs. So are the remaining 10% that make all the difference. It is not (little) like the genetic code, but the way that this information is expressed and manipulated that makes all the difference. This stupid difference we see when comparing the "pals" with "chimps". Worse, studies have shown that we are more like gorillas than chimpanzees, 18 contrarying the tree of life represented "artistically" in Figure 3.

Figure 7. Men and chimpanzees: immense anatomical, behavioral differences, and speech and reasoning, and a genetic"Big Bang" that has been estimated at up to 33%.
6. The Y chromosome: A "shovel with a ton of lime " in the alleged common simian ancestry was launched recently when compared our Y chromosomes. 5 X and Y chromosomes are those that determine sex in mammals, including humans and then the chimpanzees. The 'male' Y chromosome has long been relegated to a mere evolutionary genetics relic, to the delight of feminists, because of its small size across the X and their repetitive sequences. The "Y" was then seen as containing few genes and missing those who had, and would be of evolutionary extinction route. But again, to the anger of proponents of evolution - as a mistaken forecast of evolution - it turned out that the "Y" is actually a "super Y" chromosome small but very powerful. For Majestic functions as a "genetic switch" controlling the expression of several other genes in the chromosomes. Its effect is so pronounced that the Y is causing the main differences between men and women. The sequencing of this "super Y" 5 then showed what, and through a chromosome so fundamental and majestic? It showed a brutal and humiliating difference (Figure 8 ) to the ape-man evolution. The differences are enormous, not only in size, in base pairs (genetic repertoire), but especially genetic architecture. So different that led David Page - the chimpanzee genome project coordinator - to consider that humans and chimps have "reinvented" their chromosomes Y. Outside the Y, huge differences are also found in other chromosomes at least 3: 4, 9:12, not to mention the 21, and all code.
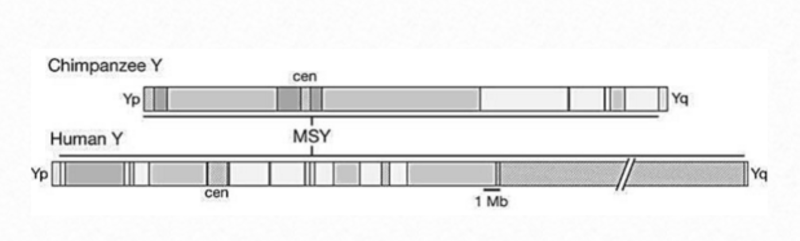
Figure 8. Comparison of the Y chromosome "Chimpanzee" and their "brothers". A "measly" 100% difference.
The authors of the Nature article - shocked, i believe, but not admitting their view as a lost case - had no choice but to appeal - and ugly - for an extremely rapid evolution of the Y chromosomes of chimpanzees and humans. In this appeal they forgot however, to inform their readers of the complete lack of time - only 6 million years - so that such genetic "mega Big Bang" could have occurred. And the worst was also they forgot to mention that the Y in humans is extremely preserved - equal to all males - which completely contradicts the idea that the Y would be mutating and evolving rapidly. The difference was so brutal and settled so fast that the authors - amazingly - compared with the genetic differences of our Y to Y chickens, which are not mammals. According to evolution, we split the chickens to 360 million years ago. In terms Y, then we are as much like chimpanzees as chickens.
And if we do not find that genetic code, to the anger of geneticists, are the best source of comparison, as recently made a geneticist, Eugene McCarthy of the University of Georgia, USA, but compare the anatomical similarities, a la Darwin and the nozzles of their finches, the best inference would be to be the result of breeding pigs with chimpanzees. Because we have many morphological characteristics similar to chimpanzees and also to pigs, so it seems we are both fruits of mating of both. Simple, right (Figure 9)?

