14. Cytoskeletal Arrays
Cytoskeletal arrays are dynamic networks of protein filaments within cells that play a fundamental role in providing structural support, cellular movement, and intracellular transport. These arrays consist primarily of three types of protein filaments: actin filaments (microfilaments), microtubules, and intermediate filaments. Each type serves specific functions and contributes to various cellular processes, including the development of organismal form and function.
Overview and Importance
Actin Filaments (Microfilaments): Actin filaments are thin protein fibers that form a flexible network. They are involved in cellular processes such as cell shape maintenance, cell migration, muscle contraction, and the formation of the contractile ring during cytokinesis. Actin arrays provide mechanical strength to cells and contribute to changes in cell shape and motility.
Microtubules: Microtubules are hollow tubes made of tubulin protein subunits. They serve as tracks for intracellular transport, including the movement of organelles and vesicles. Microtubules also play a key role in cell division, as they form the mitotic spindle that segregates chromosomes during mitosis and meiosis.
Intermediate Filaments: Intermediate filaments provide structural stability to cells and tissues. They are particularly important in cells subjected to mechanical stress, such as epithelial cells and neurons. Intermediate filaments contribute to maintaining cell shape, anchoring organelles, and transmitting forces within tissues.
Importance in Biological Systems
Cytoskeletal arrays are crucial in various biological systems due to their multifaceted roles:
Cellular Shape and Support: Cytoskeletal elements provide the framework for maintaining cell shape and integrity. They also help cells resist mechanical stress and deformation.
Intracellular Transport: Microtubules act as tracks for motor proteins to move cellular cargo, including organelles and vesicles, within the cell. This transport is essential for proper cellular functioning.
Cell Movement: Actin filaments power cellular movements, such as the extension of pseudopods in amoeboid motion and the formation of filopodia and lamellipodia in cell migration.
Cell Division: Both microtubules and actin filaments are crucial for cell division. Microtubules form the mitotic spindle, ensuring proper chromosome segregation, while actin filaments contribute to the formation of the contractile ring during cytokinesis.
Developmental Processes and Organismal Form and Function
Cytoskeletal arrays are central to the shaping of organismal form and function during development:
Embryogenesis: Cytoskeletal rearrangements play a pivotal role in embryonic development. They drive processes like cell migration, tissue organization, and the formation of complex structures.
Tissue Differentiation: The establishment of tissue-specific cytoskeletal arrays contributes to tissue differentiation. For instance, muscle cells rely on actin and myosin arrays for contraction, while neurons require microtubules for axonal growth.
Organ Formation: Cytoskeletal dynamics guide the folding and shaping of organs during development. For example, actin-based contractions influence the formation of the heart and other organs.
Cytoskeletal arrays are essential components in cells, contributing to diverse biological functions ranging from maintaining cell shape to guiding intracellular transport and enabling cell movement. Their role in development shapes the form and function of organisms by orchestrating processes critical to tissue differentiation, organ formation, and overall cellular dynamics.
How do cytoskeletal arrays contribute to cell shape, movement, and tissue morphogenesis?
Cytoskeletal arrays play integral roles in determining cell shape, facilitating cellular movement, and orchestrating tissue morphogenesis. These dynamic protein networks—comprising actin filaments, microtubules, and intermediate filaments—underpin numerous cellular processes that collectively contribute to the development and maintenance of cell shape, movement, and tissue structure.
Contribution to Cell Shape
Cytoskeletal arrays are vital for defining and maintaining cell shape in several ways:
Actin Filaments: Actin filaments, forming the actin cytoskeleton, provide structural support and allow cells to adopt various shapes. The assembly and disassembly of actin filaments dynamically regulate cell morphology, enabling processes like cell spreading, contraction, and the formation of cellular protrusions (such as filopodia and lamellipodia).
Intermediate Filaments: Intermediate filaments provide mechanical stability to cells and tissues. They help maintain cell shape in cells exposed to mechanical stress, such as epithelial cells. Different types of intermediate filaments are found in specific cell types, contributing to their distinct shapes.
Facilitating Cellular Movement
Cytoskeletal arrays are instrumental in cellular movement, aiding in both locomotion and intracellular transport:
Actin-Based Movement: Actin filaments enable cell motility through the formation of specialized structures like lamellipodia and filopodia. These structures extend from the cell's leading edge and interact with the extracellular environment, facilitating cell migration by propelling the cell forward.
Microtubule-Based Transport: Microtubules serve as tracks for motor proteins that transport cellular cargo. Kinesins and dyneins, motor proteins that move along microtubules, transport organelles, vesicles, and other cellular components within the cell.
Tissue Morphogenesis
Cytoskeletal arrays are pivotal during tissue morphogenesis, guiding the formation and organization of complex multicellular structures:
Cell Sorting and Migration: During tissue development, cells undergo rearrangements, sorting, and migration. Cytoskeletal elements, particularly actin filaments, are involved in driving these processes. For example, actin-based structures help cells move collectively to specific regions, contributing to tissue organization.
Tissue Folding and Remodeling: The controlled assembly and disassembly of cytoskeletal arrays influence tissue folding, shaping, and remodeling. Changes in the actin cytoskeleton can lead to invaginations that give rise to complex structures like the brain's sulci and gyri.
Organogenesis: Cytoskeletal dynamics guide the generation and positioning of organs. For instance, the formation of the heart involves coordinated cell movements driven by the actin cytoskeleton.
Cytoskeletal arrays are central to cell shape determination, cellular movement, and tissue morphogenesis. These arrays enable cells to adapt to their environments, navigate through tissues, and contribute to the intricate processes involved in embryonic development, tissue differentiation, and organ formation. The orchestrated interactions within and between these arrays drive the dynamic changes necessary for the proper function and structure of cells and tissues in complex organisms.
What are the roles of microtubules, actin filaments, and intermediate filaments in cellular processes during development?
Microtubules, actin filaments, and intermediate filaments are key components of the cytoskeletal system, each playing distinct roles in various cellular processes during development. Here's how they contribute to essential developmental processes:
Microtubules
Cell Division and Mitosis: Microtubules form the mitotic spindle, a structure essential for proper chromosome segregation during cell division. They ensure that each daughter cell receives the correct number of chromosomes.
Cell Migration and Morphogenesis: Microtubules guide cell migration by providing tracks for motor proteins like kinesins and dyneins. They help cells move to their designated locations during tissue morphogenesis, such as neuron migration in the developing brain.
Intracellular Transport: Microtubules serve as tracks for motor proteins to transport cellular cargo along their length. This transport is crucial for delivering organelles and molecules to specific cellular locations.
Axon Guidance and Neuronal Development: Microtubules influence the growth and guidance of axons and dendrites in neurons. They guide the extension and branching of neuronal processes to establish proper neuronal connections.
Actin Filaments
Cell Shape and Motility: Actin filaments determine cell shape and support cell motility. They create protrusive structures like lamellipodia and filopodia, which cells use for adhesion, locomotion, and interaction with the extracellular environment.
Cell Migration and Wound Healing: Actin filaments drive cell migration during development and wound healing. They enable cells to contract and exert forces, which are essential for cell movement and closing gaps in tissues.
Cytokinesis: Actin filaments participate in the formation of the contractile ring during cytokinesis, aiding in the physical separation of daughter cells.
Endocytosis and Exocytosis: Actin filaments play roles in membrane dynamics, facilitating processes like endocytosis (cellular intake of materials) and exocytosis (release of cellular products).
Intermediate Filaments
Mechanical Support: Intermediate filaments provide mechanical stability to cells and tissues exposed to mechanical stress. They help maintain cell integrity and tissue structure, especially in epithelial and connective tissues.
Tissue Integrity and Organ Formation: Intermediate filaments contribute to tissue integrity and are critical during tissue morphogenesis. They aid in the shaping and positioning of cells and tissues during organogenesis.
Nuclear Positioning and Anchoring: Intermediate filaments help anchor the nucleus within the cell, influencing cell shape and polarity. This anchoring is important for maintaining proper nuclear positioning and cellular organization.
Cell-Cell Adhesion: Intermediate filaments interact with cell-cell adhesion molecules, contributing to the mechanical strength of tissues and assisting in cell-cell communication.
Microtubules, actin filaments, and intermediate filaments are essential components of the cytoskeletal system with distinct roles in cellular processes during development. They collectively enable processes such as cell division, migration, morphogenesis, tissue integrity, and organ formation, ensuring proper growth, differentiation, and organization of tissues and organs in developing organisms.
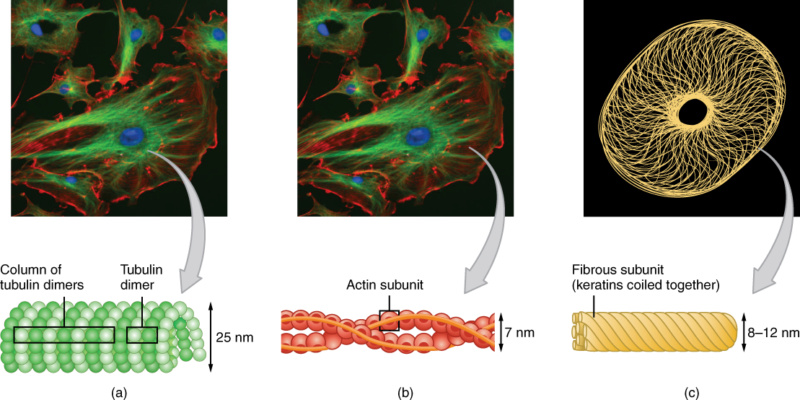
The cytoskeleton consists of (a) microtubules, (b) microfilaments, and (c) intermediate filaments. 1
Appearance of cytoskeletal arrays in the evolutionary timeline
The appearance of cytoskeletal arrays in the evolutionary timeline is hypothesized based on a combination of molecular and cellular evidence, comparative genomics, and the study of extant organisms. While the exact timeline is subject to ongoing research and investigation, here's a generalized outline of the hypothesized appearance of cytoskeletal arrays:
Prokaryotic Origins: Early prokaryotic cells lacked distinct cytoskeletal structures. Simple filamentous proteins would have provided rudimentary structural support and contributed to cell shape.
