https://reasonandscience.catsboard.com/t2219-tetrapods-evolved-really
I thought how much brainpower was required to program and make these robots from Boston dynamics. In the natural world , according to proponents of naturalism, the required coordination and invention of new limbs was due just to random natural processes. That made me have a closer look what mainstream scientific papers have to say about the subject. How did the first limbs of tetrapods emerge ? What mechanism is required to grow body parts like legs, and how do proponents of evolution explain the arise of tetrapods ?
According to proponents of evolution, tetrapods arose from a lineage of fish. This kind of dramatic change over time is called macroevolution.
The transition from life in water to life on land would have necessitated dramatic structural changes of the whole body to withstand the increased effects of gravity, amongst other new requirements.
Many aspects of tetrapod origins remain elusive. Its supposed evolution has generated great interest, but the earliest phases of their history are poorly understood. Recent studies have questioned long-accepted hypotheses about the origin of the pentadactyl limb, the phylogeny of tetrapods and the environment in which the first tetrapods lived.
The ‘earliest’ known tetrapods with feet and legs are now thought to have been aquatic animals; proponents of evolution therefore argue that feet and legs evolved in a shallow water environment and were only later co-opted for use on the land.
Most discussions of the topic concentrate to elucidate if the fossil record permits to find transitional forms that permit infer a water to land transition. Not only are there hudge gaps, but the idea bears big problems conceptually, and as a whole.
https://reasonandscience.catsboard.com/t1808-transition-from-water-to-land-dilemma?highlight=land
Moreover, as Behe explained nicely : In order to say that some function is understood, every relevant step in the process must be elucidated. The relevant steps in biological processes occur ultimately at the molecular level, so a satisfactory explanation of a biological phenomenon such as the arise of tetrapods must include how the transition occurred on a molecular explanation. It is no longer sufficient for an ‘evolutionary explanation’ of that power to invoke only the anatomical structures of whole eyes, as Darwin did in the 19th century and as most popularizers of evolution continue to do today. Anatomy is, quite simply, irrelevant. So is the fossil record. It does not matter whether or not the fossil record is consistent with evolutionary theory, any more than it mattered in physics that Newton’s theory was consistent with everyday experience. The fossil record has nothing to tell us about, say, whether or how the interactions of 11-cis-retinal with rhodopsin, transducin, and phosphodiesterase could have developed step-by-step. Neither do the patterns of biogeography matter, or of population genetics, or the explanations that evolutionary theory has given for rudimentary organs or species abundance.
So rather than stick to anatomy comparisons of fossils that might bear some similarity that could be interpreted as intermediates and evolution of tetrapod limbs from fish fins , let us try to elucidate how significant the functional and morphological shift was it in terms of the underlying genetic mechanisms . The fossil record provides insight into supposed morphological changes. However, to understand the underlying mechanisms, we must peer into the gene regulatory networks of living vertebrates.
Do new anatomical structures arise de novo, or do they evolve from pre-existing structures? Advances in developmental genetics, palaeontology and evolutionary developmental biology have recently supposedly shed light on the origins of the structures that most intrigued Charles Darwin, including tetrapod limbs. According to proponents of evolution, structures arose by the modification of pre-existing genetic regulatory circuits.
My comment: The genetic program instructs how to make new structures, but that program must be precisely programmed, and the genetic regulatory circuits need also to be programmed . That is, two separate programs need to emerge, that is 1. the program which defines the physical form and structure, and 2. the program which instructs where to find the genetic information in the genome, and when to express it during development, that is in the right sequence. That are different layers of information, which must exist fully developed in order to make the new anatomical parts in question.
The instructions that control when and where a gene is expressed are written in the sequence of DNA bases located in the regulatory region of the gene. These instructions are written in a language that is often called the ‘gene regulatory code’. This code is read and interpreted by proteins called transcription factors that bind to specific sequences of DNA (or ‘DNA words’) and increase or decrease gene expression. Changes in gene expression between species could therefore be due to changes in the transcription factors and/or changes in the instructions within the regulatory regions of specific genes.