7. Fusion of 2A + 2B chromosomes? There is still a large genetic difference between chimpanzees and humans, perhaps the most dramatic of all: humans have 23 pairs of chromosomes while the monkeys have 24. And 24 is 100% different from 23. Rationalization made to justify this "mortal difference "suggests a supposed evolutionary fusion of chromosomes 2A and 2B chimpanzee chromosome 2 in humans. It was thought then that the human chromosome 2 showed a melting point, or a kind of "genetic scar" that indicated the point where the ancestral chromosomes 2A and 2B "chimps" had merged via their telomeres, and that this scar would be proof of such a merger. But recent 6 studies have shown that there is no sign of such a scar on chromosome 2 in humans, and even more: this study presents a number of clear genetic data showing that such a merger and such scars are another two fallacies of monkey-man evolution. For example, in the supposed melting point, there is a very small number of telomere sequences expected (TTAGGG) and few of them appear connected (in "tandem), which appears to overturn the theory of chromosomal fusion via telomeres, something never seen in life . Moreover, the "centromeres" were located in very different regions other than those prescribed.
And there is more, imagine that this merger has taken place even after we split from chimpanzees. What should we then see there? Two types of humans, a guy with 24 and other with 23 pairs of chromosomes, because the merger would have occurred to a single individual while everyone else would have continued with its 24 pairs. Moreover, it is hard to imagine a very big advantage of such a merger that all humans with 24 chromosomes would have extinguished over the "super man with 23 pairs" and their descendants. That is, we are the same, it indicates 100% of chimpanzees different in terms of number of chromosome 24 because it is different 100% of 23.

Figure 9. The alleged fusion of 2A + 2B chromosomes of "chimps" to form the human chromosome 2.
8. Differences of protein content: 80% . The genetic differences between humans and apes, as we have seen, is gigantic and has ranged from 2% to 33%. But the vast majority of the genetic code - about 97-98% in humans - corresponds to the metadata (former "junk DNA"), which coordinates but does not express proteins. In an article published in 2005.7 was suggested, and I as a chemist agree - that the best way to compare men with monkeys would not be using the full genomes of the two, but the end product of these genomes expressed in the form of proteins - the building blocks of living beings. They were then compiled 127 most common proteins in humans and monkeys ("orthologous") with a total of 44 thousand amino acid sequences. And what did they find? Simply, it was discovered that humans and chimpanzees are 80% different in proteíns- as the article title 7 leaves no doubt (Figure 11).
And proteins are things so complex and finely tuned that you can not talk that a protein is virtually the same as another to have "almost" the same sequence of AA - using BLAST as a comparison method, for example - as we see in various pathologies until a simple exchange of a single AA can make the protein completely lose its function or even have quite another. This effect can be seen in the exchange of glutamic acid for valine in hemoglobin causing sickle cell anemia. Another example is the FOXP2 protein, which has only two of some 700 AAs different in chimpanzees and humans, 17 or in other words, are 99.7% similar. But FOXP2 proteins of humans have an asparagine instead of threonine at position 303 and a serine in place of an asparagine at position 325, and these "subtle" changes makes a "tremendous" difference in their functions and the regulation of their activities via phosphorylation. Thus, a very high degree of sequence similarity may be irrelevant if the AA which is different plays a crucial role, and a single or very few AAs may become a 100% protein different from each other.

Figure 11. Men and chimpanzees: immense anatomical, behavioral differences, and speech and reasoning, and proteins in a mega "Big Bang" of 80%.
