https://reasonandscience.catsboard.com/t2043-dna-and-rna-error-checking-and-repair-amazing-evidence-of-design
1. Organisms are constantly exposed to different environments, and in order to survive, require to be able to adapt to external conditions.
2. Life, in order to perpetuate, has to replicate. That includes DNA, which must be replicated with extreme accuracy. Somehow, the cell knows when DNA is accurately replicated, and when not. There are extremely complex quality control mechanisms in place, which constantly monitor the process. At least 3 error check and repair mechanisms keep error during replication down to 1 error in 10 billion nucleotides replicated.
3. These repair mechanisms, sophisticated proteins, are also encoded in DNA. So proteins are required to error check and repair DNA but accurately replicated DNA is necessary to make the proteins that repair DNA.
4. That is an all-or-nothing business. Therefore, these sophisticated systems had to emerge all at once, and require a designer.
Error detection and repair during the biogenesis & maturation of the ribosome, tRNA's, Aminoacyl-tRNA synthetases, and translation: by chance, or design?
1. In cells, in a variety of biochemical processes, when something goes havoc for some reason, there is readily an armada of different error check and repair mechanisms with their "antenna" out to detect errors, and correct them, preventing lethal consequences.
2. Leaking Cells membranes need to be fixed. During DNA replication, and translation, error check and repair is essential. Cells are endowed with a wide variety of specialized DNA repair mechanisms to counteract daily attacks: base excision repair, nucleotide excision repair, homologous recombination repair, mismatch repair, photoreactivation, nonhomologous end joining, translesion synthesis, and processing by the MRN complex. The Ribosome alone has 13 different error-check and repair mechanisms. In addition to repairing damage to existing DNA, living organisms have mechanisms to correct errors during reproduction. Bacteria have three types of DNA polymerase, all capable of detecting an incorrect base pairing, backing up one step to excise the incorrect nucleotide, and then progressing forward in a process called proofreading. The proofreading step decreases the error rate in bacteria from approximately one error in 100,000 base pairs to one error in 10,000,000 base pairs.
3. Molecules don't care if they are assembled in a way to bear a specific function. And if they do and the function is damaged and breaks down, those molecules neither "care" that they cease bearing that function.
4. Know how to implement an error check and repair system requires foresight. The very concepts of proofreading and repair implies goal orientation and "know-how" to keep something working and going. Those things can only come from an intelligent agency which implements these systems for specific purposes.
The concepts of machine and factory error monitoring, checking, and repair are all tasks performed with goal-directedness, intent, and purpose.
1. Repairing things that are broken, malfunctioning, or instantiating complex systems that autonomously prevent things to break are always actions performed by agents with intentions, volition, goal-orientedness, foresight, understanding, and know-how.
2. Man-made machines almost always require direct intelligent intervention by technicians to recognize errors, find which parts of a machine are broken, know how to remove and replace them without breaking surrounding parts of the device, and know how to construct the part that has to be replaced with fidelity, and re-insert and re-connect it where the part was removed. The entire process is complex, demanding know-how, and depends on a high quantity of intelligence in performing all involved actions.
3. Man has not been able to create a fully autonomous, preprogrammed machine or factory, that is able to quality and error monitor all manufacturing processes and the correct performance of all devices involved, and if the products are up to the required quality standard, and, if something drives havoc, repair and re-establish normal function of what was broken or malfunctioning without external intervention.
4. C.H. Loch writes in the science paper: "Organic Production Systems: What the Biological Cell Can Teach Us About Manufacturing" (2004): Biological cells are preprogrammed to use quality-management techniques used in manufacturing today. The cell invests in defect prevention at various stages of its replication process, using 100% inspection processes, quality assurance procedures, and foolproofing techniques. An example of the cell inspecting each and every part of a product is DNA proofreading. As the DNA gets replicated, the enzyme DNA polymerase adds new nucleotides to the growing DNA strand, limiting the number of errors by removing incorrectly incorporated nucleotides with a proofreading function. Following is an impressive example: Unbroken DNA conducts electricity, while an error blocks the current. Some repair enzymes exploit this. One pair of enzymes lock onto different parts of a DNA strand. One of them sends an electron down the strand. If the DNA is unbroken, the electron reaches the other enzyme and causes it to detach. I.e. this process scans the region of DNA between them, and if it’s clean, there is no need for repairs. But if there is a break, the electron doesn’t reach the second enzyme. This enzyme then moves along the strand until it reaches the error, and fixes it. This mechanism of repair seems to be present in all living things, from bacteria to man. Know-how is needed:
a. To know that something is broken (DNA damage sensing)
b. To identify where exactly it is broken
c. To know when to repair it (e.g. one has to stop/or put on hold some other ongoing processes, in other words, one needs to know lots of other things, one needs to know the whole system, otherwise one creates more damage…)
d. to know how to repair it (to use the right tools, materials, energy, etc, etc, etc )
e. to make sure that the repair was performed correctly. (this can be observed in DNA repair as well)
5. On top of that: Cells do not even wait until a protein machine fails, but replace it long before it has a chance to break down. Furthermore, it completely recycles the machine that is taken out of production. The components derived from this recycling process can be used not only to create other machines of the same type but also to create different machines if that is what is needed in the “plant.” This way of handling its machines has some clear advantages for the cell. New capacity can be installed quickly to meet current demand. At the same time, there are never idle machines around taking up space or hogging important building blocks. Maintenance is a positive “side effect” of the continuous machine renewal process, thereby guaranteeing the quality of output. Finally, the ability to quickly build new production lines from scratch has allowed the cell to take advantage of a big library of contingency plans in its DNA that allow it to quickly react to a wide range of circumstances, as we will describe next.