In order for communication to happen, 1. The sequence of DNA bases located in the regulatory region of the gene is required , and 2. transcription factors that read the code. If one of both is missing, communication fails, the gene that has to be expressed, cannot be encountered, and the whole procedure of gene expression fails. This is a irreducible complex system. The gene regulatory code could not arise in a step-wise manner either, since if that were the case, the code has only the right significance if fully developed. Thats a example par excellence of intelligent design.
https://reasonandscience.catsboard.com/t2220-shannons-theory-of-information
During vertebrate limb development, Hoxd genes are transcribed in two temporal phases; an early wave controls growth and polarity up to the forearm and a late wave patterns the digits. In this issue of Developmental Cell, Tarchini and Duboule (2006) report that two opposite regulatory modules direct early collinear expression of Hoxd genes.
Question : how could natural mechanisms have programmed and directed the right temporal phases of gene transcription of the right genes, and early wave control ? Furthermore, the limbs develop at the right place, the right coordinates and positional information is required, they could develop anywhere on the body. Did natural mechanisms find out about the right place by trial and error ? There were myriads of positions possible to add the limb. How could the right and precise coordination of axial position be achieved by mutations ?
The problem is that nature has too many options and without design couldn’t sort them all out. Natural mechanisms are too unspecific to determine any particular outcome. Mutation and natural selection could theoretically form a new complex morphological feature like a leg or a limb with the right size and form , and arrange to find out the right body location to grow them , but it could also produce all kinds of other new body forms, and grow and attach them anywhere on the body, most of which have no biological advantage or are most probably deleterious to the organism. Natural mechanisms have no constraints, they could produce any kind of novelty. Its however that kind of freedom that makes it extremely unlikely that mere natural developments provide new specific evolutionary arrangements that are advantageous to the organism. Nature would have to arrange almost a infinite number of trials and errors until getting a new positive arrangement. Since that would become a highly unlikely event, design is a better explanation.
Going over through several mainstream scientific papers, i have not come across one of them, that were able to provide a detailed description how exactly the morphological transition could have occurred through evolution.
Some biologists have also envisioned special mutations in regulatory homeobox or "Hox" genes, where simple mutations might be able to make large developmental changes in an organism which might case a radically different phenotype. However, manipulating "Hox" genes does little to solve the problem of generating novel functional biostructures, for making large changes in phenotype are rarely beneficial. Hox gene mutations may be a more simple mechanism for generating large change, but they also do not escape the problem of the "hopeful monster":"The drawback for scientists is that nature's shrewd economy conceals enormous complexity. Researchers are finding evidence that the Hox genes and the non-Hox homeobox genes are not independent agents but members of vast genetic networks that connect hundreds, perhaps thousands, of other genes. Change one component, and myriad others will change as well--and not necessarily for the better. Thus dreams of tinkering with nature's toolbox to bring to life what scientists call a "hopeful monster"- such as a fish with feet--are likely to remain elusive."Furthermore, many biologists forget when invoking Hox gene mutations that Hox genes can only re-arrange parts which are already there--they cannot create truly novel structures.
Casey Luskin : Hox mutations will never create new "body part genes", and thus cannot add truly new phenotypic functions into the genome, and at best we are left with the quandaries associated with "pre-adaptation". The majority of evolutionary change must take place through evolving new "body part genes", which Hox mutations cannot do. One reviewer in Nature recognizes this fact:"Schwartz ignores the fact that homeobox genes are selector genes. They can do nothing if the genes regulated by them are not there. It is these genes that specify in detail the adaptive structure of the organs. To be sure, turning on a homeobox gene at the wrong place can result in the appearance of an ectopic organ, but only if the genes for that organ are present in the same individual. It is totally wrong to imply that an eye could be produced by a macromutation when no eye was ever present in the lineage before.