9. Telomeres: At the end of each chromosome, there are very complex structures known as telomeres (Figure 12) formed by RNA, DNA and various proteins and which contains DNA repeated sequences bases TTAGGG in humans. The function assigned to telomeres is to preserve the chromosomes, protecting them from degradation, recombination and fusion. Yes, melting! Its size decreases over the cell duplication to a minimum size that stops cell proliferation, which would indicate that telomeres could also function as a cellular clock controlling the "life time". Repeated sequences of telomeres do not appear, however, only at the end of chromosomes, but are seen, and very often in inner regions of DNA in various chromosomes, as in chromosome 2 and human Y. The Y chromosome has about 0.25% of its sequence TTAGGG repeats, demonstrating that these repetitions have also "internal" function, and a further layer of information in DNA. These internal sequences also demystify the supposed evidence of chromosomal fusion 2A + 2B = 2 via telomeres already discussed. Telomeres of chimpanzees and other primates have about 23 000 repeated bases. Humans are unique among primates, and have much smaller telomeres, with about 10,000 repetitions, which makes us in terms of telomeres - only considering size, beside other differences, about 67% different.

Figure 12. The wonderful genetic engineering of telomeres, end shields of our chromosomes made up of DNA double helix tapes TTAGG repetitions, and various proteins.
10. "The pseudogene beta globin". Proponents of evolution have long used this gene as another pillar of pro-evolution argument "ape-man" and called it a "pseudogene" because "believed" it was broken. It was argued then that chimpanzees and humans - to have the same "pseudogene", shared a genetic relic from a common ancestor. But a recent study showed that this is another misconception of evolution. The remaining mutant is not useless because it has been found that the "pseudogene" HBBP1 β-globin "gene plays multiple roles in a wide variety of tissues and cell types as a regulatory feature cleverly designed and also be highly intolerant to mutations.8
And look, we set aside other huge differences. Ariano Suassuna paraphrases or quotes Beethoven and his fifth. symphony for "not humiliating chimps". And we not also speak speech capacity and human reasoning, another evolutionary "big bang" . And it as has been shown in humans that grew enclosed, the same speech in modern humans is only developed by stimulus. How then to imagine that such capacity arose without the stimulus from non-speaking beings? Biologists have wondered how humans and chimpanzees, as genetically "similar" beings - the myth of 2-3% - could be so different morphologically and intellectually? Corrected in face of more recent evidence, that question would be reformulated as follows: " How could so different beings be so different?" The answer then becomes obvious, and the question foolish . As never before, so the neo-Darwinian theory assumes that the evolutionary relationship between humans and chimpanzees today shows how an ideological delusion supported the alleged action of natural processes - natural -Selection, gene duplication and mutations occurring over millions of years - morphological superficial comparisons, and rhetorical arguments about the "why" forgetting the "how."
And because of the fault of our nearly ignorance of the genomes of chimps and humans, their Y chromosomes, and their telomeres, and everything, evolution "monkey-man" made sense for a while. But now that paleontologists have built a huge and very detailed "Museum of Life", and geneticists have unraveled the genetic codes of the two, and calculated their incorporation rates of beneficial and deleterious mutations, and also found almost zero action of natural selection on our genomes, and everything else, delirium broke, like a mirage in the desert. Like awakening from a long delusional dream.
_______
Referências e notas
1. J. B. S. Haldane "The Cost of Natural Selection", J. Genet. 1957, 55, 511.
2. "Cost theory and the cost of substitution— a clarification" TJ 2005, 199, 113.
3. "The Biotic Message", St. Paul Science, 1995, de Walter J. ReMine.
4. "Comprehensive Analysis of Chimpanzee and Human Chromosomes Reveals Average DNA Similarity of 70%" Tomkins, J. P. Answers Research Journal. 2013, 6, 63.
5. "Chimpanzee and human Y chromosomes are remarkably divergent in structure and gene content" Jennifer F. Hughes, Helen Skaletsky, Tatyana Pyntikova, Tina A. Graves, Saskia K. M. van Daalen, Patrick J. Minx, Robert S. Fulton, Sean D. McGrath, Devin P. Locke, Cynthia Friedman, Barbara J. Trask, Elaine R. Mardis, Wesley C. Warren, Sjoerd Repping, Steve Rozen, Richard K. Wilson, David C. Page Nature, 2010, 463, 536.