6. The more sophisticated, advanced, autonomous, complex, and information-driven machines or factories are, the more they carry the hallmark of design. The very concepts of error monitoring, checking, and repair, and replacement in advance to avoid future break-ups are tasks performed with goal-directedness, and purpose. Biological cells are far more advanced than any machine and factory ever devised and invented by man. It is therefore rational and warranted to infer, that biological cells were designed.
Tan, Change; Stadler, Rob. The Stairway To Life, page 112:
Fortunately, living organisms are endowed with a wide variety of specialized DNA repair mechanisms to counteract these daily attacks: base excision repair, nucleotide excision repair, homologous recombination repair, mismatch repair, photoreactivation, nonhomologous end joining, translesion synthesis, and processing by the MRN complex. The base excision repair mechanism occurs in prokaryotes and eukaryotes and requires the coordinated efforts of at least five enzymes to make small repairs to DNA. The nucleotide excision repair mechanism, also highly prevalent throughout life, targets more extensive damage. In E. coli, nucleotide excision repair requires five enzymes to replace a strip of twelve nucleotides when DNA damage is discovered. These repair pathways preserve information via very specific applications of energy; production and function of the enzymes both require energy.
In addition to repairing damage to existing DNA, living organisms have mechanisms to correct errors during reproduction. Bacteria have three types of DNA polymerase, all capable of detecting an incorrect base pairing, backing up one step to excise the incorrect nucleotide, and then progressing forward in a process called proofreading. The proofreading step decreases the error rate in bacteria from approximately one error in 100,000 base pairs to one error in 10,000,000 base pairs. The critical importance of DNA repair mechanisms in all living organisms immediately produces a conundrum: the DNA repair mechanisms are themselves encoded in DNA that requires repair mechanisms. Imagine that the world is full of thieves, and you have the world’s first idea for a security system that will deter all thieves. You plan to manufacture the security system in a factory. However, as you begin construction on the factory, thieves continuously steal the blueprints, the raw materials, even the funds needed to build the factory. The factory will never be completed, and a security system will never be produced. Similarly, DNA repair mechanisms could not have evolved without the protection of DNA repair mechanisms. And ordinary DNA could not have evolved before DNA repair genes evolved.
Maintaining the genetic stability that an organism needs for its survival requires not only an extremely accurate mechanism for replicating DNA, but also mechanisms for repairing the many accidental lesions that occur continually in DNA. Its evident that the repair mechanism is essential for the cell to survive. It could not have evolved after life arose, but must have come into existence before. The mechanism is highly complex and elaborated, as consequence, the design inference is justified and seems to be the best way to explain its existence.
5ʹ => 3ʹ polymerization 1 in 100.000
3ʹ => 5ʹ exonucleolytic proofreading 1 in 100
Strand-directed mismatch repair 1 in 1000
Combined 1 in 10.000.000.000
Jon Lieff MD:DNA Proofreading, Correcting Mutations during Replication, Cellullar Self Directed Engineering
During replication, nucleotides, which compose DNA, are copied. When E coli makes a copy of its DNA, it makes approximately one mistake for every billion new nucleotides. It can copy about 2000 letters per second, finishing the entire replication process in less than an hour. Compared to human engineering, this error rate is amazingly low. E coli makes so few errors because DNA is proofread in multiple ways. An enzyme, DNA polymerase, moves along the DNA strands to start copying the code from each strand of DNA. This process has an error rate of about one in 100,000: rather high. When an error occurs, though, DNA polymerase senses the irregularity as a distortion of the new DNA’s structure and stops what it is doing. How a protein can sense this is not clear. Other molecules then come to fix the mistake, removing the mistaken nucleotide base and replacing it with the correct one. After correction, the polymerase proceeds. This correction mechanism increases the accuracy 100 to 1000 times.
A Second Round of Proofreading
There are still some errors, however, that escape the previous mechanism. For those, three other complex proteins go over the newly copied DNA sequence. The first protein, called MutS (for mutator), senses a distortion in the helix shape of the new DNA and binds to the region with the mistaken nucleotides. The second protein, MutL, senses that its brother S is attached and brings a third protein over and attaches the two. The third molecule actually cuts the mistake on both sides. The three proteins then tag the incorrect section with a methyl group. Meanwhile, another partial strand of DNA is being created for the region in question, and another set of proteins cut out the exact amount of DNA needed to fill the gap. With both the mistaken piece and newly minted correct piece present, yet another protein determines which is the correct one by way of the methyl tag. That is, the correct one does not have the methyl tag on it. This new, correct section is then brought over and added to the original DNA strand. This second proofreading is itself 99% efficient and increases the overall accuracy of replication by another 100 times.Multiple Sensors
There are multiple places where a protein “senses” what needs to be done. The computer-like sensing of the original mistake, cannot be directed by the original DNA. Clearly, there are other sources of decision-making in a cell. While DNA’s “quality control” is extremely complex in E.Coli, the same process is even more complex in the human cell. Human cells contain many different polymerases and many other enzymes to cut and mend mistakes. There are even different Mut-type systems that, along with other proofreading, render human DNA replication incredibly accurate. Very recent research has shown some of the complex mechanisms of the MutL family of mutation correction molecules. It shows that an energy molecule ATP stimulates the process whereby MutL cuts the DNA around the error. There are two grooves in the MutL molecule, one for ATP and one for the DNA strand. When ATP binds to MutL it changes the protein’s shape which allows the cutting to occur. In humans when MutL is not functioning properly it is known to cause cancer.
While mutations help determine evolutionary variety, we still don’t know how these very elaborate and multi-layered quality controls came about and how they are directed. Is it possible for DNA to directed its own editing? Somehow, these processes know which are appropriate DNA sequences and which are not.