Darwins doubt, pg.239
WHAT ABOUT HOX GENES? Hox (or homeotic) genes regulate the expression of other protein-coding genes during the process of animal development. Some biologists have likened them to the conductor of an orchestra who plays the role of coordinating the contributions of the players. And because Hox genes affect so many other genes, many evo-devo advocates think that mutations in these genes can generate large- scale changes in form.
But can mutations in Hox genes transform one form of animal life—one body plan—into another? There are several reasons to doubt that they can.
First, precisely because Hox genes coordinate the expression of so many other different genes, experimentally generated mutations in Hox genes have proven harmful. in fruit flies "most mutations in homeotic [Hox] genes cause fatal birth defects." In other cases, the resulting Hox mutant phenotype, while viable in the short term, is nonetheless markedly less fit than the wild type. For example, by mutating a Hox gene in a fruit fly, biologists have produced the dramatic Antennapedia mutant, a hapless fly with legs growing out of its head where the antennae should be.
https://reasonandscience.catsboard.com/t2077-hox-genes
Recently i saw following youtube video : https://www.youtube.com/watch?v=M8YjvHYbZ9w
The evolution of tetrapods
Evolutionary patterns in early tetrapods. I. Rapid initial diversification followed by decrease in rates of character change
two aspects of tetrapod diversification allow us to contrast predictions of intrinsic constraint (i.e. developmental or genetic) or ecological restriction (i.e. filling of general ecospace) models for reducing rates of morphological change. First, Ruta & Coates's (2006) results suggest that post-Devonian tetrapods evolved from a single Late Devonian/Early Carboniferous taxon. This phylogenetic ‘bottleneck’ (sensu Jablonski 2002) yields a second radiation into similar (i.e. semi-aquatic) ecospace; thus, if ecology alone is responsible for rates of morphological change, then we expect Early Carboniferous rates to mimic Devonian rates; however, if intrinsic constraints accumulated in the interim, then we do not. Second, the diversification of stem- and basal crown-amniotes in the Late Carboniferous represents a second invasion into a new ecospace (i.e. fully terrestrial environments). If ecological restrictions affect rates of morphological change more strongly than intrinsic constraints do, then we expect to see additional high rates of change of tetrapod diversification. Conversely, if intrinsic constraints accumulated prior to this radiation, then we might see increased disparity due to diversification, but no increase in rates.
The Fish–Tetrapod Transition
Tetrapods, for example, arose from a lineage of fish. This kind of dramatic change over time is called macroevolution.
The Devonian is often referred to as the “age of fishes” because of the astonishing diversity displayed by aquatic vertebrates during this interval. However, as is clear, terrestrial plants had evolved by the Ordovician, and insects began exploiting resources on land from the Silurian or earliest Devonian; the vertebrates would not be far behind. Over the past 20 years, a series of important papers have shed light on the sequence of character evolution associated with the first land-living, limbed vertebrates—the tetrapods
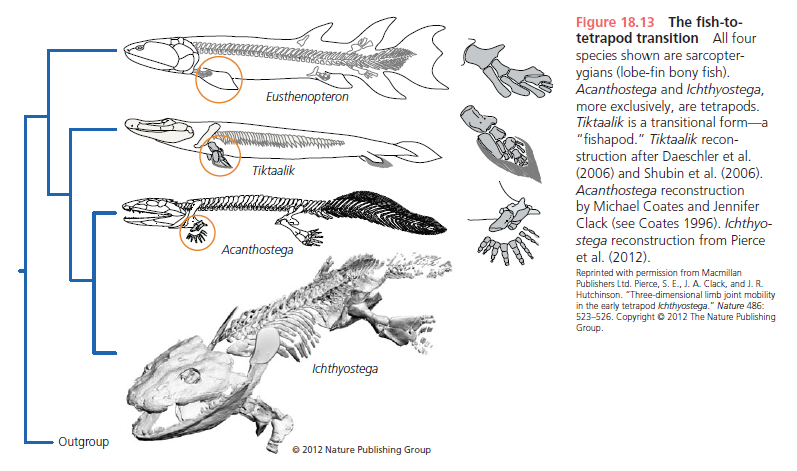