Eukaryotic Evolution: The emergence of eukaryotic cells would have brought about the development of more complex cellular organization. Microtubule-like proteins would have evolved, possibly serving functions in intracellular transport. Actin-like proteins would have emerged, contributing to cell shape and rudimentary motility.
Early Multicellularity: The transition to multicellularity required improved cell-cell adhesion and communication. Cytoskeletal elements would have begun to play roles in maintaining tissue integrity and supporting multicellular organization.
Diversification of Lineages: Different lineages of organisms would have developed unique adaptations of cytoskeletal arrays to suit their ecological niches. Intermediate filaments would have evolved in animals to provide mechanical stability in tissues subjected to stress.
Emergence of Complex Animals and Plants: Actin filaments and microtubules would have become more specialized, playing critical roles in cell division, cellular movement, and tissue morphogenesis. More sophisticated motor proteins would have evolved, enabling efficient intracellular transport along cytoskeletal tracks.
Modern Eukaryotes: Today's eukaryotic cells possess intricate cytoskeletal networks composed of microtubules, actin filaments, and intermediate filaments. These networks underlie essential cellular processes, including cell division, movement, structural support, and intracellular transport. It's important to emphasize that the evolutionary timeline of cytoskeletal array development is an area of active research, and the details of when specific cytoskeletal components emerged and diversified are still being explored.
De Novo Genetic Information necessary to instantiate cytoskeletal arrays
Creating the mechanisms of cytoskeletal arrays de novo would involve introducing new genetic information in a coordinated sequence to existing genetic material. This hypothetical process would necessitate the origination of various functional components from scratch, each contributing to the formation and regulation of cytoskeletal arrays:
Origination of Protein-Coding Sequences: New genes encoding cytoskeletal proteins, such as tubulin for microtubules and actin for actin filaments, would need to originate. These sequences would contain the necessary instructions for protein synthesis.
Promoters and Enhancers: Regulatory elements like promoters and enhancers would have to arise to control the expression of the newly originated genes. These sequences would determine when and where the genes are transcribed.
Transcription Factors: Transcription factors, which bind to specific regulatory sequences, would need to emerge. These factors would play a role in initiating gene transcription, ensuring proper temporal and spatial expression.
Translation Machinery: The machinery required for translation, including ribosomes and tRNA molecules, would need to be present to decode genetic information and synthesize proteins.
Post-Translational Modifications: Mechanisms for post-translational modifications, such as phosphorylation or acetylation, would need to originate. These modifications fine-tune the activity and function of cytoskeletal proteins.
Motor Proteins and Intracellular Transport: If motor proteins like kinesins and dyneins are considered, the genes encoding these proteins would need to originate. Additionally, regulatory elements and protein-interaction domains would need to evolve to ensure proper transport along microtubules.
Intermediate Filament Proteins: For intermediate filaments, novel genes encoding intermediate filament proteins would have to emerge. These proteins would need specific domains for filament formation and interactions.
Signal Transduction Pathways: New genes and regulatory elements for signaling pathways that regulate cytoskeletal dynamics would need to arise. These pathways control processes like cell migration, division, and differentiation.
Protein-Protein Interactions: Protein domains and motifs that enable protein-protein interactions within cytoskeletal networks would need to evolve. These interactions are crucial for forming functional arrays.
Cellular Localization Sequences: Sequences that direct newly synthesized proteins to their correct subcellular locations would have to emerge. This ensures proper incorporation of cytoskeletal components into the cytoplasm.
Epigenetic Regulation: Epigenetic mechanisms, such as DNA methylation and histone modifications, would need to originate to control gene expression and maintain stable cytoskeletal structures.
Spatial and Temporal Coordination: Mechanisms for coordinating the spatial and temporal assembly of cytoskeletal arrays would have to evolve. This coordination ensures that arrays form in the right place and at the right time.
Regulatory Feedback Loops: Regulatory circuits that respond to cellular cues and feedback loops would need to arise. These mechanisms adjust cytoskeletal dynamics in response to environmental changes.
In this hypothetical scenario, the origin of new genetic information in a precise sequence is crucial for creating the mechanisms required for cytoskeletal arrays. This includes the origination of genes, regulatory elements, protein domains, and intricate cellular processes that collectively contribute to the assembly, organization, and functioning of cytoskeletal arrays.
Manufacturing codes and languages that would have to emerge and be employed to instantiate cytoskeletal arrays
Creating a fully developed cytoskeletal array in an organism would require the establishment of intricate manufacturing codes and languages beyond the genetic information systems. These codes and languages involve various non-genetic factors that contribute to the assembly, organization, and regulation of cytoskeletal arrays:
Protein Folding Codes: The formation of functional cytoskeletal proteins requires precise folding codes. Chaperones and folding factors would need to be created to ensure newly synthesized proteins fold correctly.
Post-Translational Modification Codes: Codes for post-translational modifications, such as phosphorylation, acetylation, and ubiquitination, would need to emerge. Enzymes that catalyze these modifications would ensure proper protein function and interaction within cytoskeletal arrays.
Subcellular Localization Signals: Sequences directing proteins to specific subcellular locations, such as the plasma membrane or the nucleus, would need to be established to ensure correct protein incorporation into the cytoskeletal array.
Assembly and Disassembly Codes: Mechanisms for the assembly and disassembly of cytoskeletal arrays would have to be instantiated. These codes would guide the timing and coordination of filament formation and disintegration.
Cytoskeletal Crosslinking Codes: Codes for proteins that crosslink cytoskeletal filaments together would need to evolve. These crosslinking proteins play roles in organizing and stabilizing the array's structure.
Motor Protein Interaction Codes: Codes for motor proteins like kinesins and dyneins, involved in intracellular transport along cytoskeletal tracks, would need to emerge. These codes ensure specific interactions with filaments and cargo.
Cell Signaling Codes: Codes for signaling molecules that communicate the need for cytoskeletal rearrangements would have to be established. These codes would transmit cues related to cell migration, differentiation, and response to extracellular stimuli.
Cell Adhesion Codes: Codes for cell adhesion molecules and their interactions with the cytoskeleton would need to be introduced. These codes contribute to cell-substrate and cell-cell interactions during cytoskeletal assembly.
Feedback Loop Codes: Codes for feedback loops that sense cytoskeletal dynamics and adjust them in response to cellular needs would need to evolve. These loops maintain array stability and adapt to changing conditions.
Temporal and Spatial Patterning Codes: Codes for coordinating the temporal and spatial organization of cytoskeletal arrays during development would have to arise. These codes ensure arrays form in the correct locations and times.
Epigenetic Regulation Codes: Codes for epigenetic marks that influence the expression of genes related to cytoskeletal components would need to be created. These codes control gene accessibility and expression.
In this scenario, the emergence of fully developed cytoskeletal arrays would require the instantiation of a complex network of manufacturing codes and languages beyond genetic information. These codes would govern protein folding, modifications, interactions, localization, and coordination, enabling the assembly and functioning of the intricate cytoskeletal arrays.
Epigenetic Regulatory Mechanisms necessary to be instantiated for cytoskeletal arrays
The hypothetical creation of epigenetic regulation to develop cytoskeletal arrays from scratch would involve intricate coordination between various systems. While this scenario is speculative, the following are some potential mechanisms that would need to be established:
DNA Methylation: DNA methylation patterns could be established to regulate the expression of genes encoding cytoskeletal components. Methylation of promoter regions could silence or activate these genes.
Histone Modifications: Specific histone modifications, such as acetylation and methylation, would need to be introduced to influence chromatin structure and gene accessibility.
Histone marks associated with active or repressive chromatin states could determine the expression levels of genes related to cytoskeletal proteins.
Non-Coding RNAs: Non-coding RNAs, such as microRNAs and long non-coding RNAs, would need to originate and target mRNAs encoding cytoskeletal proteins, affecting their translation and stability.
Chromatin Remodeling Complexes: Chromatin remodeling complexes could evolve to alter chromatin structure and accessibility, allowing for precise regulation of gene expression during cytoskeletal development.
Epigenetic Inheritance Systems: Systems for epigenetic inheritance would need to be established to ensure the transmission of epigenetic information from one generation to the next. This might involve mechanisms similar to DNA methylation or histone modifications.
Collaboration with Signaling Pathways: Epigenetic regulation would likely collaborate with signaling pathways that respond to extracellular cues. This cooperation would enable the integration of environmental signals into the development of cytoskeletal arrays.
Transcription Factors and Enhancers: Transcription factors and enhancers would need to evolve to precisely control the expression of genes encoding cytoskeletal components.
These factors and enhancers would collaborate with epigenetic marks to fine-tune gene expression patterns.
