Apoptosis
Apoptosis is a fundamental biological process of programmed cell death that plays a crucial role in shaping tissues and organs during development, maintaining tissue homeostasis, and eliminating damaged or surplus cells. Unlike necrosis, which is a chaotic and uncontrolled form of cell death, apoptosis follows a tightly regulated sequence of events. During apoptosis, cells undergo controlled dismantling in response to specific signals. These signals can originate from internal factors like DNA damage or external cues such as growth factor withdrawal. The process involves a series of distinct stages:
Initiation: Apoptosis can be triggered by various stimuli, either intrinsic (e.g., DNA damage) or extrinsic (e.g., binding of death ligands to cell surface receptors).
Signaling Pathways: Activation of signaling pathways leads to the expression or activation of specific genes and proteins that orchestrate apoptosis.
Caspase Activation: Caspases, a family of protease enzymes, play a central role in apoptosis. Initiator caspases are activated first, triggering a cascade that culminates in the activation of executioner caspases.
Cellular Changes: Apoptotic cells undergo characteristic changes, including cell shrinkage, chromatin condensation, and nuclear fragmentation.
Plasma Membrane Alterations: Phospholipids flip from the inner to the outer leaflet of the cell membrane, signaling to phagocytes that the cell is undergoing apoptosis.
Blebbing: The cell membrane forms bulges called blebs, which are eventually shed as apoptotic bodies containing cellular debris.
Phagocytosis: Phagocytes recognize apoptotic bodies through "eat me" signals on their surfaces. The phagocytes engulf and digest these bodies, preventing inflammation and tissue damage.
Resolution: Apoptotic cells are efficiently cleared, leading to minimal impact on surrounding tissues.
What is the role of apoptosis in sculpting and refining tissues during development?
Tissue Patterning and Shape Formation: Apoptosis is involved in eliminating excess cells and shaping developing tissues and organs. By selectively removing specific cells, apoptosis helps establish and refine the proper structure and shape of organs. This is particularly important in processes like limb development, where apoptosis is responsible for creating spaces between digits (interdigital spaces) and sculpting the final shape of the limb.
Digit Formation: In vertebrate limb development, apoptosis removes cells from the areas between developing digits, allowing them to separate. This process is essential for forming individual fingers or toes. The removal of cells in the interdigital regions is orchestrated by signaling pathways that activate apoptosis in a precisely coordinated manner.
Nervous System Development: Apoptosis plays a role in shaping the nervous system. During neural development, there is an initial overproduction of neurons, and apoptosis eliminates excess neurons that do not establish proper connections or synapses. This helps refine neural circuits and optimize their functionality.
Organ Development and Homeostasis: Apoptosis is involved in eliminating unwanted or abnormal cells during organ development. It helps shape organs by selectively removing cells that would otherwise disrupt the proper structure or function of the organ. Additionally, apoptosis continues to operate in adulthood to maintain tissue homeostasis by removing damaged or aged cells.
Immune System Formation: In the immune system, apoptosis is involved in shaping lymphoid organs and eliminating self-reactive immune cells. Immature immune cells undergo selection processes that involve apoptosis to ensure that only functional and non-self-reactive cells mature and become part of the immune repertoire.
Cell Number Control: Apoptosis helps regulate cell numbers in various tissues to achieve the appropriate balance between cell proliferation and cell death. This balance is essential for maintaining the overall integrity and functionality of tissues and organs.
Preventing Abnormal Development: Apoptosis acts as a quality control mechanism by eliminating cells with developmental defects, DNA damage, or other abnormalities. This prevents the propagation of genetic or cellular errors that could lead to malformation or disease.
Apoptosis serves as a precise and controlled mechanism for eliminating cells that are no longer needed or that could disrupt proper tissue and organ development. By sculpting and refining tissues, apoptosis contributes to the establishment of functional and well-organized structures in the developing organism.
How do the regulatory mechanisms of apoptosis ensure the precise elimination of specific cell populations?
The regulatory mechanisms of apoptosis ensure the precise elimination of specific cell populations through a series of tightly controlled steps and molecular interactions. These mechanisms allow cells to be targeted for elimination while minimizing the risk of collateral damage to neighboring cells. Here's how these regulatory mechanisms work:
Caspase Activation: Caspases are a group of protease enzymes that play a central role in apoptosis. They are initially present as inactive procaspases. Activation of caspases is a key step in initiating apoptosis. Activation can occur through two main pathways: the extrinsic pathway, which is initiated by death receptors on the cell surface, and the intrinsic pathway, which is activated by intracellular stress signals.
Bcl-2 Family Proteins: The Bcl-2 family of proteins includes both pro-apoptotic and anti-apoptotic members. These proteins regulate the permeability of the mitochondrial membrane and the release of cytochrome c, a trigger for caspase activation. Pro-apoptotic members promote apoptosis by inducing mitochondrial membrane permeabilization, while anti-apoptotic members inhibit apoptosis by preventing cytochrome c release.
Caspase Cascade: Activated caspases initiate a cascade of proteolytic events that lead to cell dismantling. Initiator caspases, such as caspase-8 and caspase-9, cleave and activate effector caspases, such as caspase-3. Effector caspases cleave a wide range of cellular substrates, including structural and functional proteins, resulting in cell disassembly.
Cellular Engulfment: Phagocytic cells, such as macrophages, play a role in engulfing apoptotic cells. This process, called phagocytosis or efferocytosis, prevents the release of cellular contents that could trigger inflammation and damage neighboring cells. Engulfment is facilitated by "eat-me" signals displayed on the surface of apoptotic cells and recognized by phagocytes.
Death Receptors and Ligands: In the extrinsic pathway, death receptors on the cell surface, such as Fas (CD95) and TNF receptor, bind to specific ligands. This binding triggers a signaling cascade that leads to caspase activation and apoptosis. This pathway is particularly important for immune system regulation and defense against infected or abnormal cells.
Survival Factors and Apoptotic Signals: Cells receive survival signals from their environment through growth factors and other molecules. These signals activate intracellular pathways that promote cell survival by inhibiting apoptosis. Conversely, absence of survival signals or exposure to apoptotic signals can tip the balance towards apoptosis.