Cellular Repair Capabilities. 20
First, then, all cells from bacteria to man possess a truly astonishing array of repair systems that serve to remove accidental and stochastic sources of mutation. Multiple levels of proofreading mechanisms recognize and remove errors that inevitably occur during DNA replication. These proofreading systems are capable of distinguishing between newly synthesized and parental strands of the DNA double helix, so they operate efficiently to rectify rather than fix the results of accidental misincorporations of the wrong nucleotide. Other systems scan non-replicating DNA for chemical changes that could lead to miscoding and remove modified nucleotides, while additional functions monitor the pools of precursors and remove potentially mutagenic contaminants. In anticipation of chemical and physical insults to the genome, such as alkylating agents and ultraviolet radiation,
additional repair systems are encoded in the genome and can be induced to correct damage when it occurs. It has been a surprise to learn how thoroughly cells protect themselves against precisely the kinds of accidental genetic change that, according to conventional theory, are the sources of evolutionary variability. By virtue of their proofreading and repair systems, living cells are not passive victims of the random forces of chemistry and physics. They devote large resources to suppressing random genetic variation and have the capacity to set the level of background localized mutability by adjusting the activity of their repair systems.
https://reasonandscience.catsboard.com/t2043-dna-and-rna-error-checking-and-repair-amazing-evidence-of-design
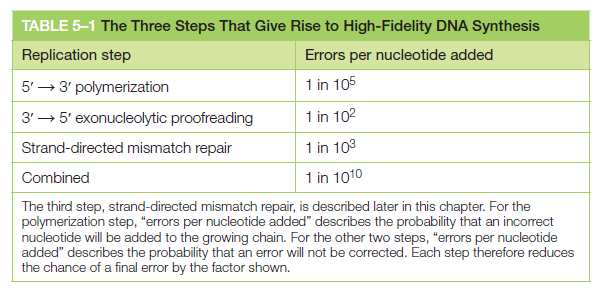
DNA damage is an alteration in the chemical structure of DNA, such as a break in a strand of DNA, a base missing from the backbone of DNA, or a chemically changed base. Naturally occurring DNA damages arise more than 60,000 times per day per mammalian cell. DNA damage appears to be a fundamental problem for life. DNA damages are a major primary cause of cancer. DNA damages give rise to mutations and epimutations. The mutations, if not corrected, would be propagated throughout subsequent cell generations. Such a high rate of random changes in the DNA sequence would have disastrous consequences for an organism
Different pathways for DNA repair exists,
Nucleotide excision repair (NER),
Base excision repair (BER),
DNA mismatch repair (MMR),
Repair through alkyltransferase-like proteins (ATLs) amongst others.
Base excision repair (BER) involves a category of enzymes known as DNA-N-glycosylases.
One example of DNA's automatic error-correction utilities are enough to stagger the imagination. There are dozens of repair mechanisms to shield our genetic code from damage; one of them was portrayed in Nature in terms that should inspire awe.
From Nature's article :
Structure of a repair enzyme interrogating undamaged DNA elucidates recognition of damaged DNA 11
How DNA repair proteins distinguish between the rare sites of damage and the vast expanse of normal DNA is poorly understood. Recognizing the mutagenic lesion 8-oxoguanine (oxoG) represents an especially formidable challenge, because this oxidized nucleobase differs by only two atoms from its normal counterpart, guanine (G). The X-ray structure of the trapped complex features a target G nucleobase extruded from the DNA helix but denied insertion into the lesion recognition pocket of the enzyme. Free energy difference calculations show that both attractive and repulsive interactions have an important role in the preferential binding of oxoG compared with G to the active site. The structure reveals a remarkably effective gate-keeping strategy for lesion discrimination and suggests a mechanism for oxoG insertion into the hOGG1 active site.
Of the four bases in DNA (C, G, A, and T) cytosine or C is always supposed to pair with guanine, G, and adenine, A, is always supposed to pair with thymine, T. The enzyme studied by Banerjee et al. in Nature is one of a host of molecular machines called BER glycosylases; this one is called human oxoG glycosylase repair enzyme (hOGG1), and it is specialized for finding a particular type of error: an oxidized G base (guanine). Oxidation damage can be caused by exposure to ionizing radiation (like sunburn) or free radicals roaming around in the cell nucleus. The normal G becomes oxoG, making it very slightly out of shape. There might be one in a million of these on a DNA strand. While it seems like a minor typo, it can actually cause the translation machinery to insert the wrong amino acid into a protein, with disastrous results, such as colorectal cancer. 12
The machine latches onto the DNA double helix and works its way down the strand, feeling every base on the way. As it proceeds, it kinks the DNA strand into a sharp angle. It is built to ignore the T and A bases, but whenever it feels a C, it knows there is supposed to be a G attached. The machine has precision contact points for C and G. When the C engages, the base paired to it is flipped up out of the helix into a slot inside the enzyme that is finely crafted to mate with a pure, clean G. If all is well, it flips the G back into the DNA helix and moves on. If the base is an oxoG, however, that base gets flipped into another slot further inside, where powerful forces yank the errant base out of the strand so that other machines can insert the correct one.
Now this is all wonderful stuff so far, but as with many things in living cells, the true wonder is in the details. The thermodynamic energy differences between G and oxoG are extremely slight – oxoG contains only one extra atom of oxygen – and yet this machine is able to discriminate between them to high levels of accuracy.
The author, David, says in the Nature article :
Structural biology: DNA search and rescue
DNA-repair enzymes amaze us with their ability to search through vast tracts of DNA to find subtle anomalies in the structure. The human repair enzyme 8-oxoguanine glycosylase (hOGG1) is particularly impressive in this regard because it efficiently removes 8-oxoguanine (oxoG), a damaged guanine (G) base containing an extra oxygen atom, and ignores undamaged bases.