Cell Cycle Control: Proper coordination with the cell cycle control machinery would be essential to ensure that epigenetic modifications are established and maintained during different phases of cell division and development.
Protein-Protein Interactions: Protein complexes involved in epigenetic regulation would need to interact with other cellular components, including those involved in chromatin structure and gene expression.
Metabolic Pathways: Metabolic pathways might influence epigenetic modifications through the availability of cofactors and substrates required for enzymatic activities.
Feedback Mechanisms: Feedback loops could evolve to maintain epigenetic stability and adapt the epigenome to changing cellular conditions.
In this hypothetical scenario, the creation of epigenetic regulation for cytoskeletal array development would necessitate the establishment of various systems working in concert. These systems would collaborate to ensure the proper establishment, maintenance, and inheritance of epigenetic marks, ultimately influencing the expression of genes related to cytoskeletal components and their assembly into functional arrays.
Signaling Pathways necessary to create, and maintain cytoskeletal arrays
The hypothetical emergence of cytoskeletal arrays from scratch would involve the creation and orchestration of intricate signaling pathways that guide their formation and regulation. While this scenario is speculative, the following are some potential signaling pathways and their interconnectedness:
Wnt Signaling Pathway: The Wnt pathway could be involved in cytoskeletal array development, influencing cell polarity, migration, and tissue morphogenesis.
Wnt signaling crosstalks with pathways like the Rho GTPase pathway to regulate actin dynamics and cytoskeletal rearrangements.
Notch Signaling Pathway: Notch signaling might play a role in cell fate determination and differentiation during cytoskeletal array development.
Cross-talk with other pathways like the Hedgehog pathway could fine-tune cellular responses.
Hedgehog Signaling Pathway: The Hedgehog pathway could contribute to tissue patterning and differentiation, impacting cytoskeletal organization.
Interaction with pathways like the MAPK pathway could influence cytoskeletal rearrangements during cell migration and morphogenesis.
MAPK/ERK Signaling Pathway: The MAPK/ERK pathway could regulate gene expression patterns related to cytoskeletal components and control cellular processes like migration and proliferation.
Crosstalk with the PI3K/AKT pathway could affect actin dynamics and cell motility.
PI3K/AKT Signaling Pathway: The PI3K/AKT pathway might influence cell growth, motility, and survival, which in turn could impact cytoskeletal organization.
Interaction with the mTOR pathway could coordinate cytoskeletal changes with cellular energy status.
TGF-β Signaling Pathway: The TGF-β pathway could contribute to tissue development and morphogenesis by regulating cell differentiation and migration.
Crosstalk with pathways like the Rho GTPase pathway could influence actin dynamics and cytoskeletal reorganization.
Cell Adhesion Signaling: Cell adhesion molecules, such as integrins, could initiate signaling cascades that guide cytoskeletal organization during adhesion, migration, and tissue development.
These pathways could crosstalk with growth factor pathways to integrate extracellular signals.
Cell Cycle Checkpoints and p53 Signaling: Signaling pathways involved in cell cycle checkpoints and DNA damage response might regulate cytoskeletal dynamics to ensure proper cell division and repair.
Crosstalk with growth factor pathways could link cellular growth and division to cytoskeletal changes.
Feedback Loops and Integration: These pathways could form complex feedback loops to maintain homeostasis and adjust cytoskeletal dynamics in response to changing conditions.
Integration with metabolic pathways would connect cellular energy status with cytoskeletal remodeling.
In this speculative scenario, the emergence of cytoskeletal arrays would involve a network of interconnected signaling pathways. These pathways would communicate, crosstalk, and coordinate with each other to ensure proper cytoskeletal organization, cell movement, tissue morphogenesis, and overall developmental processes. Additionally, these pathways would eventually intersect with broader biological systems, such as those governing cell cycle progression, differentiation, and response to environmental cues.
Regulatory codes necessary for maintenance and operation of cytoskeletal arrays
The maintenance and operation of cytoskeletal arrays would necessitate the establishment of regulatory codes and languages that orchestrate their dynamic functions and stability:
Dynamic Remodeling Codes: Codes that initiate dynamic remodeling of cytoskeletal arrays would need to be created. These codes trigger changes in array organization during processes like cell migration, division, and tissue morphogenesis.
Feedback Loop Codes: Feedback loop codes would be essential to sense changes in cellular conditions and adjust cytoskeletal dynamics accordingly. These codes maintain homeostasis and adapt arrays to varying demands.
Stabilization Codes: Codes for stabilizing cytoskeletal arrays, especially during mechanical stress, would need to emerge. These codes prevent excessive disassembly and ensure structural integrity.
Cellular Adhesion Codes: Regulatory codes for cell adhesion molecules would be required. These codes would coordinate adhesion with cytoskeletal dynamics, influencing cell migration and tissue organization.
Motor Protein Regulation Codes: Codes for regulating motor protein activity and interactions with cytoskeletal filaments would be essential. These codes ensure proper intracellular transport and cargo delivery.
Signaling Integration Codes: Regulatory codes that integrate signals from different pathways into cytoskeletal regulation would need to be instantiated. These codes enable the coordination of various cellular processes.
Cell Cycle Coordination Codes: Codes that link cytoskeletal dynamics to the cell cycle would be crucial. These codes ensure proper cytoskeletal organization during cell division and cell cycle progression.
Differentiation and Development Codes: Codes involved in cell differentiation and development would regulate cytoskeletal changes specific to different cell types. These codes contribute to tissue morphogenesis.
Response to Environmental Codes: Codes that allow the cytoskeletal arrays to respond to environmental cues would need to evolve. These codes adapt arrays to changes in the extracellular environment.
Adaptation Codes: Regulatory codes that enable cytoskeletal arrays to adapt to cellular growth and changes in cell size would need to be established. These codes maintain proportionality.
Epigenetic Maintenance Codes: Codes for maintaining epigenetic marks related to cytoskeletal components would be required. These codes ensure stable gene expression patterns over time.
Cross-Talk and Integration Codes: Regulatory codes that facilitate cross-talk and integration between different cytoskeletal components and pathways would need to be instantiated. These codes ensure coordinated functioning.
In this scenario, a complex array of regulatory codes and languages would need to be instantiated to ensure the maintenance, stability, and proper operation of cytoskeletal arrays. These codes would enable precise control over array dynamics, integration with cellular processes, and adaptation to changing conditions.
How would the evolution of cytoskeletal arrays enable the development of diverse cell shapes and functions?
The evolution of cytoskeletal arrays has played a fundamental role in enabling the development of diverse cell shapes and functions across different organisms. Cytoskeletal arrays provide structural support, facilitate intracellular transport, and drive cellular movements, all of which contribute to the formation of distinct cell shapes and the execution of specialized functions. Here's how the evolution of cytoskeletal arrays enables this diversity:
Structural Support and Shape Determination: Cytoskeletal arrays, particularly microtubules and actin filaments, help define and maintain cell shape by providing a structural framework.
The arrangement and organization of cytoskeletal filaments contribute to the development of specific cell shapes. For instance, neuron morphology is influenced by the organization of microtubules and actin filaments.
Cell Polarization and Asymmetry: Cytoskeletal arrays contribute to cell polarization, where different regions of a cell exhibit distinct structures and functions.
Asymmetrical distribution of cytoskeletal components allows cells to establish polarity, which is crucial for processes like cell migration, neuronal development, and tissue morphogenesis.
Cell Migration and Motility: The dynamic rearrangement of actin filaments and microtubules enables cell migration and movement. Lamellipodia and filopodia formation, driven by actin polymerization, are key to cell motility.
Specialized cytoskeletal arrays within cells, such as the leading edge of migrating cells, allow them to move to specific locations within tissues.
Intracellular Transport: Microtubules provide tracks for motor proteins like kinesins and dyneins, enabling the transport of organelles, vesicles, and other cargo within cells.
Intracellular transport facilitated by cytoskeletal arrays allows cells to distribute resources, maintain organelle function, and establish cellular compartments.
Cell Division and Growth: Cytoskeletal arrays are essential for proper cell division. Microtubules form the mitotic spindle, ensuring accurate chromosome segregation, while actin filaments contribute to cytokinesis.
These processes influence cell growth, proliferation, and tissue development.
Cell-Cell Interactions and Tissue Morphogenesis: Cytoskeletal arrays are involved in cell-cell interactions, cell adhesion, and tissue morphogenesis.
Cell adhesion molecules, interacting with cytoskeletal components, facilitate the formation of tissues with specific structures and functions.
Specialized Functions: Different cell types have evolved specialized cytoskeletal arrays that enable unique functions. For example, the flagellar and ciliary arrays allow cells to move through fluid environments, as seen in sperm cells and ciliated epithelial cells.
Response to Environmental Cues: Cytoskeletal arrays enable cells to respond to external signals and adapt their shape and behavior accordingly. This is crucial for processes like wound healing, immune responses, and sensory functions.
Overall, the evolution of cytoskeletal arrays has enabled cells to develop diverse shapes and functions by providing them with the mechanical and dynamic capabilities needed to interact with their environment, move, divide, and perform specialized tasks. The versatile nature of cytoskeletal arrays has been a driving force in the adaptation and diversification of life forms on Earth.
Is there scientific evidence supporting the idea that cytoskeletal arrays were brought about by the process of evolution?