Checkpoint Mechanisms: Cells have built-in checkpoints to ensure that apoptosis is activated only when appropriate. For example, DNA damage triggers the activation of p53, a tumor suppressor protein. p53 can induce cell cycle arrest to allow DNA repair, but if the damage is severe, it can activate apoptosis to prevent propagation of genetic errors.
Tissue-Specific Regulation: The regulation of apoptosis can be tissue-specific. Some cells are more sensitive to apoptosis signals due to their expression of certain proteins or the presence of specific receptors. This allows for precise elimination of particular cell populations during development, differentiation, and tissue homeostasis.
Collectively, these regulatory mechanisms ensure that apoptosis is a controlled and highly orchestrated process. The interplay between pro-apoptotic and anti-apoptotic signals, along with checks and balances, allows cells to be targeted for elimination with high specificity, contributing to the precise elimination of specific cell populations during development and tissue maintenance.
How were apoptotic pathways instantiated to balance tissue growth and removal in different organisms?
The instantiation of apoptotic pathways to balance tissue growth and removal in different organisms is a complex process that involves both conserved and organism-specific mechanisms. While the exact details can vary among species, certain core principles guide the establishment of apoptotic pathways to achieve proper tissue homeostasis:
Conserved Core Components: Many of the core components of apoptotic pathways are evolutionarily conserved across different organisms. For instance, caspases, Bcl-2 family proteins, and death receptors are present in a wide range of species. These conserved components provide a foundation for the initiation and execution of apoptosis.
Diversification of Pathways: Different organisms may have evolved specific apoptotic pathways tailored to their physiological and developmental needs. For example, the extrinsic pathway involving death receptors is more prominent in mammals, particularly in immune regulation. In contrast, the intrinsic pathway, centered around mitochondria, is a more universal mechanism present in various organisms.
Tissue-Specific Regulation: Apoptosis is often regulated in a tissue-specific manner. The expression of certain pro-apoptotic and anti-apoptotic factors can vary between tissues, allowing for selective control of apoptosis in different parts of the body. This tissue-specific regulation is crucial for achieving the proper balance between tissue growth and removal.
Adaptation to Developmental Stages: Apoptosis is involved in various developmental stages, from embryogenesis to adulthood. Different organisms have adapted their apoptotic pathways to suit the requirements of each developmental phase. For example, apoptosis during embryogenesis shapes tissue formation and organ development, while apoptosis in adult organisms contributes to tissue maintenance and removal of damaged cells.
Integration with Signaling Networks: Apoptotic pathways are integrated with other signaling networks in the cell. For instance, growth factors, survival signals, and DNA damage responses influence the balance between survival and apoptosis. The integration of apoptotic pathways with these networks allows organisms to respond dynamically to changing environmental and cellular conditions.
Fine-Tuning and Feedback: Apoptotic pathways often incorporate feedback loops and fine-tuning mechanisms. Regulatory feedback loops can enhance the precision of apoptotic responses and prevent excessive cell death. This ensures that tissue removal occurs only when needed and prevents the loss of essential cell populations.
Genetic and Functional Redundancy: Some organisms possess genetic redundancy, where multiple genes encode functionally similar proteins. This redundancy provides backup mechanisms to ensure that apoptotic pathways can function effectively even if some components are compromised.
Overall, the instantiation of apoptotic pathways in different organisms involves a combination of conserved elements and adaptive mechanisms. The diverse strategies employed by different species reflect the complexity and flexibility of apoptotic regulation across the tree of life.
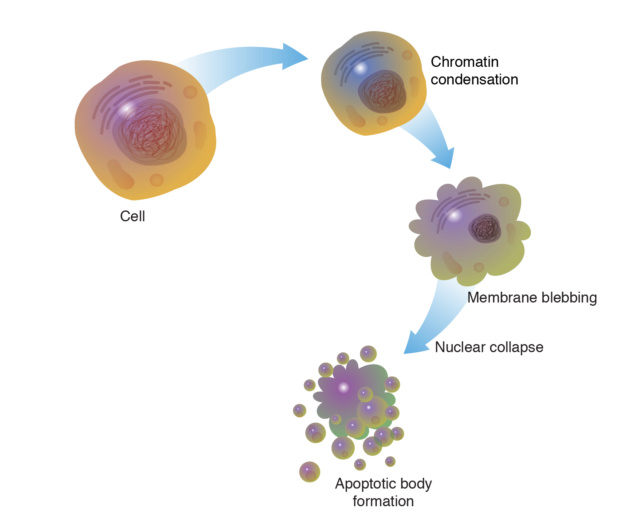
Apoptosis is critical for normal development, tissue remodeling, immune response regulation, and maintaining tissue integrity. Dysregulation of apoptosis is associated with various diseases, including cancer (where excessive cell survival occurs) and neurodegenerative disorders (where excessive cell death occurs). The complex and highly orchestrated nature of apoptosis suggests that it serves as a fine-tuned mechanism that is essential for the proper functioning of multicellular organisms. The emergence of apoptosis, a complex process involving cellular self-destruction, would have required the establishment of specific manufacturing codes and languages to orchestrate the production, assembly, and regulation of the components involved.
Appearance of apoptosis in the evolutionary timeline
The emergence of apoptosis, a highly regulated form of programmed cell death, is believed to have played a crucial role in shaping the development and complexity of multicellular organisms. While the exact timing of when apoptosis first appeared in the evolutionary timeline is not definitively known, researchers have proposed theories based on comparative studies and evidence from various organisms.
Early Single-Celled Organisms (Prokaryotes): It is unlikely that apoptosis, as understood in multicellular organisms, would have existed in early single-celled organisms like prokaryotes. The cellular complexity and mechanisms necessary for programmed cell death as seen in higher organisms would not have been present.
Emergence of Eukaryotes: With the supposed evolution of eukaryotic cells, which possess more complex internal structures and organelles, the potential for controlled cell death mechanisms might have increased. However, at this stage, any form of cell death would have been more similar to necrosis, a less regulated process compared to apoptosis.