Natural selection cannot act without accurate replication, yet the protein machinery for the level of accuracy required is itself built by the very genetic code it is designed to protect. Thats a catch22 situation. It would have been challenging enough to explain accurate transcription and translation alone by natural means, but as consequence of UV radiation, it would have quickly been destroyed through accumulation of errors. So accurate replication and proofreading are required for the origin of life. How on earth could proofreading enzymes emerge, especially with this degree of fidelity, when they depend on the very information that they are designed to protect? Think about it.... This is one more prima facie example of chicken and egg situation. What is the alternative explanation to design ? Proofreading DNA by chance ? And a complex suite of translation machinery without a designer?
I enjoy to learn about the wonder of these incredible mechanisms. If the apostle Paul could understand that creation demands a Creator as he wrote in Romans chapter one 18, how much more we today with all the revelations about cell biology and molecular machines?
https://reasonandscience.catsboard.com/t2043-dna-repair#3475
DNA repair mechanisms, designed with special care in order to provide integrity of DNA, and essential for living organisms of all domains.
In fact, Nature uses special proteins called ‘proofreading enzymes’ to prevent the occurrence of slight changes in sequence when DNA replicates.
Inaccurate replication would likely have limited the size of the progenote genome due to the risk of “error catastrophe,” the accumulation of so many genetic mistakes that the organism is no longer viable. To illustrate this point, consider the problem of replicating a genome of one million bases, which is sufficient to encode a few hundred RNAs and proteins. (The smallest known genome for an extant free-living bacterium is that of Pelagibacter ubique, which consists of 1.3 million bases.) If replication were even modestly faithful, with an error frequency of 0.1%, every replication of a genome consisting of 1 million bases would result in 1000 errors, approximately one or two in every gene. Some of those errors would have been harmless, and a few might have been beneficial, but many would have been detrimental, leading to macromolecules with impaired functions.
DNA damage and repair
https://reasonandscience.catsboard.com/t2043-dna-repair?highlight=dna+repair
https://reasonandscience.catsboard.com/t1849p30-dna-replication-of-prokaryotes#4401
Replication forks may stall frequently and require some form of repair to allow completion of chromosomal duplication. Failure to solve these replicative problems comes at a high price, with the consequences being genome instability, cell death and, in higher organisms, cancer. Replication fork repair and hence reloading of DnaB may be needed away from oriC at any point within the chromosome and at any stage during chromosomal duplication. The potentially catastrophic effects of uncontrolled initiation of chromosomal duplication on genome stability suggests that replication restart must be regulated as tightly as DnaA-directed replication initiation at oriC. This implies reloading of DnaB must occur only on ssDNA at repaired forks or D-loops rather than onto other regions of ssDNA, such as those created by blocks to lagging strand synthesis.Thus an alternative replication initiator protein, PriA helicase, is utilized during replication restart to reload DnaB back onto the chromosome
Question: Could the first cell, with its required complement of genes coded for by DNA, have successfully reproduced for a significant number of generations without a proofreading function? A further question is how the function of synthesis of the lagging strand could have arisen, and the machinery to do so. That is, the Primosome, and the function of Polymerase I to remove the short peaces of RNA that the cell uses to prime replication, allowing the polymerase III function to fill the gap. These functions all require precise regulation, and coordinated functional machine-like steps. These are all complex, advanced functions and had to be present right from the beginning. How could this complex machinery have emerged in a gradual manner? the Primosome had to be fully functional, otherwise, polymerisation could not have started, since a prime sequence is required.
The enzymes that copy DNA to DNA, or DNA to RNA, are indeed very clever. They can sense at several stages during synthesis whether anything is going wrong; for example, if they have added or are about to add the wrong base, according to the Watson–Crick rules of pairing. Also, there are ‘repair’ enzymes that go around correcting occasional mistakes of copying or ‘mismatches’. Thus, Nature goes to great lengths to avoid errors in the copying of DNA, even though the atoms in the DNA structure are actually quite tolerant of mismatch pairings. These enzymes are extremely efficient in doing their job, yet no one knows exactly how they work.
The evidence through DNA repair
1. Broken or mismatched DNA strands can lead to serious diseases and even death. It is essential that DNA damage is recognized and repaired quickly.
2. A team at Rockefeller University and Harvard Medical School that found two essential proteins that act like “molecular tailors” that can snip out an error and sew it back up with the correct molecules.
3. These proteins, FANC1 and FANCD2, repair inter-strand cross-links, “one of the most lethal types of DNA damage.” This problem “occurs when the two strands of the double helix are linked together, blocking replication and transcription.”
4. Each of your cells is likely to get 10 alarm calls a day for inter-strand cross-links.
5. The FANC1 and FANCD2 link together and join other members of the repair pathway, and are intimately involved in the excision and insertion steps.
6. One repair operation requires 13 protein parts.
7. “If any one of the 13 proteins in this pathway is damaged, the result is Fanconi anemia, a blood disorder that leads to bone marrow failure and leukemia, among other cancers, as well as many physiological defects.”
a. “Our results show that multiple steps of the essential S-phase ICL repair mechanism fail when the Fanconi anemia pathway is compromised.”
8. In the scientific paper and press release nor Darwin nor the possible way of how this tightly-integrated system might have evolved was mentioned.
9. The absolute necessity of FANC1 and FANCD2 are very much obvious from this discovery not only in one species but in all that has DNA. Their crucial role for survival of the species is undismissable.
10. There must have existed as perfectly functional units from the time of appearance of any species on this planet otherwise existence would be not possible.
11. This implies creation what further implies that God necessarily exists.
Reference:
1. Knipscheer et al, “The Fanconi Anemia Pathway Promote Replication-Dependent DNA Interstrand Cross-Link Repair,” Science, 18 December 2009: Vol. 326. no. 5960, pp. 1698-1701, DOI: 10.1126/science.1182372.
Argument from detection/correction codes
1. The GCL binary representation makes possible the existence of error detection/correction codes that operate along the strands of DNA.