The intricate nature of cytoskeletal arrays and their essential role in cellular functions presents significant challenges for their evolution through gradual, step-by-step processes. The simultaneous instantiation of various codes, languages, signaling pathways, and proteins necessary for the functioning of cytoskeletal arrays is a more plausible explanation than a stepwise evolutionary process. Here's why:
Functional Interdependence: Cytoskeletal arrays require the coordination of numerous components, including protein filaments, motor proteins, signaling pathways, and regulatory codes. Each component is interdependent with others, and their functions are tightly linked. It is implausible that these components would have evolved independently and later coincidentally converged to form functional arrays.
Lack of Intermediate Functionality: The stepwise evolution of cytoskeletal arrays would involve the gradual emergence of individual components and intermediate stages. However, these intermediate stages would likely lack functionality since the array's proper functioning depends on the precise assembly, regulation, and interactions of multiple components.
Irreducible Complexity: Cytoskeletal arrays are irreducibly complex systems where the removal of any essential component would result in the loss of function. For instance, without proper motor proteins, the transport of cellular cargo along filaments would be compromised, rendering the array non-functional.
Informational Complexity: The simultaneous establishment of codes, languages, and regulatory networks that govern the assembly and behavior of cytoskeletal components requires a high degree of informational complexity. This level of complexity challenges the idea that these systems could have gradually emerged through random mutations and natural selection.
Lack of Selective Advantage: During the hypothetical stepwise evolution of cytoskeletal arrays, intermediate stages lacking functionality would not offer a selective advantage to organisms. Natural selection typically favors traits that confer immediate benefits, making it difficult to envision how such non-functional intermediates would persist and accumulate in populations.
Coordination of Components: The coordinated operation of cytoskeletal arrays necessitates the existence of multiple components that interact precisely. The simultaneous emergence of these components through a stepwise process is highly unlikely, as the odds of random mutations leading to the correct sequence of events are exceedingly low.
Fine-Tuned Regulation: The regulation of cytoskeletal arrays involves intricate control mechanisms, including signaling pathways and epigenetic regulation. Such fine-tuned regulation requires these systems to be in place from the beginning to ensure proper functioning.
In summary, the complex and interdependent nature of cytoskeletal arrays, coupled with the lack of intermediate functionality and the informational complexity required, challenges the notion that these structures could have evolved step by step. The concept of intelligent design offers an alternative perspective that accounts for the simultaneous instantiation of all necessary components and systems, reflecting a purposeful and integrated approach to the origin of cytoskeletal arrays.
Irreducibility and Interdependence of the systems to instantiate and operate cytoskeletal arrays
The creation, development, and operation of cytoskeletal arrays involve a complex interplay of manufacturing, signaling, and regulatory codes and languages that are irreducible and interdependent. These systems collectively orchestrate the assembly, organization, and functioning of cytoskeletal arrays, and their simultaneous instantiation is more plausible than a stepwise evolutionary process. Here's how these components interact and why their interdependence challenges gradual evolution:
Manufacturing Codes and Post-Translational Modifications
Manufacturing codes guide the synthesis of cytoskeletal proteins, but their functionality often relies on post-translational modifications (PTMs) like phosphorylation, acetylation, and ubiquitination. PTMs fine-tune the properties of cytoskeletal components, affecting their interactions, stability, and function within the array. The absence of the manufacturing code would prevent the production of proteins, and the absence of PTMs would hinder their proper function.
Regulatory Codes and Epigenetic Regulation
Regulatory codes determine when and where cytoskeletal genes are expressed, while epigenetic regulation controls gene accessibility and expression over time. Epigenetic marks, such as DNA methylation and histone modifications, influence the expression of cytoskeletal genes and their responsiveness to cellular cues. Without regulatory codes, genes wouldn't be expressed appropriately, and without epigenetic regulation, stable gene expression patterns necessary for cytoskeletal assembly wouldn't be maintained.
Signaling Pathways and Protein Activation
Signaling pathways communicate extracellular cues to the cell, triggering responses that impact cytoskeletal arrays. Crosstalk between signaling pathways allows integration of multiple cues for coordinated cellular behavior. For example, growth factor signaling can influence cytoskeletal dynamics via pathways like MAPK/ERK and PI3K/AKT. If one signaling pathway were missing or disrupted, the cell's ability to receive and respond to signals influencing cytoskeletal organization would be compromised.
Feedback Loops and Adaptation
Feedback loops regulate cytoskeletal dynamics in response to changing conditions, ensuring stability and adaptation. These loops integrate information from various cellular processes, signaling pathways, and environmental cues to adjust cytoskeletal organization. Without functional feedback loops, cells would struggle to maintain the balance between stability and adaptability required for proper cytoskeletal function.
Motor Protein Interaction and Transport Codes
Motor protein codes and codes for cargo recognition ensure efficient intracellular transport along cytoskeletal tracks. These codes are intertwined with signaling pathways and cellular cues that determine when and where transport occurs. Without proper motor protein codes and the integration of transport with signaling, cellular cargo distribution would be impaired. The intricate interdependence of these codes and languages presents a significant challenge for the gradual evolution of cytoskeletal arrays. In a stepwise process, the absence or dysfunction of one system would disrupt the entire functional network. Since intermediate stages without complete systems would not offer a selective advantage, it's more reasonable to propose that these systems were instantiated together, fully operational, to enable the creation and function of cytoskeletal arrays. This perspective aligns with the concept of intelligent design, where the integrated complexity of cytoskeletal arrays suggests a purposeful and coordinated origin.
Once is instantiated and operational, what other intra and extracellular systems are Cytoskeletal Arrays interdependent with?
Once cytoskeletal arrays are instantiated and operational, they become interdependent with a wide range of intra and extracellular systems, reflecting their central role in various cellular processes. These interdependencies ensure proper cell functioning, structural integrity, and adaptation to the environment. Here are some of the key systems that cytoskeletal arrays are interdependent with:
Intracellular Systems
Cell Membrane and Receptor Systems: Cytoskeletal arrays interact with the cell membrane through adhesion molecules and receptor complexes. This interaction affects cell shape, adhesion, and signaling.
Endomembrane System: Vesicle trafficking and organelle movement within cells rely on cytoskeletal tracks and motor proteins. Proper function of the endomembrane system is intertwined with cytoskeletal dynamics.
Organelles and Subcellular Structures: Proper positioning and segregation of organelles during cell division and cellular activities are facilitated by cytoskeletal arrays. For instance, the mitotic spindle (made of microtubules) ensures accurate chromosome segregation.
Nucleus and Nuclear Pore Complexes: The nuclear envelope interacts with cytoskeletal elements during cell migration and division. Nuclear pore complexes enable molecular transport between the nucleus and cytoplasm, influenced by cytoskeletal dynamics.
Mitochondria and Energy Production: Mitochondria, responsible for energy production, require efficient transport along cytoskeletal tracks for proper distribution within the cell.
Extracellular Systems
Extracellular Matrix (ECM)
Cytoskeletal arrays interact with the ECM through integrin-mediated adhesion. This interaction influences cell migration, differentiation, and tissue organization.
Cell-Cell Junctions: Junctions like adherens junctions and desmosomes anchor cytoskeletal components to neighboring cells, contributing to tissue integrity and organization.
Cell-Cell Communication: Cytoskeletal arrays are essential for cellular movements that facilitate direct cell-cell communication, such as during immune responses and tissue development.
Extracellular Signaling Molecules: Extracellular signaling molecules, like growth factors and cytokines, can trigger cytoskeletal rearrangements that influence cell behavior and responses to the environment.
Mechanical Forces and Tissue Morphogenesis: Mechanical forces exerted by cytoskeletal arrays can shape tissues during development and contribute to wound healing and tissue remodeling.
Nervous System: In neurons, cytoskeletal arrays are critical for dendritic branching, axon guidance, and synapse formation. These processes underlie nervous system development and function.
The interdependence of cytoskeletal arrays with these intra and extracellular systems underscores their central role in cellular physiology and multicellular organismal development. The complexity and precision of these interrelationships raise questions about how such an integrated system could have emerged through gradual evolution, leading proponents of intelligent design to posit a purposeful and coordinated origin of these systems.
The interdependence of cytoskeletal arrays with various intra and extracellular systems reveals an intricate web of relationships that points toward a purposeful and coordinated design rather than a gradual evolutionary process. This can be highlighted through a syllogism:
1. Complex Interdependencies: Cytoskeletal arrays exhibit intricate interdependencies with multiple cellular and extracellular systems, including the cell membrane, endomembrane system, organelles, nucleus, mitochondria, extracellular matrix, cell-cell junctions, communication networks, mechanical forces, and the nervous system.
2. Interlocked Functions: These interdependencies are not just linear but form a highly interlocked network. The functioning of one system directly impacts others, and their coordinated operation is essential for cell shape, movement, division, tissue organization, and various specialized functions.
3. Absence of Selective Advantage in Intermediate Stages: The gradual evolution of these interconnected systems presents a challenge. In a stepwise scenario, intermediate stages lacking complete systems would not offer selective advantage, as isolated components would likely serve little or no purpose.
4. Simultaneous Emergence: The simultaneous emergence of these interdependent systems in a fully operational state is a more reasonable explanation. A purposeful and orchestrated design would ensure that all necessary components are instantiated together, allowing cells to function optimally from the outset.
Informational Complexity: The intricate interplay of codes, languages, signaling pathways, and protein interactions within and between these systems requires a high level of informational complexity. Such complexity suggests an intentional design rather than a gradual accumulation of random changes.