Multicellular Organisms: The transition to multicellularity would have introduced new challenges related to cell differentiation, tissue development, and maintaining proper cell numbers. Apoptosis might have started to emerge as a potential mechanism to eliminate excess or damaged cells, refine tissue structures, and aid in proper development. Rudimentary forms of programmed cell death would have been present even in early multicellular organisms.
Invertebrates: As organisms became more complex, particularly within the animal kingdom, it is speculated that apoptosis would have become more sophisticated. Invertebrates would have employed some form of programmed cell death to assist in shaping tissues, organs, and structures during development. However, the molecular mechanisms and regulation would have been less intricate than in more advanced organisms.
Vertebrates: With the appearance of vertebrates and the evolution of intricate organ systems, it's suggested that apoptosis would have become more refined and tightly regulated. This process would have played critical roles in various aspects including organogenesis, immune system development, tissue repair, and the removal of potentially harmful or unnecessary cells.
Evolution of Adaptive Immunity: The emergence of the adaptive immune system in vertebrates would have supposedly introduced a necessity for precise cell death mechanisms to eliminate unwanted immune cells and prevent autoimmune responses. Apoptosis might have played a central role in maintaining the balance of the immune system.
De Novo Genetic Information to instantiate apoptosis
The emergence of apoptosis, a complex and regulated process of programmed cell death, would have required the addition of specific genetic information to enable its functions.
Apoptosis Initiation Genes: New genetic elements encoding proteins responsible for initiating apoptosis would need to evolve. These proteins, often activated in response to cellular stress or signals, would trigger the apoptotic cascade.
Caspase Genes: Caspases are a family of protease enzymes crucial for executing apoptosis. The evolution of apoptosis would require the emergence of genes encoding various types of caspases, each with distinct roles in apoptosis progression.
Cell Death Regulatory Proteins: Proteins that regulate the balance between pro-apoptotic and anti-apoptotic signals would need to evolve. These proteins would control the decision-making process of whether a cell undergoes apoptosis.
Apoptotic Signaling Pathways: New genetic information would be necessary to establish the intricate signaling pathways that transmit pro-apoptotic and anti-apoptotic signals, leading to the activation of caspases and subsequent cell death.
DNA Fragmentation Genes: During apoptosis, DNA is fragmented into smaller pieces. Genes responsible for this fragmentation process would need to evolve, ensuring the controlled degradation of the cell's genetic material.
Cellular Membrane Alteration Genes: Changes in the cell's membrane structure and properties are common in apoptosis. Genes encoding proteins responsible for altering the membrane would be essential.
Phagocytosis Recognition Genes: In multicellular organisms, phagocytes engulf apoptotic cells. Genes encoding surface molecules on apoptotic cells that signal for their recognition and engulfment by phagocytes would need to emerge.
Regulation of Apoptosis Timing: The evolution of apoptosis would require genetic mechanisms to regulate the timing of cell death, ensuring that it occurs at the right stage of development or in response to appropriate signals.
Apoptosis in Single-Celled Organisms: In prokaryotes or unicellular eukaryotes, apoptosis-like mechanisms could involve genes that trigger self-destruction under certain conditions, such as nutrient depletion or environmental stress.
The emergence of apoptosis would require the simultaneous addition of multiple genes and regulatory elements to form a functional and controlled process of programmed cell death. The complexity and coordination involved in apoptosis suggest that such genetic information would need to be instantiated and integrated into existing genetic systems to achieve its crucial functions.
Manufacturing codes and languages employed to instantiate apoptosis
Exempting genetic codes and languages, the emergence of apoptosis would have required the establishment of intricate manufacturing codes and languages within cells. These codes and languages encompass a series of coordinated biochemical processes that lead to the controlled dismantling and elimination of cells.
Cellular Signaling Network: Cells would need to develop a sophisticated network of signaling pathways that sense both internal and external cues. These pathways would translate specific triggers into appropriate responses that initiate apoptosis.
Protein Activation and Inactivation: Intricate protein interactions would be established, involving activation and inactivation of key enzymes and factors. This would require the development of mechanisms to ensure precise timing and regulation.
Enzyme Cascades: Manufacturing codes would involve creating enzyme cascades, like the caspase cascade in apoptosis. These cascades amplify signals, ensuring a rapid and coordinated response throughout the cell.
Protein Modifications: The emergence of new manufacturing codes would facilitate various protein modifications, including phosphorylation, ubiquitination, and proteolytic cleavage. These modifications would control protein functions within the apoptosis pathway.
Membrane Remodeling: Manufacturing languages would govern the reorganization of cellular membranes during apoptosis, leading to characteristic changes such as blebbing and externalization of phospholipids.
Cellular Morphological Changes: The process of apoptosis involves specific changes in cell shape and structure. Manufacturing codes would be needed to coordinate these changes, such as cell shrinkage and chromatin condensation.
Phagocytosis Coordination: Apoptotic bodies, the remnants of apoptotic cells, must be recognized and engulfed by phagocytes to prevent inflammation. Manufacturing languages would regulate the signals that facilitate this recognition and engulfment.
Removal of Cellular Debris: Efficient removal of cellular debris resulting from apoptosis would necessitate manufacturing codes for the disassembly and recycling of cellular components.
Tissue Repair and Homeostasis: Manufacturing languages would ensure that apoptosis contributes to tissue repair and homeostasis, avoiding excessive cell loss and enabling the replacement of damaged cells.
Integration with Other Processes: The codes for apoptosis would need to integrate with other cellular processes, such as inflammation and cell survival pathways, to maintain balanced responses and prevent excessive tissue damage. The development of these manufacturing codes and languages would require a comprehensive understanding of cell biology, biochemistry, and cellular interactions. The interplay and synchronization of these processes would be vital to ensure proper apoptosis execution while avoiding unintended consequences. The emergence of apoptosis would represent a finely tuned system that contributes to the overall health and functionality of multicellular organisms.
Epigenetic Regulatory Mechanisms necessary to be instantiated for Apoptosis
To instantiate the development of apoptosis from scratch, several epigenetic regulatory mechanisms would need to be created and subsequently employed. These mechanisms involve intricate interactions between different cellular systems to ensure proper regulation and functioning of apoptosis.