2. “An error-control mechanism implies the organization of the redundancy in a mathematically structured way,” and “the genetic code exhibits a strong mathematical structure that is difficult to put in relation with biological advantages other than error correction.”
3. A peculiar and unique mathematical model accounts for the key properties of the genetic code that exhibits symmetry, organized redundancy, and a mathematical structure crucial for the existence of error-coding techniques operating along the DNA strands.
4. The DNA data tested using this model gave a strong indication that error-coding techniques do exist.
5. Such a wonderful design indicates purposeful creation that further indicates the existence of God.
6. God most probably exists.
The evidence of Rad51
1. The scientists from the Lawrence Berkeley National Lab in their essay: “Safeguarding genome integrity through extraordinary DNA repair,” write:
Homologous recombination is a complex mechanism with multiple steps, but also with many points of regulation to ensure accurate recombination at every stage. This could be why this method has been favored during evolution. The machinery that relocalizes the damaged DNA before loading Rad51 might have evolved because the consequences of not having it would be terrible.
2. If evolution is a chance process with no goal or purpose, it would not care if something emerges or not. How can a mindless process “favor” a method? How would a mindless process “know” that the consequences of not having something would be terrible? How would that motivate a non-mind to produce machinery and complex mechanisms to avoid terrible consequences?
3. Thus instead of saying ‘Rad51 might have evolved’ it is clear that Rad51 was designed by an intelligent designer since without such a complex mechanism with multiple steps with many points of regulation to insure accurate recombination at every stage, life could not exist.
4. The ability of Rad51 that has the ability of extraordinary DNA repair proofs the existence of an intelligent designer all men call God.
5. God most probably exists.
DNA repair
http://reasonandscience.heavenforum.org/t2043-dna-repair?highlight=dna+repair
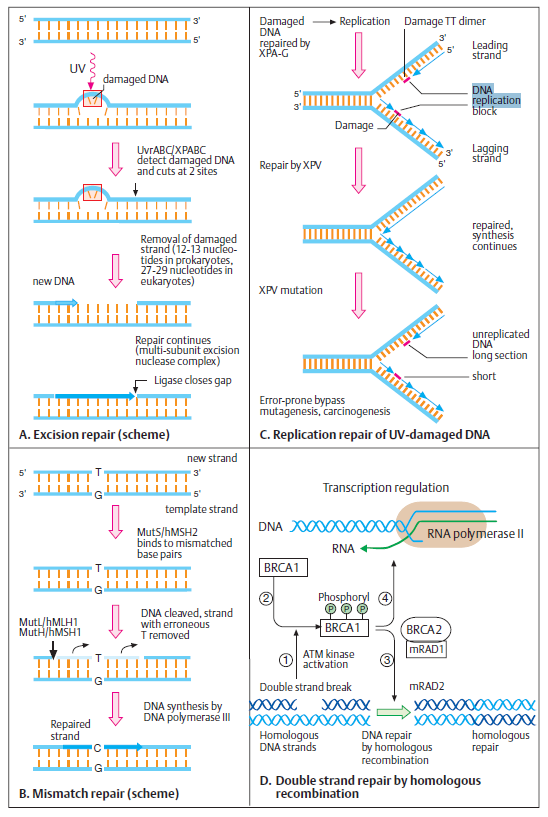
DNA repair mechanisms make no sense in an evolutionary presupposition. Error correction requires error detection, and that requires the detection process to be able to compare the DNA as it is to the way it ought to be. DNA repair is regarded as one of the essential events in all life forms. 18 The stability of the genome is essential for the proper function and survival of all organisms. DNA damage is very frequent and appears to be a fundamental problem for life. DNA damage can trigger the development of cancer, and accelerate aging. 19
Kunkel, T.A., DNA Replication Fidelity, J. Biological Chemistry 279:16895–16898, 23 April 2004.
This machinery keeps the error rate down to less than one error per 100 million letters
Maintaining the genetic stability that an organism needs for its survival requires not only an extremely accurate mechanism for replicating DNA, but also mechanisms for repairing the many accidental lesions that occur continually in DNA. Most such spontaneous changes in DNA are temporary because they are immediately corrected by a set of processes that are collectively called DNA repair. Of the thousands of random changes created every day in the DNA of a human cell by heat, metabolic accidents, radiation of various sorts, and exposure to substances in the environment, only a few accumulate as mutations in the DNA sequence. For example, we now know that fewer than one in 1000 accidental base changes in DNA results in a permanent mutation; the rest are eliminated with remarkable efficiency by DNA repair. The importance of DNA repair is evident from the large investment that cells make in DNA repair enzymes. For example, analysis of the genomes of bacteria and yeasts has revealed that several percent of the coding capacity of these organisms is devoted solely to DNA repair functions.
Without DNA repair, spontaneous DNA damage would rapidly change DNA sequences
Although DNA is a highly stable material, as required for the storage of genetic information, it is a complex organic molecule that is susceptible, even under normal cell conditions, to spontaneous changes that would lead to mutations if left unrepaired.
DNA damage is an alteration in the chemical structure of DNA, such as a break in a strand of DNA, a base missing from the backbone of DNA, or a chemically changed base. 15
Naturally occurring DNA damages arise more than 60,000 times per day per mammalian cell.
DNA damage appears to be a fundamental problem for life. DNA damages are a major primary cause of cancer. DNA damages give rise to mutations and epimutations that, by a process of natural selection, can cause progression to cancer. 16
Different pathways to repair DNA
DNA repair mechanisms fall into 2 categories
– Repair of damaged bases
– Repair of incorrectly basepaired bases during replication
Cells have multiple pathways to repair their DNA using different enzymes that act upon different kinds of lesions.