Figure 18.13 shows four Late Devonian vertebrates that span the fish-totetrapod transition. All are sarcopterygians (lobe-fin bony fish) characterized by a single proximal bone in the fin/limb recognized as the humerus (forelimb) or femur (hindlimb). In addition to modifying the fin into a limb, the transition from life in water to life on land necessitated structural changes to withstand the increased effects of gravity. Tetrapods such as Acanthostega show well-developed joints between consecutive vertebrae (zygapophyses) that are absent in aquatic taxa like Eusthenopteron, as well as enlarged rib attachments. Moreover, the pelvis was solidly connected to the vertebral column by means of novel sacral ribs. Many physiological changes undoubtedly also occurred (e.g., respiration, osmoregulation), but these are extremely difficult to gauge in the fossil record. The loss of fin rays (lepidotrichia) and their replacement by digits (Figure 18.14)
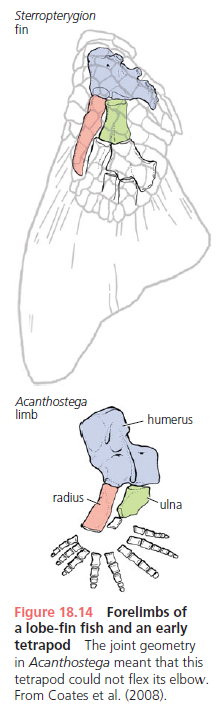
is a tetrapod hallmark, but there is increasing evidence that the earliest tetrapods spent much of their time in water (Coates et al. 2008; Pierce et al. 2012). For example, Acanthostega retained a caudal fin and had a fully formed gill skeleton. Equally surprising is the knowledge that many presumed tetrapod features were already present in derived aquatic sarcopterygians (such as Tiktaalik), like the loss of dorsal and anal fins and a flattened skull with dorsally facing orbits. Fossils of Acanthostega, Ichthyostega, and other early tetrapods were recovered from rocks interpreted as being freshwater, estuarine, or marginal marine in origin. The lack of tetrapod fossils from marine rocks helps to eliminate some prospective habitats for the origin of the group, but many aspects of tetrapod origins remain elusive. Why did tetrapods invade dry land? The classic theory is largely based on the ecology of the modern Australian lungfish, which is known to move between ponds that shrink during the dry season. Climate models suggest that the Late Devonian was substantially warmer than today, but also important were untapped foods available on land, in the form of insects, which were in turn feeding on Devonian plants. The recent discovery of tetrapod trackways from the Early Devonian of Poland (Niedzwiedzki et al. 2010; see Figure 18.4) suggests that paleontologists may need to look in rocks approximately 18 million years older than the Late Devonian rocks that currently provide the bulk of tetrapod body fossils.
Recent work has done much to illuminate the details of the origin of tetrapods. We can now see that it was a fairly gradual affair, with the transition from aquatic to fully terrestrial life stretching across the upper part of the stem group from the Panderichthys node to the base of the crown group and occupying a time interval of about 15-20 million years from the late Middle Devonian to the Early Carboniferous. However, within this extended period of change lies a brief pulse of much more rapid and dramatic morphological evolution, the traditionally recognised “origin of tetrapods”, which occupied about 5 million years and corresponds to the internode between Panderichthys and Elginerpeton. This phase of rapid change was probably driven by selection pressure for terrestrial or extreme shallow-water competence, but it is possible that pleiotropic effects due to linked gene expression patterns were also involved in some of the changes. Phylogenetic and developmental genetic data can at present only be synthesised (in a preliminary manner) in relation to the evolution of limbs.
2) Evolutionary Analysis (5th Edition) Jon C. Herron (Author), Scott Freeman page 701
Last edited by Otangelo on Wed Jan 19, 2022 8:59 am; edited 21 times in total