DNA Methylation: Epigenetic marks involving DNA methylation could be established to regulate the expression of genes related to apoptosis. Methylation of promoter regions could silence or activate specific apoptotic genes.
Histone Modifications: Various histone modifications, such as acetylation, methylation, and phosphorylation, would need to be established to influence the accessibility of chromatin regions associated with apoptotic genes.
Non-Coding RNAs: Non-coding RNAs, like microRNAs and long non-coding RNAs, could emerge to fine-tune the expression of apoptotic genes by interacting with mRNA transcripts or chromatin-modifying complexes.
Systems Involved in Instantiating and Employing Apoptotic Regulation
Transcriptional Machinery: The core transcriptional machinery, including RNA polymerases and transcription factors, would need to be in place to enable the transcription of apoptotic genes based on the epigenetic marks.
Chromatin Remodeling Complexes: Complexes responsible for chromatin remodeling would play a crucial role in modulating the accessibility of apoptotic gene promoters and enhancers, guided by epigenetic modifications.
RNA Processing Machinery: The emergence of a functional RNA processing machinery would be necessary for the production and regulation of non-coding RNAs that control apoptotic gene expression.
Systems Collaborating to Maintain Apoptotic Regulation
DNA Repair and Replication Systems: These systems would collaborate to ensure the faithful inheritance of epigenetic marks during cell division, maintaining the proper epigenetic regulation of apoptotic genes.
Cell Signaling Pathways: Cellular signaling pathways that respond to various cues, such as stress or developmental signals, would work in conjunction with epigenetic mechanisms to initiate or suppress apoptosis as needed.
Apoptotic Pathways: Once initiated, the apoptotic pathways themselves would engage in a feedback loop to reinforce or inhibit apoptotic signals, further shaping the outcome of the process.
The instantiation and employment of epigenetic regulation for apoptosis would require a coordinated effort between multiple cellular systems. The DNA methylation, histone modification, and non-coding RNA systems would work together to establish the proper epigenetic marks on apoptotic genes. Subsequently, transcriptional machinery, chromatin remodeling complexes, and RNA processing machinery would collaborate to translate these marks into appropriate gene expression patterns. Maintenance of this regulation would involve systems that ensure accurate epigenetic inheritance during cell division and the integration of signals from various cellular pathways to determine whether apoptosis should be initiated or inhibited. Overall, these interdependent systems would contribute to the proper development and functioning of apoptosis.
Signaling Pathways necessary to create, and maintain apoptosis
The emergence of apoptosis from scratch would require the creation and subsequent involvement of specific signaling pathways that coordinate and regulate the process of programmed cell death. These signaling pathways would be interconnected, and interdependent, and would crosstalk with each other and with other biological systems to ensure proper apoptotic regulation.
Intrinsic Apoptotic Pathway
Activation Trigger: Cellular stress, DNA damage, or other internal factors.
Pathway: Mitochondrial outer membrane permeabilization (MOMP) releases cytochrome c, activating caspases.
Crosstalk: Interacts with anti-apoptotic Bcl-2 family proteins that counteract MOMP.
Connection to Other Systems: Responds to DNA damage and stress signals, integrates with cell cycle checkpoints, and engages DNA repair systems.
Extrinsic Apoptotic Pathway
Activation Trigger: External signals, such as binding of death ligands (e.g., Fas ligand) to death receptors (e.g., Fas receptor).
Pathway: Ligand-receptor binding activates caspase-8, initiating downstream caspase cascade.
Crosstalk: Inhibitory proteins like FLIP can block caspase-8 activation.
Connection to Other Systems: Interacts with immune responses and inflammation pathways, and integrates signals from death ligands.
Caspase Activation Pathway
Activation Trigger: Initiators such as caspase-8 (extrinsic) or caspase-9 (intrinsic) are activated.
Pathway: Initiators cleave and activate effector caspases (caspase-3, -6, -7) leading to cell dismantling.
Crosstalk: Inhibitors like XIAP can directly block effector caspases.
Connection to Other Systems: Links to DNA repair systems and cellular stress responses.
PI3K/AKT Survival Pathway
Activation Trigger: Growth factors and survival signals.
Pathway: Activation of PI3K and AKT promotes cell survival and inhibits apoptosis by phosphorylating pro-apoptotic factors.
Crosstalk: Counteracted by PTEN, which opposes PI3K.
Connection to Other Systems: Integrates with cell growth, proliferation, and nutrient sensing pathways.
p53 Signaling Pathway
Activation Trigger: DNA damage or stress signals.
Pathway: Activation of p53 leads to transcription of pro-apoptotic genes (e.g., PUMA, Bax).
Crosstalk: Interaction with MDM2 regulates p53 stability.
Connection to Other Systems: Coordinates DNA repair mechanisms, cell cycle arrest, and apoptosis in response to genotoxic stress.
NF-κB Pathway
Activation Trigger: Inflammation, immune responses, and stress signals.
Pathway: Activation of NF-κB promotes cell survival and inhibits apoptosis by regulating the expression of anti-apoptotic genes.
Crosstalk: IKK complex phosphorylation controls NF-κB activation.
Connection to Other Systems: Links apoptosis to inflammation and immune responses.
These signaling pathways are interconnected, often converging and diverging to fine-tune the apoptotic response based on cellular conditions. They are interdependent, with some pathways directly regulating others to maintain proper cell fate decisions. Crosstalk between pathways allows cells to integrate various signals, ensuring a balanced and appropriate apoptotic response. Additionally, these pathways interact with broader biological systems, including immune responses, DNA repair, and cell cycle control, to create a sophisticated network that orchestrates the emergence and regulation of apoptosis.
Regulatory codes necessary for maintenance and operation of apoptosis
The emergence, maintenance, and operation of apoptosis would require the instantiation of regulatory codes and languages that ensure proper coordination, execution, and control of the process. These regulatory codes and languages would involve intricate interactions between various molecules and cellular components to regulate apoptosis.