At least four excision repair pathways exist to repair single stranded DNA damage:
Nucleotide excision repair (NER)
Base excision repair (BER)
DNA mismatch repair (MMR)
Repair through alkyltransferase-like proteins (ATLs)
In most cases, DNA repair is a multi-step process
– 1. An irregularity in DNA structure is detected
– 2. The abnormal DNA is removed
– 3. Normal DNA is synthesized
DNA bases are also occasionally damaged by an encounter with reactive metabolites produced in the cell (including reactive forms of oxygen) or by exposure to chemicals in the environment. Likewise, ultraviolet radiation from the sun can produce a covalent Iinkage between two adjacent pyrimidine bases in DNA to form, for example, thymine dimers This type of damage occurs in the DNA of cells exposed to ultraviolet or radiation(as in sunlight) A similar dimer will form between any two neighboring pyrimidine bases ( C or T residues ) in DNA. ( see below )
If left uncorrected when the DNA is replicated, most of these changes would be expected to lead either to the deletion of one or more base pairs or to a base-pair substitution in the daughter DNA chain. ( see below ) The mutations would then be propagated throughout subsequent cell generations. Such a high rate of random changes in the DNA sequence would have disastrous consequences for an organism
Its evident that the repair mechanism is essential for the cell to survive. It could not have evolved after life arose, but must have come into existence before. The mechanism is highly complex and elaborated, as consequence, the design inference is justified and seems to be the best way to explain its existence.
The DNA double helix is readily repaired
The double-helical structure of DNA is ideally suited for repair because it carries two separate copies of all the genetic information-one in each of its two strands. Thus, when one strand is damaged, the complementary strand retains an intact copy of the same information, and this copy is generally used to restore the correct nucleotide sequences to the damaged strand. An indication of the importance of a double-stranded helix to the safe storage of genetic information is that all cells use it; only a few small viruses use single stranded DNA or RNA as their genetic material. The types of repair processes described in this section cannot operate on such nucleic acids, and once damaged, the chance of a permanent nucleotide change occurring in these singlestranded genomes of viruses is thus very high. It seems that only organisms with tiny genomes (and therefore tiny targets for DNA damage) can afford to encode their genetic information in any molecule other than a DNA double helix.Below shows two of the most common pathways. In both, the damage is excised, the original DNA sequence is restored by a DNA polymerase that uses the undamaged strand as its template, and a remaining break in the double helix is sealed by DNA ligase.
DNA ligase.
The reaction catalyzed by DNA ligase. This enzyme seals a broken phosphodiester bond. As shown, DNA ligase uses a molecule of ATP to activate the 5' end at the nick (step 1 ) before forming the new bond (step 2). In this way, the energetically unfavorable nick-sealing reaction is driven by being coupled to the energetically favorable
process of ATP hydrolysis.
The main two pathways differ in the way in which they remove the damage from DNA. The first pathway, called
Base excision repair (BER) 9
It involves a battery of enzymes called DNA glycosylases, each of which can recognize a specific tlpe of altered base in DNA and catalyze its hydrolltic removal. There are at least six types of these enzymes, including those that remove deaminated Cs, deaminated As, different types of alkylated or oxidized bases, bases with opened rings, and bases in which a carbon-carbon double bond has been accidentally converted to a carbon-carbon single bond.
How is an altered base detected within the context of the double helix? A key step is an enzyme-mediated "flipping-out" of the altered nucleotide from the helix, which allows the DNA glycosylase to probe all faces of the base for damage ( see above image ) It is thought that these enzymes travel along DNA using base-flipping to evaluate the status of each base. Once an enzyme finds the damaged base that it recognizes, it removes the base from its sugar. The "missing tooth" created by DNA glycosylase action is recognized by an enzyme called AP endonuclease (AP for apurinic or apyrimidinic, endo to signify that the nuclease cleaves within the polynucleotide chain), which cuts the phosphodiester backbone, after which the damage is removed and the resulting gap repaired ( see figure below ) Depurination, which is by far the most frequent rype of damage suffered by DNA, also leaves a deoxyribose sugar with a missing base. Depurinations are directly repaired beginning with AP endonuclease.
While the BER pathway can recognize specific non-bulky lesions in DNA, it can correct only damaged bases that are removed by specific glycosylases. Similarly, the MMR pathway only targets mismatched Watson-Crick base pairs. 2
Molecular lesion A molecular lesion or point lesion is damage to the structure of a biological molecule such as DNA, enzymes, or proteins that results in reduction or absence of normal function or, in rare cases, the gain of a new function. Lesions in DNA consist of breaks and other changes in the chemical structure of the helix (see types of DNA lesions) while lesions in proteins consist of both broken bonds and improper folding of the amino acid chain. 6
DNA-N-glycosylases
Base excision repair (BER) involves a category of enzymes known as DNA-N-glycosylases These enzymes can recognize a single damaged base and cleave the bond between it and the sugar in the DNA removes one base, excises several around it, and replaces with several new bases using Pol adding to 3’ ends then ligase attaching to 5’ end
DNA glycosylases are a family of enzymes involved in base excision repair, classified under EC number EC 3.2.2. Base excision repair is the mechanism by which damaged bases in DNA are removed and replaced. DNA glycosylases catalyze the first step of this process. They remove the damaged nitrogenous base while leaving the sugar-phosphate backbone intact, creating an apurinic/apyrimidinic site, commonly referred to as an AP site. This is accomplished by flipping the damaged base out of the double helix followed by cleavage of the N-glycosidic bond. Glycosylases were first discovered in bacteria, and have since been found in all kingdoms of life. 8
One example of DNA's automatic error-correction utilities are enough to stagger the imagination. There are dozens of repair mechanisms to shield our genetic code from damage; one of them was portrayed in Nature in terms that should inspire awe. 10
How do DNA-repair enzymes find aberrant nucleotides among the myriad of normal ones?
One enzyme has been caught in the act of checking for damage, providing clues to its quality-control process.