6. Genomic Structure and Evolution of the Ancestral Chromosome Fusion Site in 2q13–2q14.1 and Paralogous Regions on Other Human Chromosomes Yuxin Fan, Elena Linardopoulou, Cynthia Friedman, Eleanor Williams, Barbara J. Trask Genome Research 2002, 12, 1651.
7. "Eighty percent of proteins are different between humans and chimpanzees" Galina Glazko, Vamsi Veeramachaneni, Masatoshi Nei, Wojciech MakayowskiGene , 346, 215–219, 2004.
8. "Evolutionary Constraints in the β-Globin Cluster: The Signature of Purifying Selection at the δ-Globin (HBD) Locus and Its Role in Developmental Gene Regulation" Ana Moleirinho, Susana Seixas, Alexandra M. Lopes, Celeste Bento, Maria J. Prata, António Amorim, Genome Biology and Evolution, 2013, 5, 559.
9. Ann Gauger et al. Science and Human Origins, Discovery Institute Press, June 18, 2012.
10. J. C. Sanford "Genetic Entropy & The Mistery of the Genome", Elim Publishing, New York, 2005.
11. Temos 1/3 de genes específicos em humanos quando comparados com os chimpanzés segundo esse artigo: "Mapping Human Genetic Ancestry" Ingo Ebersberger, Petra Galgoczy, Stefan Taudien, Simone Taenzer, Matthias Platzer, Arndt von Haeseler, Mol. Biol. Evol. 24, 2266, 2007.
12. "Relative Differences: The Myth of 1%" John Cohen Science 316, 1836, 2007.
14. "Documented Anomaly in Recent Versions of the BLASTN Algorithm and a Complete Reanalysis of Chimpanzee and Human Genome-Wide DNA Similarity Using Nucmer and LASTZ" Jeffrey P. Tomkins Answers Research Journal 2015, 8, 379.
15. A simple statistical test for the alleged “99% genetic identity” between humans and chimps. Uncommon Descent September 17, 2010. http://www.uncommondescent.com/intelligent-design/a-simple-statistical-test-for-the-alleged-99-genetic-identity-between-humans-and-chimps/
16. “Which of Our Genes Make Us Human?” Ann Gibbons, Science 281, 1432, 1998.
17. “Molecular Evolution of FOXP2, a Gene Involved in Speech and Language” Wolfgang Enard, Molly Przeworski, Simon E. Fisher, Cecilia S. L. Lai, Victor Wiebe, Takashi Kitano, Anthony P. Monaco, Svante Pääbo Nature 418, 869, 2002.
18. “Genetic Evidence for Complex Speciation of Humans and Chimpanzees" N. Patterson et al., ” Nature 441, 315, 2006.
19. George C. Williams - outro geneticista evolucionista famoso, "afrontando" toda uma comunidade, escreveu em seu livro "Natural Selection: Domains, Levels, and Challenges", 1992, 143-144: "The Haldane's Dilemma was never solved, by Wallace or anyone else". ou em portugues: "O dilema de Haldane nunca foi solucionado, por Wallace ou quem quer que seja".
Junk DNA' defines differences between humans and chimps
https://phys.org/news/2011-10-junk-dna-differences-humans-chimps.html
The impact of retrotransposons on human genome evolution
https://www.ncbi.nlm.nih.gov/pmc/articles/PMC2884099/
Chimps, our brothers ?
https://reasonandscience.catsboard.com/t2272-chimps-our-brothers
Chimp-human-dna
https://reasonandscience.catsboard.com/t1643-chimp-human-dna
Transposons and Retrotransposons
https://reasonandscience.catsboard.com/t2393-transposons-and-retrotransposons
Early Man
http://www.detectingdesign.com/earlyman.html
Characterization and potential functional significance of human-chimpanzee large INDEL variation
https://mobilednajournal.biomedcentral.com/articles/10.1186/1759-8753-2-13
Last edited by Admin on Mon Feb 11, 2019 9:07 pm; edited 8 times in total