Transcriptional Regulatory Code: The activation of specific genes associated with apoptosis, including pro-apoptotic and anti-apoptotic factors, would require a transcriptional regulatory code. Transcription factors such as p53, NF-κB, and STATs could bind to specific DNA sequences, promoting or inhibiting the expression of apoptotic genes.
Post-Translational Modification Code: Phosphorylation, acetylation, ubiquitination, and other post-translational modifications would be involved in modulating the activity of apoptotic regulators. For instance, phosphorylation of Bcl-2 family proteins can affect their pro-apoptotic or anti-apoptotic functions.
Protein Interaction Networks: Regulatory codes would govern the interactions between proteins involved in apoptosis. These interactions would dictate the formation of protein complexes, such as apoptosome assembly, which activate caspase cascades.
Inhibitory and Activating Signals: The language of signaling molecules, including growth factors, cytokines, and ligands, would determine whether apoptosis is initiated or inhibited. These signals would engage receptors and trigger downstream signaling events.
Feedback Loops: Regulatory codes could involve feedback loops that sense cellular stress, DNA damage, or other triggers and modulate the apoptotic response accordingly. These loops could fine-tune the process to ensure an appropriate response.
Epigenetic Regulation: Epigenetic marks, such as DNA methylation and histone modifications, might be established to regulate the accessibility of apoptotic genes. Epigenetic changes could be involved in maintaining proper balance between pro-apoptotic and anti-apoptotic factors.
Protein Stability Control: Regulatory codes would control the stability of key apoptosis regulators. For instance, proteins like p53 are tightly regulated through degradation mechanisms.
Feedback Inhibition: Inhibitory factors, such as caspase inhibitors (cIAPs) and anti-apoptotic Bcl-2 proteins, would be part of the regulatory language to prevent excessive or premature apoptosis.
Cell-Cell Communication: Apoptosis can be influenced by signals from neighboring cells. Communication between cells through death ligands and their receptors (e.g., Fas/FasL) would be an important aspect of the regulatory code.
Feedback to Other Cellular Processes: The apoptotic regulatory code would interact with other cellular processes like DNA repair, cell cycle control, and immune responses to ensure proper integration of signals and responses.
These regulatory codes and languages would be intertwined, forming a complex network that governs the initiation, execution, and modulation of apoptosis. The balance and coordination of these codes would be crucial to ensure the appropriate elimination of cells and maintenance of tissue homeostasis.
Is there scientific evidence supporting the idea that apoptosis is brought about by the process of evolution?
Apoptosis, or programmed cell death, is a complex and highly regulated process that plays a crucial role in maintaining tissue homeostasis, development, and immune responses.
Functional Complexity: Apoptosis involves a series of intricate steps, including cellular signaling, organelle fragmentation, DNA degradation, and cell engulfment by neighboring cells or phagocytes. These steps require multiple interacting components to function properly.
Regulation and Signaling: Apoptosis is tightly regulated to ensure that it occurs when necessary and doesn't harm the organism. It requires a sophisticated signaling network involving pro-apoptotic and anti-apoptotic factors. This network must be fully operational to prevent accidental cell death or survival.
Cellular Communication: Apoptosis often involves communication between cells to signal the need for cell death. The evolution of the ability to send and receive such signals, and the corresponding cellular responses, is complex.
Selective Advantage: For an evolutionary process to proceed, intermediate stages must offer some selective advantage to the organism. The early stages of apoptosis, without the complete set of regulatory mechanisms, could potentially lead to harmful outcomes, such as uncontrolled cell death or immune system dysfunction.
Interdependence of Components: Apoptosis requires the coordinated activity of various proteins, enzymes, and regulatory factors. The evolution of these components in a stepwise manner might not offer any functional advantage until the entire system is in place.
Conservation and Complexity: Apoptosis is a highly conserved process found across diverse organisms. This suggests that the components and mechanisms involved are fundamental to life. The complex nature of apoptosis raises questions about how these components could have evolved incrementally.
Cellular Consequences: Incomplete or partially functional apoptotic pathways could have detrimental effects on an organism. If intermediate stages led to excessive cell death or impaired cell survival, they might be disadvantageous and not favored by natural selection.
Integration with Other Systems: Apoptosis is interconnected with various cellular processes, including cell proliferation, immune responses, and tissue development. Evolution would need to consider how apoptosis fits into these existing systems.
Irreducibility and Interdependence of the systems to instantiate and operate apoptosis
The emergence, development, and operation of apoptosis involve a highly intricate and interdependent web of manufacturing, signaling, and regulatory codes and languages. These codes are irreducible and cannot function independently; they rely on each other to achieve a coherent and functional apoptotic process. Communication between these codes is crucial for normal cell operation, ensuring that apoptosis is triggered, executed, and controlled accurately. It becomes apparent that these interdependencies point to a simultaneous and purposeful instantiation of these codes.
Manufacturing Codes and Languages: The manufacturing codes are responsible for producing the intricate molecular machinery required for apoptosis, including the components of the apoptosome, caspases, and their regulators. These codes are interdependent, as without the manufacturing of these specific components, apoptosis cannot be executed effectively. The coordination between the manufacturing codes ensures that the required proteins and structures are produced accurately and in the right quantities.
Signaling Pathways: Signaling pathways, like those involving death receptors and their ligands, guide the initiation and propagation of apoptotic signals. These pathways communicate with each other through crosstalk, amplifying or dampening the apoptotic response based on cellular context. For example, interactions between the extrinsic and intrinsic pathways ensure a balanced response. Without these interconnected signaling pathways, the decision to initiate apoptosis or not would lack proper integration and coordination.
Regulatory Codes and Languages: Regulatory codes control the activation, inhibition, and modulation of apoptosis. These codes are interconnected, forming a delicate balance between pro-apoptotic and anti-apoptotic factors. The communication between these codes is crucial to fine-tune the apoptotic response and prevent unintended cell death or survival. The regulatory codes communicate with the manufacturing and signaling codes to ensure that the execution of apoptosis is well-timed and controlled.