From Nature's article :
Structure of a repair enzyme interrogating undamaged DNA elucidates recognition of damaged DNA 11
How DNA repair proteins distinguish between the rare sites of damage and the vast expanse of normal DNA is poorly understood. Recognizing the mutagenic lesion 8-oxoguanine (oxoG) represents an especially formidable challenge, because this oxidized nucleobase differs by only two atoms from its normal counterpart, guanine (G). The X-ray structure of the trapped complex features a target G nucleobase extruded from the DNA helix but denied insertion into the lesion recognition pocket of the enzyme. Free energy difference calculations show that both attractive and repulsive interactions have an important role in the preferential binding of oxoG compared with G to the active site. The structure reveals a remarkably effective gate-keeping strategy for lesion discrimination and suggests a mechanism for oxoG insertion into the hOGG1 active site.
Of the four bases in DNA (C, G, A, and T) cytosine or C is always supposed to pair with guanine, G, and adenine, A, is always supposed to pair with thymine, T. The enzyme studied by Banerjee et al. in Nature is one of a host of molecular machines called BER glycosylases; this one is called human oxoG glycosylase repair enzyme (hOGG1), and it is specialized for finding a particular type of error: an oxidized G base (guanine). Oxidation damage can be caused by exposure to ionizing radiation (like sunburn) or free radicals roaming around in the cell nucleus. The normal G becomes oxoG, making it very slightly out of shape. There might be one in a million of these on a DNA strand. While it seems like a minor typo, it can actually cause the translation machinery to insert the wrong amino acid into a protein, with disastrous results, such as colorectal cancer. 12
The machine latches onto the DNA double helix and works its way down the strand, feeling every base on the way. As it proceeds, it kinks the DNA strand into a sharp angle. It is built to ignore the T and A bases, but whenever it feels a C, it knows there is supposed to be a G attached. The machine has precision contact points for C and G. When the C engages, the base paired to it is flipped up out of the helix into a slot inside the enzyme that is finely crafted to mate with a pure, clean G. If all is well, it flips the G back into the DNA helix and moves on. If the base is an oxoG, however, that base gets flipped into another slot further inside, where powerful forces yank the errant base out of the strand so that other machines can insert the correct one.
Now this is all wonderful stuff so far, but as with many things in living cells, the true wonder is in the details. The thermodynamic energy differences between G and oxoG are extremely slight – oxoG contains only one extra atom of oxygen – and yet this machine is able to discriminate between them to high levels of accuracy.
The author, David, says in the Nature article :
Structural biology: DNA search and rescue
DNA-repair enzymes amaze us with their ability to search through vast tracts of DNA to find subtle anomalies in the structure. The human repair enzyme 8-oxoguanine glycosylase (hOGG1) is particularly impressive in this regard because it efficiently removes 8-oxoguanine (oxoG), a damaged guanine (G) base containing an extra oxygen atom, and ignores undamaged bases.
The team led by Anirban Banerjee of Harvard, using a clever new stop-action method of imaging, caught this little enzyme in the act of binding to a bad guanine, helping scientists visualize how the machinery works. Some other amazing details are mentioned about this molecular proofreader. It checks every C-G pair, but slips right past the A-T pairs. The enzyme, “much like a train that stops only at certain locations,” pauses at each C and, better than any railcar conductor inspecting each ticket, flips up the G to validate it. Unless it conforms to the slot perfectly – even though G and oxoG differ in their match by only one hydrogen bond – it is ejected like a freeloader in a Pullman car and tossed out into the desert. David elaborates:
Calculations of differences in free energy indicate that both favourable and unfavourable interactions lead to preferential binding of oxoG over G in the oxoG-recognition pocket, and of G over oxoG in the alternative site. This structure [the image resolved by the scientific team] captures an intermediate that forms in the process of finding oxoG, and illustrates that the damaged base must pass through a series of ‘gates’, or checkpoints, within the enzyme; only oxoG satisfies the requirements for admission to the damage-specific pocket, where it will be clipped from the DNA. Other bases (C, A and T) may be rejected outright without extrusion from the helix because hOGG1 scrutinizes both bases in each pair, and only bases opposite a C will be examined more closely.
Natural selection cannot act without accurate replication, yet the protein machinery for the level of accuracy required is itself built by the very genetic code it is designed to protect. Thats a catch22 situation. It would have been challenging enough to explain accurate transcription and translation alone by natural means, but as consequence of UV radiation, it would have quickly been destroyed through accumulation of errors. So accurate replication and proofreading are required for the origin of life. How on earth could proofreading enzymes emerge, especially with this degree of fidelity, when they depend on the very information that they are designed to protect? Think about it.... This is one more prima facie example of chicken and egg situation. What is the alternative explanation to design ? Proofreading DNA by chance ? And a complex suite of translation machinery without a designer?
I enjoy to learn about the wonder of these incredible mechanisms. If the apostle Paul could understand that creation demands a Creator as he wrote in Romans chapter one 18, how much more we today with all the revelations about cell biology and molecular machines?
Since the editing machinery itself requires proper proofreading and editing during its manufacturing, how would the information for the machinery be transmitted accurately before the machinery was in place and working properly? Lest it be argued that the accuracy could be achieved stepwise through selection, note that a high degree of accuracy is needed to prevent ‘error catastrophe’ in the first place—from the accumulation of ‘noise’ in the form of junk proteins specified by the damaged DNA. 18
Depending on the species, this repair system can eliminate abnormal bases such as Uracil; Thymine dimers 3-methyladenine; 7-methylguanine

14
Since many mutations are deleterious, DNA repair systems are vital to the survival of all organisms
Living cells contain several DNA repair systems that can fix different type of DNA alterations
Nucleotide excision repair (NER)
Nucleotide excision repair is a DNA repair mechanism. DNA damage occurs constantly because of chemicals (i.e. intercalating agents), radiation and other mutagens.