1. Cytoskeleton
Cytoskeletal arrays are dynamic networks of protein filaments within cells that play a fundamental role in providing structural support, cellular movement, and intracellular transport. These arrays consist primarily of three types of protein filaments: actin filaments (microfilaments), microtubules, and intermediate filaments. Each type serves specific functions and contributes to various cellular processes, including the development of organismal form and function.
Overview and Importance
Actin Filaments (Microfilaments): Actin filaments are thin protein fibers that form a flexible network. They are involved in cellular processes such as cell shape maintenance, cell migration, muscle contraction, and the formation of the contractile ring during cytokinesis. Actin arrays provide mechanical strength to cells and contribute to changes in cell shape and motility.
Microtubules: Microtubules are hollow tubes made of tubulin protein subunits. They serve as tracks for intracellular transport, including the movement of organelles and vesicles. Microtubules also play a key role in cell division, as they form the mitotic spindle that segregates chromosomes during mitosis and meiosis.
Intermediate Filaments: Intermediate filaments provide structural stability to cells and tissues. They are particularly important in cells subjected to mechanical stress, such as epithelial cells and neurons. Intermediate filaments contribute to maintaining cell shape, anchoring organelles, and transmitting forces within tissues.
Importance in Biological Systems
Cytoskeletal arrays are crucial in various biological systems due to their multifaceted roles:
Cellular Shape and Support: Cytoskeletal elements provide the framework for maintaining cell shape and integrity. They also help cells resist mechanical stress and deformation.
Intracellular Transport: Microtubules act as tracks for motor proteins to move cellular cargo, including organelles and vesicles, within the cell. This transport is essential for proper cellular functioning.
Cell Movement: Actin filaments power cellular movements, such as the extension of pseudopods in amoeboid motion and the formation of filopodia and lamellipodia in cell migration.
Cell Division: Both microtubules and actin filaments are crucial for cell division. Microtubules form the mitotic spindle, ensuring proper chromosome segregation, while actin filaments contribute to the formation of the contractile ring during cytokinesis.
Developmental Processes and Organismal Form and Function
Cytoskeletal arrays are central to the shaping of organismal form and function during development:
Embryogenesis: Cytoskeletal rearrangements play a pivotal role in embryonic development. They drive processes like cell migration, tissue organization, and the formation of complex structures.
Tissue Differentiation: The establishment of tissue-specific cytoskeletal arrays contributes to tissue differentiation. For instance, muscle cells rely on actin and myosin arrays for contraction, while neurons require microtubules for axonal growth.
Organ Formation: Cytoskeletal dynamics guide the folding and shaping of organs during development. For example, actin-based contractions influence the formation of the heart and other organs.
Cytoskeletal arrays are essential components in cells, contributing to diverse biological functions ranging from maintaining cell shape to guiding intracellular transport and enabling cell movement. Their role in development shapes the form and function of organisms by orchestrating processes critical to tissue differentiation, organ formation, and overall cellular dynamics.
How do cytoskeletal arrays contribute to cell shape, movement, and tissue morphogenesis?
Cytoskeletal arrays play integral roles in determining cell shape, facilitating cellular movement, and orchestrating tissue morphogenesis. These dynamic protein networks—comprising actin filaments, microtubules, and intermediate filaments—underpin numerous cellular processes that collectively contribute to the development and maintenance of cell shape, movement, and tissue structure.
Contribution to Cell Shape
Cytoskeletal arrays are vital for defining and maintaining cell shape in several ways:
Actin Filaments: Actin filaments, forming the actin cytoskeleton, provide structural support and allow cells to adopt various shapes. The assembly and disassembly of actin filaments dynamically regulate cell morphology, enabling processes like cell spreading, contraction, and the formation of cellular protrusions (such as filopodia and lamellipodia).
Intermediate Filaments: Intermediate filaments provide mechanical stability to cells and tissues. They help maintain cell shape in cells exposed to mechanical stress, such as epithelial cells. Different types of intermediate filaments are found in specific cell types, contributing to their distinct shapes.
Facilitating Cellular Movement
Cytoskeletal arrays are instrumental in cellular movement, aiding in both locomotion and intracellular transport:
Actin-Based Movement: Actin filaments enable cell motility through the formation of specialized structures like lamellipodia and filopodia. These structures extend from the cell's leading edge and interact with the extracellular environment, facilitating cell migration by propelling the cell forward.
Microtubule-Based Transport: Microtubules serve as tracks for motor proteins that transport cellular cargo. Kinesins and dyneins, motor proteins that move along microtubules, transport organelles, vesicles, and other cellular components within the cell.
Tissue Morphogenesis
Cytoskeletal arrays are pivotal during tissue morphogenesis, guiding the formation and organization of complex multicellular structures:
Cell Sorting and Migration: During tissue development, cells undergo rearrangements, sorting, and migration. Cytoskeletal elements, particularly actin filaments, are involved in driving these processes. For example, actin-based structures help cells move collectively to specific regions, contributing to tissue organization.
Tissue Folding and Remodeling: The controlled assembly and disassembly of cytoskeletal arrays influence tissue folding, shaping, and remodeling. Changes in the actin cytoskeleton can lead to invaginations that give rise to complex structures like the brain's sulci and gyri.
Organogenesis: Cytoskeletal dynamics guide the generation and positioning of organs. For instance, the formation of the heart involves coordinated cell movements driven by the actin cytoskeleton.
Cytoskeletal arrays are central to cell shape determination, cellular movement, and tissue morphogenesis. These arrays enable cells to adapt to their environments, navigate through tissues, and contribute to the intricate processes involved in embryonic development, tissue differentiation, and organ formation. The orchestrated interactions within and between these arrays drive the dynamic changes necessary for the proper function and structure of cells and tissues in complex organisms.
What are the roles of microtubules, actin filaments, and intermediate filaments in cellular processes during development?
Microtubules, actin filaments, and intermediate filaments are key components of the cytoskeletal system, each playing distinct roles in various cellular processes during development. Here's how they contribute to essential developmental processes:
Microtubules
Cell Division and Mitosis: Microtubules form the mitotic spindle, a structure essential for proper chromosome segregation during cell division. They ensure that each daughter cell receives the correct number of chromosomes.
Cell Migration and Morphogenesis: Microtubules guide cell migration by providing tracks for motor proteins like kinesins and dyneins. They help cells move to their designated locations during tissue morphogenesis, such as neuron migration in the developing brain.
Intracellular Transport: Microtubules serve as tracks for motor proteins to transport cellular cargo along their length. This transport is crucial for delivering organelles and molecules to specific cellular locations.
Axon Guidance and Neuronal Development: Microtubules influence the growth and guidance of axons and dendrites in neurons. They guide the extension and branching of neuronal processes to establish proper neuronal connections.
Actin Filaments
Cell Shape and Motility: Actin filaments determine cell shape and support cell motility. They create protrusive structures like lamellipodia and filopodia, which cells use for adhesion, locomotion, and interaction with the extracellular environment.
Cell Migration and Wound Healing: Actin filaments drive cell migration during development and wound healing. They enable cells to contract and exert forces, which are essential for cell movement and closing gaps in tissues.
Cytokinesis: Actin filaments participate in the formation of the contractile ring during cytokinesis, aiding in the physical separation of daughter cells.
Endocytosis and Exocytosis: Actin filaments play roles in membrane dynamics, facilitating processes like endocytosis (cellular intake of materials) and exocytosis (release of cellular products).
Intermediate Filaments
Mechanical Support: Intermediate filaments provide mechanical stability to cells and tissues exposed to mechanical stress. They help maintain cell integrity and tissue structure, especially in epithelial and connective tissues.
Tissue Integrity and Organ Formation: Intermediate filaments contribute to tissue integrity and are critical during tissue morphogenesis. They aid in the shaping and positioning of cells and tissues during organogenesis.
Nuclear Positioning and Anchoring: Intermediate filaments help anchor the nucleus within the cell, influencing cell shape and polarity. This anchoring is important for maintaining proper nuclear positioning and cellular organization.
Cell-Cell Adhesion: Intermediate filaments interact with cell-cell adhesion molecules, contributing to the mechanical strength of tissues and assisting in cell-cell communication.
Microtubules, actin filaments, and intermediate filaments are essential components of the cytoskeletal system with distinct roles in cellular processes during development. They collectively enable processes such as cell division, migration, morphogenesis, tissue integrity, and organ formation, ensuring proper growth, differentiation, and organization of tissues and organs in developing organisms.
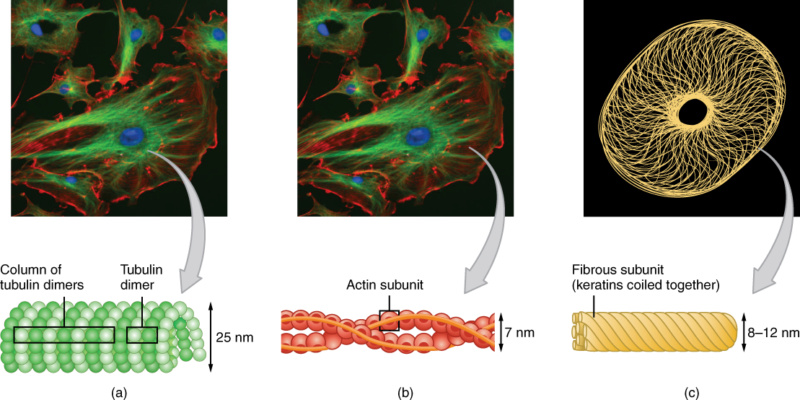
The cytoskeleton consists of (a) microtubules, (b) microfilaments, and (c) intermediate filaments. 1
Appearance of cytoskeletal arrays in the evolutionary timeline
The appearance of cytoskeletal arrays in the evolutionary timeline is hypothesized based on a combination of molecular and cellular evidence, comparative genomics, and the study of extant organisms. While the exact timeline is subject to ongoing research and investigation, here's a generalized outline of the hypothesized appearance of cytoskeletal arrays:
Prokaryotic Origins: Early prokaryotic cells lacked distinct cytoskeletal structures. Simple filamentous proteins would have provided rudimentary structural support and contributed to cell shape.