Communication among these codes is essential to ensure proper cell operation. For instance, the manufacturing of apoptotic components must be closely regulated to prevent premature apoptosis or cell survival. Signaling pathways communicate the cellular status to initiate apoptosis only when appropriate. Regulatory codes ensure that apoptosis proceeds correctly, preventing aberrant outcomes. This intricate interplay is unlikely to have evolved in a stepwise manner, as individual components would lack functionality without the presence of the others. An incomplete manufacturing code would lead to missing apoptotic components, rendering signaling and regulatory codes meaningless. Similarly, signaling pathways without regulatory controls would lead to uncontrolled cell death or survival. These codes were likely instantiated all at once, fully operational, to ensure the coordinated and purposeful execution of apoptosis. The precise interdependencies, communication, and crosstalk among these codes point to a carefully orchestrated system that couldn't have emerged gradually through evolution. The interdependence of manufacturing, signaling, and regulatory codes strongly suggests that they were designed to work together harmoniously from the outset.
Once apoptosis is operational, what other intra and extracellular systems is it interdependent with?
Apoptosis, as a highly regulated process of programmed cell death, is interconnected with various intracellular and extracellular systems to ensure proper functioning, tissue homeostasis, and the overall health of the organism.
Intracellular Systems
Cell Cycle Control: Apoptosis interacts with the cell cycle machinery. In cases of irreparable DNA damage or cell stress, apoptosis prevents the replication and division of damaged cells.
DNA Repair Pathways: If DNA damage can be repaired, apoptosis might be averted. However, if repair mechanisms fail, apoptosis eliminates cells with potentially harmful mutations.
Cell Signaling Pathways: Apoptosis interacts with various intracellular signaling pathways, such as growth factor pathways, stress response pathways (e.g., p53), and immune signaling pathways, to integrate signals that determine whether a cell should undergo apoptosis.
Mitochondrial Function: Apoptosis involves the mitochondrial pathway, where mitochondria release pro-apoptotic factors. The health and integrity of mitochondria impact the sensitivity of cells to apoptosis.
Endoplasmic Reticulum Stress: Disruption of protein folding in the endoplasmic reticulum can trigger apoptosis, ensuring that misfolded proteins don't accumulate.
Cell Adhesion and ECM: Cells undergoing apoptosis often detach from neighboring cells and the extracellular matrix to facilitate their removal by phagocytic cells.
Extracellular Systems
Immune Response: Apoptosis plays a role in immune regulation. Dead or dying cells release signals that attract immune cells to remove cellular debris and prevent inflammation.
Phagocytosis: Apoptotic cells release "eat me" signals that attract phagocytic cells (macrophages and dendritic cells), which engulf and clear the dying cells.
Inflammation: Failure to properly clear apoptotic cells can lead to secondary necrosis, where cellular contents spill out and trigger inflammation. Timely apoptosis prevents this.
Tissue Development and Homeostasis: Apoptosis is essential for sculpting tissues during development, eliminating excess or unwanted cells. It also maintains tissue homeostasis by removing damaged or aged cells.
Cancer and Tumor Suppression: Dysregulated apoptosis can contribute to cancer development. Apoptosis acts as a fail-safe mechanism to eliminate cells with potential oncogenic mutations.
Vascular System: Apoptosis can play a role in vascular regression during development and disease. In angiogenesis, for example, excess blood vessels are pruned through apoptosis.
Neurodevelopment: Apoptosis is involved in sculpting the developing nervous system by eliminating excess neurons and establishing proper connections.
The interdependence of apoptosis with these systems highlights its role in maintaining tissue integrity, preventing disease, and contributing to the overall function and health of multicellular organisms.
1. The intricate interdependence of apoptosis with various intracellular and extracellular systems, including cell cycle control, DNA repair, signaling pathways, mitochondrial function, immune response, phagocytosis, tissue development, and others, is crucial for maintaining tissue integrity, proper immune regulation, and overall health in multicellular organisms.
2. These interdependent systems must function harmoniously and collaboratively from the outset to ensure that apoptosis serves its vital roles, including eliminating damaged cells, preventing inflammation, sculpting tissues, and maintaining homeostasis.
The seamless integration of apoptosis with these interconnected systems suggests a coherent and intentional design that facilitates the coordinated functioning of diverse cellular and physiological processes.
Conclusion: The interdependence of apoptosis with various intracellular and extracellular systems, each contributing to the health and function of the organism, strongly implies a purposeful and intricately designed setup. The simultaneous and interlocked emergence of these systems underscores a level of complexity and coordination that appears to go beyond gradual step-by-step evolution, pointing to an orchestrated design that ensures the holistic functioning and well-being of multicellular organisms.
Premise 1: The emergence, development, and operation of apoptosis involve a highly intricate and interdependent web of manufacturing, signaling, and regulatory codes and languages. These codes are irreducible and cannot function independently; they rely on each other to achieve a coherent and functional apoptotic process.
Premise 2: Communication between these codes is crucial for normal cell operation, ensuring accurate triggering, execution, and control of apoptosis.
Conclusion: Therefore, the intricate interdependencies, irreducibility, and seamless communication among manufacturing, signaling, and regulatory codes indicate a purposeful and simultaneous instantiation of these codes to orchestrate apoptosis, pointing towards intelligent design rather than a stepwise evolutionary process.
Apoptosis is a fundamental biological process of programmed cell death that plays a crucial role in shaping tissues and organs during development, maintaining tissue homeostasis, and eliminating damaged or surplus cells. Unlike necrosis, which is a chaotic and uncontrolled form of cell death, apoptosis follows a tightly regulated sequence of events. During apoptosis, cells undergo controlled dismantling in response to specific signals. These signals can originate from internal factors like DNA damage or external cues such as growth factor withdrawal. The process involves a series of distinct stages:
Initiation: Apoptosis can be triggered by various stimuli, either intrinsic (e.g., DNA damage) or extrinsic (e.g., binding of death ligands to cell surface receptors).
Signaling Pathways: Activation of signaling pathways leads to the expression or activation of specific genes and proteins that orchestrate apoptosis.
Caspase Activation: Caspases, a family of protease enzymes, play a central role in apoptosis. Initiator caspases are activated first, triggering a cascade that culminates in the activation of executioner caspases.