Nucleotide excision repair (NER) is a highly conserved DNA repair mechanism. NER systems recognize the damaged DNA strand, cleave it on both sides of the lesion, remove and newly synthesize the fragment. UvrB is a central component of the bacterial NER system participating in damage recognition, strand excision and repair synthesis.[/b] We have solved the crystal structure of UvrB in the apo and the ATP-bound forms. UvrB contains two domains related in structure to helicases, and two additional domains unique to repair proteins. The structure contains all elements of an intact helicase, and is evidence that UvrB utilizes ATP hydrolysis to move along the DNA to probe for damage. The location of conserved residues and structural comparisons allow us to predict the path of the DNA and suggest that the tight preincision complex of UvrB and the damaged DNA is formed by insertion of a flexible β-hairpin between the two DNA strands. 3
DNA constantly requires repair due to damage that can occur to bases from a vast variety of sources including chemicals but also ultraviolet (UV) light from the sun. Nucleotide excision repair (NER) is a particularly important mechanism by which the cell can prevent unwanted mutations by removing the vast majority of UV-induced DNA damage (mostly in the form of thymine dimers and 6-4-photoproducts). The importance of this repair mechanism is evidenced by the severe human diseases that result from in-born genetic mutations of NER proteins including Xeroderma pigmentosum and Cockayne's syndrome. While the base excision repair machinery can recognize specific lesions in the DNA and can correct only damaged bases that can be removed by a specific glycosylase, the nucleotide excision repair enzymes recognize bulky distortions in the shape of the DNA double helix. Recognition of these distortions leads to the removal of a short single-stranded DNA segment that includes the lesion, creating a single-strand gap in the DNA, which is subsequently filled in by DNA polymerase, which uses the undamaged strand as a template. NER can be divided into two subpathways (Global genomic NER and Transcription coupled NER) that differ only in their recognition of helix-distorting DNA damage. 4
Nucleotide excision repair (NER) is a particularly important excision mechanism that removes DNA damage induced by ultraviolet light (UV). 2UV DNA damage results in bulky DNA adducts - these adducts are mostly thymine dimers and 6,4-photoproducts. Recognition of the damage leads to removal of a short single-stranded DNA segment that contains the lesion. The undamaged single-stranded DNA remains and DNA polymerase uses it as a template to synthesize a short complementary sequence. Final ligation to complete NER and form a double stranded DNA is carried out by DNA ligase. NER can be divided into two subpathways: global genomic NER (GG-NER) and transcription coupled NER (TC-NER). The two subpathways differ in how they recognize DNA damage but they share the same process for lesion incision, repair, and ligation.
The importance of NER is evidenced by the severe human diseases that result from in-born genetic mutations of NER proteins. Xeroderma pigmentosum and Cockayne's syndrome are two examples of NER associated diseases.
Maintaining genomic integrity is essential for living organisms. NER is a major pathway allowing the removal of lesions which would otherwise accumulate and endanger the health of the affected organism. 5
Nucleotide excision repair (NER) is a mechanism to recognize and repair bulky DNA damage caused by compounds, environmental carcinogens, and exposure to UV-light. In humans hereditary defects in the NER pathway are linked to at least three diseases: xeroderma pigmentosum (XP), Cockayne syndrome (CS), and trichothiodystrophy (TTD). The repair of damaged DNA involves at least 30 polypeptides within two different sub-pathways of NER known as transcription-coupled repair (TCR-NER) and global genome repair (GGR-NER). TCR refers to the expedited repair of lesions located in the actively transcribed strand of genes by RNA polymerase II (RNAP II). In GGR-NER the first step of damage recognition involves XPC-hHR23B complex together with XPE complex (in prokaryotes, uvrAB complex). The following steps of GGR-NER and TCR-NER are similar.

Paulina Prorok Evolutionary Origins of DNA Repair Pathways: Role of Oxygen Catastrophe in the Emergence of DNA Glycosylases 2021 Jun 24
https://www.ncbi.nlm.nih.gov/pmc/articles/PMC8307549/
1) http://www.genome.jp/dbget-bin/www_bget?ko03420
2) http://en.wikipedia.org/wiki/Nucleotide_excision_repair
3) http://www.ncbi.nlm.nih.gov/pmc/articles/PMC1171753/pdf/006899.pdf
4) http://bioisolutions.blogspot.com.br/2008/04/ner-pathway.html
5) http://intelligent-sequences.blogspot.com.br/2008_06_01_archive.html
6) https://en.wikipedia.org/wiki/Molecular_lesion
8 ) https://en.wikipedia.org/wiki/DNA_glycosylase
9) http://www.csun.edu/~cmalone/pdf360/Ch15-2repairtanspose.pdf
10) http://www.nature.com/nature/journal/v434/n7033/full/nature03458.html
11) http://www.nature.com/nature/journal/v434/n7033/full/nature03458.html
12) http://creationsafaris.com/crev200503.htm
13) http://fire.biol.wwu.edu/trent/trent/DNAsearchrescue.pdf
14) http://www.genome.jp/kegg-bin/show_pathway?ko03410
15) https://en.wikipedia.org/wiki/DNA_damage_(naturally_occurring)
16) http://www.intechopen.com/books/new-research-directions-in-dna-repair/dna-damage-dna-repair-and-cancer
17) http://creation.com/dna-best-information-storage
18) http://nrc58.nas.edu/RAPLab10/Opportunity/Opportunity.aspx?LabCode=50&ROPCD=506451&RONum=B7083
19) http://www.ladydavis.ca/en/DNAdamage?mid=ctl00_MainMenu_ctl00_TheMenu-menuItem002-subMenu-menuItem001
20) http://truemedmd.com/wp-content/uploads/2015/01/Shapiro.1997.BostonReview1997.ThirdWay.pdf
Last edited by Otangelo on Mon Oct 31, 2022 9:21 am; edited 107 times in total