Eukaryotic Evolution: The emergence of eukaryotic cells would have brought about the development of more complex cellular organization. Microtubule-like proteins would have evolved, possibly serving functions in intracellular transport. Actin-like proteins would have emerged, contributing to cell shape and rudimentary motility.
Early Multicellularity: The transition to multicellularity required improved cell-cell adhesion and communication. Cytoskeletal elements would have begun to play roles in maintaining tissue integrity and supporting multicellular organization.
Diversification of Lineages: Different lineages of organisms would have developed unique adaptations of cytoskeletal arrays to suit their ecological niches. Intermediate filaments would have evolved in animals to provide mechanical stability in tissues subjected to stress.
Emergence of Complex Animals and Plants: Actin filaments and microtubules would have become more specialized, playing critical roles in cell division, cellular movement, and tissue morphogenesis. More sophisticated motor proteins would have evolved, enabling efficient intracellular transport along cytoskeletal tracks.
Modern Eukaryotes: Today's eukaryotic cells possess intricate cytoskeletal networks composed of microtubules, actin filaments, and intermediate filaments. These networks underlie essential cellular processes, including cell division, movement, structural support, and intracellular transport. It's important to emphasize that the evolutionary timeline of cytoskeletal array development is an area of active research, and the details of when specific cytoskeletal components emerged and diversified are still being explored.
De Novo Genetic Information necessary to instantiate cytoskeletal arrays
Creating the mechanisms of cytoskeletal arrays de novo would involve introducing new genetic information in a coordinated sequence to existing genetic material. This hypothetical process would necessitate the origination of various functional components from scratch, each contributing to the formation and regulation of cytoskeletal arrays:
Origination of Protein-Coding Sequences: New genes encoding cytoskeletal proteins, such as tubulin for microtubules and actin for actin filaments, would need to originate. These sequences would contain the necessary instructions for protein synthesis.
Promoters and Enhancers: Regulatory elements like promoters and enhancers would have to arise to control the expression of the newly originated genes. These sequences would determine when and where the genes are transcribed.
Transcription Factors: Transcription factors, which bind to specific regulatory sequences, would need to emerge. These factors would play a role in initiating gene transcription, ensuring proper temporal and spatial expression.
Translation Machinery: The machinery required for translation, including ribosomes and tRNA molecules, would need to be present to decode genetic information and synthesize proteins.
Post-Translational Modifications: Mechanisms for post-translational modifications, such as phosphorylation or acetylation, would need to originate. These modifications fine-tune the activity and function of cytoskeletal proteins.
Motor Proteins and Intracellular Transport: If motor proteins like kinesins and dyneins are considered, the genes encoding these proteins would need to originate. Additionally, regulatory elements and protein-interaction domains would need to evolve to ensure proper transport along microtubules.
Intermediate Filament Proteins: For intermediate filaments, novel genes encoding intermediate filament proteins would have to emerge. These proteins would need specific domains for filament formation and interactions.
Signal Transduction Pathways: New genes and regulatory elements for signaling pathways that regulate cytoskeletal dynamics would need to arise. These pathways control processes like cell migration, division, and differentiation.
Protein-Protein Interactions: Protein domains and motifs that enable protein-protein interactions within cytoskeletal networks would need to evolve. These interactions are crucial for forming functional arrays.
Cellular Localization Sequences: Sequences that direct newly synthesized proteins to their correct subcellular locations would have to emerge. This ensures proper incorporation of cytoskeletal components into the cytoplasm.
Epigenetic Regulation: Epigenetic mechanisms, such as DNA methylation and histone modifications, would need to originate to control gene expression and maintain stable cytoskeletal structures.
Spatial and Temporal Coordination: Mechanisms for coordinating the spatial and temporal assembly of cytoskeletal arrays would have to evolve. This coordination ensures that arrays form in the right place and at the right time.
Regulatory Feedback Loops: Regulatory circuits that respond to cellular cues and feedback loops would need to arise. These mechanisms adjust cytoskeletal dynamics in response to environmental changes.
In this hypothetical scenario, the origin of new genetic information in a precise sequence is crucial for creating the mechanisms required for cytoskeletal arrays. This includes the origination of genes, regulatory elements, protein domains, and intricate cellular processes that collectively contribute to the assembly, organization, and functioning of cytoskeletal arrays.
Manufacturing codes and languages that would have to emerge and be employed to instantiate cytoskeletal arrays
Creating a fully developed cytoskeletal array in an organism would require the establishment of intricate manufacturing codes and languages beyond the genetic information systems. These codes and languages involve various non-genetic factors that contribute to the assembly, organization, and regulation of cytoskeletal arrays:
Protein Folding Codes: The formation of functional cytoskeletal proteins requires precise folding codes. Chaperones and folding factors would need to be created to ensure newly synthesized proteins fold correctly.
Post-Translational Modification Codes: Codes for post-translational modifications, such as phosphorylation, acetylation, and ubiquitination, would need to emerge. Enzymes that catalyze these modifications would ensure proper protein function and interaction within cytoskeletal arrays.
Subcellular Localization Signals: Sequences directing proteins to specific subcellular locations, such as the plasma membrane or the nucleus, would need to be established to ensure correct protein incorporation into the cytoskeletal array.
Assembly and Disassembly Codes: Mechanisms for the assembly and disassembly of cytoskeletal arrays would have to be instantiated. These codes would guide the timing and coordination of filament formation and disintegration.
Cytoskeletal Crosslinking Codes: Codes for proteins that crosslink cytoskeletal filaments together would need to evolve. These crosslinking proteins play roles in organizing and stabilizing the array's structure.
Motor Protein Interaction Codes: Codes for motor proteins like kinesins and dyneins, involved in intracellular transport along cytoskeletal tracks, would need to emerge. These codes ensure specific interactions with filaments and cargo.
Cell Signaling Codes: Codes for signaling molecules that communicate the need for cytoskeletal rearrangements would have to be established. These codes would transmit cues related to cell migration, differentiation, and response to extracellular stimuli.
Cell Adhesion Codes: Codes for cell adhesion molecules and their interactions with the cytoskeleton would need to be introduced. These codes contribute to cell-substrate and cell-cell interactions during cytoskeletal assembly.
Feedback Loop Codes: Codes for feedback loops that sense cytoskeletal dynamics and adjust them in response to cellular needs would need to evolve. These loops maintain array stability and adapt to changing conditions.
Temporal and Spatial Patterning Codes: Codes for coordinating the temporal and spatial organization of cytoskeletal arrays during development would have to arise. These codes ensure arrays form in the correct locations and times.
Epigenetic Regulation Codes: Codes for epigenetic marks that influence the expression of genes related to cytoskeletal components would need to be created. These codes control gene accessibility and expression.
In this scenario, the emergence of fully developed cytoskeletal arrays would require the instantiation of a complex network of manufacturing codes and languages beyond genetic information. These codes would govern protein folding, modifications, interactions, localization, and coordination, enabling the assembly and functioning of the intricate cytoskeletal arrays.
Epigenetic Regulatory Mechanisms necessary to be instantiated for cytoskeletal arrays
The hypothetical creation of epigenetic regulation to develop cytoskeletal arrays from scratch would involve intricate coordination between various systems. While this scenario is speculative, the following are some potential mechanisms that would need to be established:
DNA Methylation: DNA methylation patterns could be established to regulate the expression of genes encoding cytoskeletal components. Methylation of promoter regions could silence or activate these genes.
Histone Modifications: Specific histone modifications, such as acetylation and methylation, would need to be introduced to influence chromatin structure and gene accessibility.
Histone marks associated with active or repressive chromatin states could determine the expression levels of genes related to cytoskeletal proteins.
Non-Coding RNAs: Non-coding RNAs, such as microRNAs and long non-coding RNAs, would need to originate and target mRNAs encoding cytoskeletal proteins, affecting their translation and stability.
Chromatin Remodeling Complexes: Chromatin remodeling complexes could evolve to alter chromatin structure and accessibility, allowing for precise regulation of gene expression during cytoskeletal development.
Epigenetic Inheritance Systems: Systems for epigenetic inheritance would need to be established to ensure the transmission of epigenetic information from one generation to the next. This might involve mechanisms similar to DNA methylation or histone modifications.
Collaboration with Signaling Pathways: Epigenetic regulation would likely collaborate with signaling pathways that respond to extracellular cues. This cooperation would enable the integration of environmental signals into the development of cytoskeletal arrays.
Transcription Factors and Enhancers: Transcription factors and enhancers would need to evolve to precisely control the expression of genes encoding cytoskeletal components.
These factors and enhancers would collaborate with epigenetic marks to fine-tune gene expression patterns.