Cellular Changes: Apoptotic cells undergo characteristic changes, including cell shrinkage, chromatin condensation, and nuclear fragmentation.
Plasma Membrane Alterations: Phospholipids flip from the inner to the outer leaflet of the cell membrane, signaling to phagocytes that the cell is undergoing apoptosis.
Blebbing: The cell membrane forms bulges called blebs, which are eventually shed as apoptotic bodies containing cellular debris.
Phagocytosis: Phagocytes recognize apoptotic bodies through "eat me" signals on their surfaces. The phagocytes engulf and digest these bodies, preventing inflammation and tissue damage.
Resolution: Apoptotic cells are efficiently cleared, leading to minimal impact on surrounding tissues.
What is the role of apoptosis in sculpting and refining tissues during development?
Tissue Patterning and Shape Formation: Apoptosis is involved in eliminating excess cells and shaping developing tissues and organs. By selectively removing specific cells, apoptosis helps establish and refine the proper structure and shape of organs. This is particularly important in processes like limb development, where apoptosis is responsible for creating spaces between digits (interdigital spaces) and sculpting the final shape of the limb.
Digit Formation: In vertebrate limb development, apoptosis removes cells from the areas between developing digits, allowing them to separate. This process is essential for forming individual fingers or toes. The removal of cells in the interdigital regions is orchestrated by signaling pathways that activate apoptosis in a precisely coordinated manner.
Nervous System Development: Apoptosis plays a role in shaping the nervous system. During neural development, there is an initial overproduction of neurons, and apoptosis eliminates excess neurons that do not establish proper connections or synapses. This helps refine neural circuits and optimize their functionality.
Organ Development and Homeostasis: Apoptosis is involved in eliminating unwanted or abnormal cells during organ development. It helps shape organs by selectively removing cells that would otherwise disrupt the proper structure or function of the organ. Additionally, apoptosis continues to operate in adulthood to maintain tissue homeostasis by removing damaged or aged cells.
Immune System Formation: In the immune system, apoptosis is involved in shaping lymphoid organs and eliminating self-reactive immune cells. Immature immune cells undergo selection processes that involve apoptosis to ensure that only functional and non-self-reactive cells mature and become part of the immune repertoire.
Cell Number Control: Apoptosis helps regulate cell numbers in various tissues to achieve the appropriate balance between cell proliferation and cell death. This balance is essential for maintaining the overall integrity and functionality of tissues and organs.
Preventing Abnormal Development: Apoptosis acts as a quality control mechanism by eliminating cells with developmental defects, DNA damage, or other abnormalities. This prevents the propagation of genetic or cellular errors that could lead to malformation or disease.
Apoptosis serves as a precise and controlled mechanism for eliminating cells that are no longer needed or that could disrupt proper tissue and organ development. By sculpting and refining tissues, apoptosis contributes to the establishment of functional and well-organized structures in the developing organism.
How do the regulatory mechanisms of apoptosis ensure the precise elimination of specific cell populations?
The regulatory mechanisms of apoptosis ensure the precise elimination of specific cell populations through a series of tightly controlled steps and molecular interactions. These mechanisms allow cells to be targeted for elimination while minimizing the risk of collateral damage to neighboring cells. Here's how these regulatory mechanisms work:
Caspase Activation: Caspases are a group of protease enzymes that play a central role in apoptosis. They are initially present as inactive procaspases. Activation of caspases is a key step in initiating apoptosis. Activation can occur through two main pathways: the extrinsic pathway, which is initiated by death receptors on the cell surface, and the intrinsic pathway, which is activated by intracellular stress signals.
Bcl-2 Family Proteins: The Bcl-2 family of proteins includes both pro-apoptotic and anti-apoptotic members. These proteins regulate the permeability of the mitochondrial membrane and the release of cytochrome c, a trigger for caspase activation. Pro-apoptotic members promote apoptosis by inducing mitochondrial membrane permeabilization, while anti-apoptotic members inhibit apoptosis by preventing cytochrome c release.
Caspase Cascade: Activated caspases initiate a cascade of proteolytic events that lead to cell dismantling. Initiator caspases, such as caspase-8 and caspase-9, cleave and activate effector caspases, such as caspase-3. Effector caspases cleave a wide range of cellular substrates, including structural and functional proteins, resulting in cell disassembly.
Cellular Engulfment: Phagocytic cells, such as macrophages, play a role in engulfing apoptotic cells. This process, called phagocytosis or efferocytosis, prevents the release of cellular contents that could trigger inflammation and damage neighboring cells. Engulfment is facilitated by "eat-me" signals displayed on the surface of apoptotic cells and recognized by phagocytes.
Death Receptors and Ligands: In the extrinsic pathway, death receptors on the cell surface, such as Fas (CD95) and TNF receptor, bind to specific ligands. This binding triggers a signaling cascade that leads to caspase activation and apoptosis. This pathway is particularly important for immune system regulation and defense against infected or abnormal cells.
Survival Factors and Apoptotic Signals: Cells receive survival signals from their environment through growth factors and other molecules. These signals activate intracellular pathways that promote cell survival by inhibiting apoptosis. Conversely, absence of survival signals or exposure to apoptotic signals can tip the balance towards apoptosis.
Checkpoint Mechanisms: Cells have built-in checkpoints to ensure that apoptosis is activated only when appropriate. For example, DNA damage triggers the activation of p53, a tumor suppressor protein. p53 can induce cell cycle arrest to allow DNA repair, but if the damage is severe, it can activate apoptosis to prevent propagation of genetic errors.
Tissue-Specific Regulation: The regulation of apoptosis can be tissue-specific. Some cells are more sensitive to apoptosis signals due to their expression of certain proteins or the presence of specific receptors. This allows for precise elimination of particular cell populations during development, differentiation, and tissue homeostasis.
Collectively, these regulatory mechanisms ensure that apoptosis is a controlled and highly orchestrated process. The interplay between pro-apoptotic and anti-apoptotic signals, along with checks and balances, allows cells to be targeted for elimination with high specificity, contributing to the precise elimination of specific cell populations during development and tissue maintenance.