Cell Cycle Control: Proper coordination with the cell cycle control machinery would be essential to ensure that epigenetic modifications are established and maintained during different phases of cell division and development.
Protein-Protein Interactions: Protein complexes involved in epigenetic regulation would need to interact with other cellular components, including those involved in chromatin structure and gene expression.
Metabolic Pathways: Metabolic pathways might influence epigenetic modifications through the availability of cofactors and substrates required for enzymatic activities.
Feedback Mechanisms: Feedback loops could evolve to maintain epigenetic stability and adapt the epigenome to changing cellular conditions.
In this hypothetical scenario, the creation of epigenetic regulation for cytoskeletal array development would necessitate the establishment of various systems working in concert. These systems would collaborate to ensure the proper establishment, maintenance, and inheritance of epigenetic marks, ultimately influencing the expression of genes related to cytoskeletal components and their assembly into functional arrays.
Signaling Pathways necessary to create, and maintain cytoskeletal arrays
The hypothetical emergence of cytoskeletal arrays from scratch would involve the creation and orchestration of intricate signaling pathways that guide their formation and regulation. While this scenario is speculative, the following are some potential signaling pathways and their interconnectedness:
Wnt Signaling Pathway: The Wnt pathway could be involved in cytoskeletal array development, influencing cell polarity, migration, and tissue morphogenesis.
Wnt signaling crosstalks with pathways like the Rho GTPase pathway to regulate actin dynamics and cytoskeletal rearrangements.
Notch Signaling Pathway: Notch signaling might play a role in cell fate determination and differentiation during cytoskeletal array development.
Cross-talk with other pathways like the Hedgehog pathway could fine-tune cellular responses.
Hedgehog Signaling Pathway: The Hedgehog pathway could contribute to tissue patterning and differentiation, impacting cytoskeletal organization.
Interaction with pathways like the MAPK pathway could influence cytoskeletal rearrangements during cell migration and morphogenesis.
MAPK/ERK Signaling Pathway: The MAPK/ERK pathway could regulate gene expression patterns related to cytoskeletal components and control cellular processes like migration and proliferation.
Crosstalk with the PI3K/AKT pathway could affect actin dynamics and cell motility.
PI3K/AKT Signaling Pathway: The PI3K/AKT pathway might influence cell growth, motility, and survival, which in turn could impact cytoskeletal organization.
Interaction with the mTOR pathway could coordinate cytoskeletal changes with cellular energy status.
TGF-β Signaling Pathway: The TGF-β pathway could contribute to tissue development and morphogenesis by regulating cell differentiation and migration.
Crosstalk with pathways like the Rho GTPase pathway could influence actin dynamics and cytoskeletal reorganization.
Cell Adhesion Signaling: Cell adhesion molecules, such as integrins, could initiate signaling cascades that guide cytoskeletal organization during adhesion, migration, and tissue development.
These pathways could crosstalk with growth factor pathways to integrate extracellular signals.
Cell Cycle Checkpoints and p53 Signaling: Signaling pathways involved in cell cycle checkpoints and DNA damage response might regulate cytoskeletal dynamics to ensure proper cell division and repair.
Crosstalk with growth factor pathways could link cellular growth and division to cytoskeletal changes.
Feedback Loops and Integration: These pathways could form complex feedback loops to maintain homeostasis and adjust cytoskeletal dynamics in response to changing conditions.
Integration with metabolic pathways would connect cellular energy status with cytoskeletal remodeling.
In this speculative scenario, the emergence of cytoskeletal arrays would involve a network of interconnected signaling pathways. These pathways would communicate, crosstalk, and coordinate with each other to ensure proper cytoskeletal organization, cell movement, tissue morphogenesis, and overall developmental processes. Additionally, these pathways would eventually intersect with broader biological systems, such as those governing cell cycle progression, differentiation, and response to environmental cues.
Regulatory codes necessary for maintenance and operation of cytoskeletal arrays
The maintenance and operation of cytoskeletal arrays would necessitate the establishment of regulatory codes and languages that orchestrate their dynamic functions and stability:
Dynamic Remodeling Codes: Codes that initiate dynamic remodeling of cytoskeletal arrays would need to be created. These codes trigger changes in array organization during processes like cell migration, division, and tissue morphogenesis.
Feedback Loop Codes: Feedback loop codes would be essential to sense changes in cellular conditions and adjust cytoskeletal dynamics accordingly. These codes maintain homeostasis and adapt arrays to varying demands.
Stabilization Codes: Codes for stabilizing cytoskeletal arrays, especially during mechanical stress, would need to emerge. These codes prevent excessive disassembly and ensure structural integrity.
Cellular Adhesion Codes: Regulatory codes for cell adhesion molecules would be required. These codes would coordinate adhesion with cytoskeletal dynamics, influencing cell migration and tissue organization.
Motor Protein Regulation Codes: Codes for regulating motor protein activity and interactions with cytoskeletal filaments would be essential. These codes ensure proper intracellular transport and cargo delivery.
Signaling Integration Codes: Regulatory codes that integrate signals from different pathways into cytoskeletal regulation would need to be instantiated. These codes enable the coordination of various cellular processes.
Cell Cycle Coordination Codes: Codes that link cytoskeletal dynamics to the cell cycle would be crucial. These codes ensure proper cytoskeletal organization during cell division and cell cycle progression.
Differentiation and Development Codes: Codes involved in cell differentiation and development would regulate cytoskeletal changes specific to different cell types. These codes contribute to tissue morphogenesis.
Response to Environmental Codes: Codes that allow the cytoskeletal arrays to respond to environmental cues would need to evolve. These codes adapt arrays to changes in the extracellular environment.
Adaptation Codes: Regulatory codes that enable cytoskeletal arrays to adapt to cellular growth and changes in cell size would need to be established. These codes maintain proportionality.
Epigenetic Maintenance Codes: Codes for maintaining epigenetic marks related to cytoskeletal components would be required. These codes ensure stable gene expression patterns over time.
Cross-Talk and Integration Codes: Regulatory codes that facilitate cross-talk and integration between different cytoskeletal components and pathways would need to be instantiated. These codes ensure coordinated functioning.
In this scenario, a complex array of regulatory codes and languages would need to be instantiated to ensure the maintenance, stability, and proper operation of cytoskeletal arrays. These codes would enable precise control over array dynamics, integration with cellular processes, and adaptation to changing conditions.
How would the evolution of cytoskeletal arrays enable the development of diverse cell shapes and functions?
The evolution of cytoskeletal arrays has played a fundamental role in enabling the development of diverse cell shapes and functions across different organisms. Cytoskeletal arrays provide structural support, facilitate intracellular transport, and drive cellular movements, all of which contribute to the formation of distinct cell shapes and the execution of specialized functions. Here's how the evolution of cytoskeletal arrays enables this diversity:
Structural Support and Shape Determination: Cytoskeletal arrays, particularly microtubules and actin filaments, help define and maintain cell shape by providing a structural framework.
The arrangement and organization of cytoskeletal filaments contribute to the development of specific cell shapes. For instance, neuron morphology is influenced by the organization of microtubules and actin filaments.
Cell Polarization and Asymmetry: Cytoskeletal arrays contribute to cell polarization, where different regions of a cell exhibit distinct structures and functions.
Asymmetrical distribution of cytoskeletal components allows cells to establish polarity, which is crucial for processes like cell migration, neuronal development, and tissue morphogenesis.
Cell Migration and Motility: The dynamic rearrangement of actin filaments and microtubules enables cell migration and movement. Lamellipodia and filopodia formation, driven by actin polymerization, are key to cell motility.
Specialized cytoskeletal arrays within cells, such as the leading edge of migrating cells, allow them to move to specific locations within tissues.
Intracellular Transport: Microtubules provide tracks for motor proteins like kinesins and dyneins, enabling the transport of organelles, vesicles, and other cargo within cells.
Intracellular transport facilitated by cytoskeletal arrays allows cells to distribute resources, maintain organelle function, and establish cellular compartments.
Cell Division and Growth: Cytoskeletal arrays are essential for proper cell division. Microtubules form the mitotic spindle, ensuring accurate chromosome segregation, while actin filaments contribute to cytokinesis.
These processes influence cell growth, proliferation, and tissue development.
Cell-Cell Interactions and Tissue Morphogenesis: Cytoskeletal arrays are involved in cell-cell interactions, cell adhesion, and tissue morphogenesis.
Cell adhesion molecules, interacting with cytoskeletal components, facilitate the formation of tissues with specific structures and functions.
Specialized Functions: Different cell types have evolved specialized cytoskeletal arrays that enable unique functions. For example, the flagellar and ciliary arrays allow cells to move through fluid environments, as seen in sperm cells and ciliated epithelial cells.
Response to Environmental Cues: Cytoskeletal arrays enable cells to respond to external signals and adapt their shape and behavior accordingly. This is crucial for processes like wound healing, immune responses, and sensory functions.
Overall, the evolution of cytoskeletal arrays has enabled cells to develop diverse shapes and functions by providing them with the mechanical and dynamic capabilities needed to interact with their environment, move, divide, and perform specialized tasks. The versatile nature of cytoskeletal arrays has been a driving force in the adaptation and diversification of life forms on Earth.
Is there scientific evidence supporting the idea that cytoskeletal arrays were brought about by the process of evolution?
The intricate nature of cytoskeletal arrays and their essential role in cellular functions presents significant challenges for their evolution through gradual, step-by-step processes. The simultaneous instantiation of various codes, languages, signaling pathways, and proteins necessary for the functioning of cytoskeletal arrays is a more plausible explanation than a stepwise evolutionary process. Here's why:
Functional Interdependence: Cytoskeletal arrays require the coordination of numerous components, including protein filaments, motor proteins, signaling pathways, and regulatory codes. Each component is interdependent with others, and their functions are tightly linked. It is implausible that these components would have evolved independently and later coincidentally converged to form functional arrays.
Lack of Intermediate Functionality: The stepwise evolution of cytoskeletal arrays would involve the gradual emergence of individual components and intermediate stages. However, these intermediate stages would likely lack functionality since the array's proper functioning depends on the precise assembly, regulation, and interactions of multiple components.
Irreducible Complexity: Cytoskeletal arrays are irreducibly complex systems where the removal of any essential component would result in the loss of function. For instance, without proper motor proteins, the transport of cellular cargo along filaments would be compromised, rendering the array non-functional.
Informational Complexity: The simultaneous establishment of codes, languages, and regulatory networks that govern the assembly and behavior of cytoskeletal components requires a high degree of informational complexity. This level of complexity challenges the idea that these systems could have gradually emerged through random mutations and natural selection.
Lack of Selective Advantage: During the hypothetical stepwise evolution of cytoskeletal arrays, intermediate stages lacking functionality would not offer a selective advantage to organisms. Natural selection typically favors traits that confer immediate benefits, making it difficult to envision how such non-functional intermediates would persist and accumulate in populations.
Coordination of Components: The coordinated operation of cytoskeletal arrays necessitates the existence of multiple components that interact precisely. The simultaneous emergence of these components through a stepwise process is highly unlikely, as the odds of random mutations leading to the correct sequence of events are exceedingly low.
Fine-Tuned Regulation: The regulation of cytoskeletal arrays involves intricate control mechanisms, including signaling pathways and epigenetic regulation. Such fine-tuned regulation requires these systems to be in place from the beginning to ensure proper functioning.
In summary, the complex and interdependent nature of cytoskeletal arrays, coupled with the lack of intermediate functionality and the informational complexity required, challenges the notion that these structures could have evolved step by step. The concept of intelligent design offers an alternative perspective that accounts for the simultaneous instantiation of all necessary components and systems, reflecting a purposeful and integrated approach to the origin of cytoskeletal arrays.
Irreducibility and Interdependence of the systems to instantiate and operate cytoskeletal arrays
The creation, development, and operation of cytoskeletal arrays involve a complex interplay of manufacturing, signaling, and regulatory codes and languages that are irreducible and interdependent. These systems collectively orchestrate the assembly, organization, and functioning of cytoskeletal arrays, and their simultaneous instantiation is more plausible than a stepwise evolutionary process. Here's how these components interact and why their interdependence challenges gradual evolution:
Manufacturing Codes and Post-Translational Modifications
Manufacturing codes guide the synthesis of cytoskeletal proteins, but their functionality often relies on post-translational modifications (PTMs) like phosphorylation, acetylation, and ubiquitination. PTMs fine-tune the properties of cytoskeletal components, affecting their interactions, stability, and function within the array. The absence of the manufacturing code would prevent the production of proteins, and the absence of PTMs would hinder their proper function.
Regulatory Codes and Epigenetic Regulation
Regulatory codes determine when and where cytoskeletal genes are expressed, while epigenetic regulation controls gene accessibility and expression over time. Epigenetic marks, such as DNA methylation and histone modifications, influence the expression of cytoskeletal genes and their responsiveness to cellular cues. Without regulatory codes, genes wouldn't be expressed appropriately, and without epigenetic regulation, stable gene expression patterns necessary for cytoskeletal assembly wouldn't be maintained.
Signaling Pathways and Protein Activation
Signaling pathways communicate extracellular cues to the cell, triggering responses that impact cytoskeletal arrays. Crosstalk between signaling pathways allows integration of multiple cues for coordinated cellular behavior. For example, growth factor signaling can influence cytoskeletal dynamics via pathways like MAPK/ERK and PI3K/AKT. If one signaling pathway were missing or disrupted, the cell's ability to receive and respond to signals influencing cytoskeletal organization would be compromised.
Feedback Loops and Adaptation
Feedback loops regulate cytoskeletal dynamics in response to changing conditions, ensuring stability and adaptation. These loops integrate information from various cellular processes, signaling pathways, and environmental cues to adjust cytoskeletal organization. Without functional feedback loops, cells would struggle to maintain the balance between stability and adaptability required for proper cytoskeletal function.
Motor Protein Interaction and Transport Codes
Motor protein codes and codes for cargo recognition ensure efficient intracellular transport along cytoskeletal tracks. These codes are intertwined with signaling pathways and cellular cues that determine when and where transport occurs. Without proper motor protein codes and the integration of transport with signaling, cellular cargo distribution would be impaired. The intricate interdependence of these codes and languages presents a significant challenge for the gradual evolution of cytoskeletal arrays. In a stepwise process, the absence or dysfunction of one system would disrupt the entire functional network. Since intermediate stages without complete systems would not offer a selective advantage, it's more reasonable to propose that these systems were instantiated together, fully operational, to enable the creation and function of cytoskeletal arrays. This perspective aligns with the concept of intelligent design, where the integrated complexity of cytoskeletal arrays suggests a purposeful and coordinated origin.
Once is instantiated and operational, what other intra and extracellular systems are Cytoskeletal Arrays interdependent with?
Once cytoskeletal arrays are instantiated and operational, they become interdependent with a wide range of intra and extracellular systems, reflecting their central role in various cellular processes. These interdependencies ensure proper cell functioning, structural integrity, and adaptation to the environment. Here are some of the key systems that cytoskeletal arrays are interdependent with:
Intracellular Systems
Cell Membrane and Receptor Systems: Cytoskeletal arrays interact with the cell membrane through adhesion molecules and receptor complexes. This interaction affects cell shape, adhesion, and signaling.
Endomembrane System: Vesicle trafficking and organelle movement within cells rely on cytoskeletal tracks and motor proteins. Proper function of the endomembrane system is intertwined with cytoskeletal dynamics.
Organelles and Subcellular Structures: Proper positioning and segregation of organelles during cell division and cellular activities are facilitated by cytoskeletal arrays. For instance, the mitotic spindle (made of microtubules) ensures accurate chromosome segregation.
Nucleus and Nuclear Pore Complexes: The nuclear envelope interacts with cytoskeletal elements during cell migration and division. Nuclear pore complexes enable molecular transport between the nucleus and cytoplasm, influenced by cytoskeletal dynamics.
Mitochondria and Energy Production: Mitochondria, responsible for energy production, require efficient transport along cytoskeletal tracks for proper distribution within the cell.
Extracellular Systems
Extracellular Matrix (ECM)
Cytoskeletal arrays interact with the ECM through integrin-mediated adhesion. This interaction influences cell migration, differentiation, and tissue organization.
Cell-Cell Junctions: Junctions like adherens junctions and desmosomes anchor cytoskeletal components to neighboring cells, contributing to tissue integrity and organization.
Cell-Cell Communication: Cytoskeletal arrays are essential for cellular movements that facilitate direct cell-cell communication, such as during immune responses and tissue development.
Extracellular Signaling Molecules: Extracellular signaling molecules, like growth factors and cytokines, can trigger cytoskeletal rearrangements that influence cell behavior and responses to the environment.
Mechanical Forces and Tissue Morphogenesis: Mechanical forces exerted by cytoskeletal arrays can shape tissues during development and contribute to wound healing and tissue remodeling.
Nervous System: In neurons, cytoskeletal arrays are critical for dendritic branching, axon guidance, and synapse formation. These processes underlie nervous system development and function.
The interdependence of cytoskeletal arrays with these intra and extracellular systems underscores their central role in cellular physiology and multicellular organismal development. The complexity and precision of these interrelationships raise questions about how such an integrated system could have emerged through gradual evolution, leading proponents of intelligent design to posit a purposeful and coordinated origin of these systems.
The interdependence of cytoskeletal arrays with various intra and extracellular systems reveals an intricate web of relationships that points toward a purposeful and coordinated design rather than a gradual evolutionary process. This can be highlighted through a syllogism:
1. Complex Interdependencies: Cytoskeletal arrays exhibit intricate interdependencies with multiple cellular and extracellular systems, including the cell membrane, endomembrane system, organelles, nucleus, mitochondria, extracellular matrix, cell-cell junctions, communication networks, mechanical forces, and the nervous system.
2. Interlocked Functions: These interdependencies are not just linear but form a highly interlocked network. The functioning of one system directly impacts others, and their coordinated operation is essential for cell shape, movement, division, tissue organization, and various specialized functions.
3. Absence of Selective Advantage in Intermediate Stages: The gradual evolution of these interconnected systems presents a challenge. In a stepwise scenario, intermediate stages lacking complete systems would not offer selective advantage, as isolated components would likely serve little or no purpose.
4. Simultaneous Emergence: The simultaneous emergence of these interdependent systems in a fully operational state is a more reasonable explanation. A purposeful and orchestrated design would ensure that all necessary components are instantiated together, allowing cells to function optimally from the outset.
Informational Complexity: The intricate interplay of codes, languages, signaling pathways, and protein interactions within and between these systems requires a high level of informational complexity. Such complexity suggests an intentional design rather than a gradual accumulation of random changes.
1. Cytoskeleton