How were apoptotic pathways instantiated to balance tissue growth and removal in different organisms?
The instantiation of apoptotic pathways to balance tissue growth and removal in different organisms is a complex process that involves both conserved and organism-specific mechanisms. While the exact details can vary among species, certain core principles guide the establishment of apoptotic pathways to achieve proper tissue homeostasis:
Conserved Core Components: Many of the core components of apoptotic pathways are evolutionarily conserved across different organisms. For instance, caspases, Bcl-2 family proteins, and death receptors are present in a wide range of species. These conserved components provide a foundation for the initiation and execution of apoptosis.
Diversification of Pathways: Different organisms may have evolved specific apoptotic pathways tailored to their physiological and developmental needs. For example, the extrinsic pathway involving death receptors is more prominent in mammals, particularly in immune regulation. In contrast, the intrinsic pathway, centered around mitochondria, is a more universal mechanism present in various organisms.
Tissue-Specific Regulation: Apoptosis is often regulated in a tissue-specific manner. The expression of certain pro-apoptotic and anti-apoptotic factors can vary between tissues, allowing for selective control of apoptosis in different parts of the body. This tissue-specific regulation is crucial for achieving the proper balance between tissue growth and removal.
Adaptation to Developmental Stages: Apoptosis is involved in various developmental stages, from embryogenesis to adulthood. Different organisms have adapted their apoptotic pathways to suit the requirements of each developmental phase. For example, apoptosis during embryogenesis shapes tissue formation and organ development, while apoptosis in adult organisms contributes to tissue maintenance and removal of damaged cells.
Integration with Signaling Networks: Apoptotic pathways are integrated with other signaling networks in the cell. For instance, growth factors, survival signals, and DNA damage responses influence the balance between survival and apoptosis. The integration of apoptotic pathways with these networks allows organisms to respond dynamically to changing environmental and cellular conditions.
Fine-Tuning and Feedback: Apoptotic pathways often incorporate feedback loops and fine-tuning mechanisms. Regulatory feedback loops can enhance the precision of apoptotic responses and prevent excessive cell death. This ensures that tissue removal occurs only when needed and prevents the loss of essential cell populations.
Genetic and Functional Redundancy: Some organisms possess genetic redundancy, where multiple genes encode functionally similar proteins. This redundancy provides backup mechanisms to ensure that apoptotic pathways can function effectively even if some components are compromised.
Overall, the instantiation of apoptotic pathways in different organisms involves a combination of conserved elements and adaptive mechanisms. The diverse strategies employed by different species reflect the complexity and flexibility of apoptotic regulation across the tree of life.
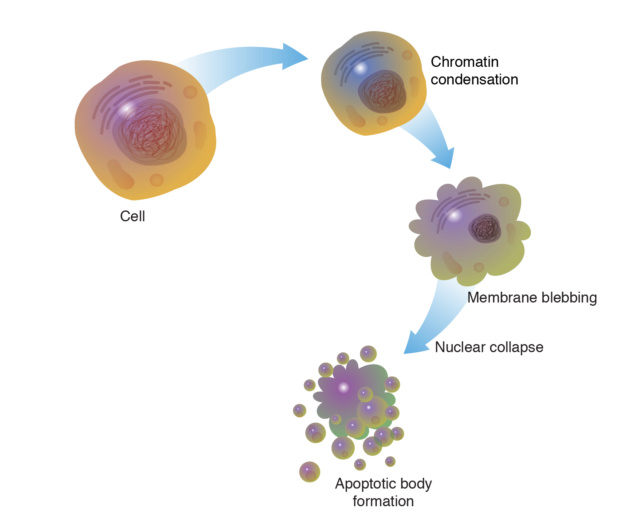
Apoptosis is critical for normal development, tissue remodeling, immune response regulation, and maintaining tissue integrity. Dysregulation of apoptosis is associated with various diseases, including cancer (where excessive cell survival occurs) and neurodegenerative disorders (where excessive cell death occurs). The complex and highly orchestrated nature of apoptosis suggests that it serves as a fine-tuned mechanism that is essential for the proper functioning of multicellular organisms. The emergence of apoptosis, a complex process involving cellular self-destruction, would have required the establishment of specific manufacturing codes and languages to orchestrate the production, assembly, and regulation of the components involved.
Appearance of apoptosis in the evolutionary timeline
The emergence of apoptosis, a highly regulated form of programmed cell death, is believed to have played a crucial role in shaping the development and complexity of multicellular organisms. While the exact timing of when apoptosis first appeared in the evolutionary timeline is not definitively known, researchers have proposed theories based on comparative studies and evidence from various organisms.
Early Single-Celled Organisms (Prokaryotes): It is unlikely that apoptosis, as understood in multicellular organisms, would have existed in early single-celled organisms like prokaryotes. The cellular complexity and mechanisms necessary for programmed cell death as seen in higher organisms would not have been present.
Emergence of Eukaryotes: With the supposed evolution of eukaryotic cells, which possess more complex internal structures and organelles, the potential for controlled cell death mechanisms might have increased. However, at this stage, any form of cell death would have been more similar to necrosis, a less regulated process compared to apoptosis.
Multicellular Organisms: The transition to multicellularity would have introduced new challenges related to cell differentiation, tissue development, and maintaining proper cell numbers. Apoptosis might have started to emerge as a potential mechanism to eliminate excess or damaged cells, refine tissue structures, and aid in proper development. Rudimentary forms of programmed cell death would have been present even in early multicellular organisms.
Invertebrates: As organisms became more complex, particularly within the animal kingdom, it is speculated that apoptosis would have become more sophisticated. Invertebrates would have employed some form of programmed cell death to assist in shaping tissues, organs, and structures during development. However, the molecular mechanisms and regulation would have been less intricate than in more advanced organisms.
Vertebrates: With the appearance of vertebrates and the evolution of intricate organ systems, it's suggested that apoptosis would have become more refined and tightly regulated. This process would have played critical roles in various aspects including organogenesis, immune system development, tissue repair, and the removal of potentially harmful or unnecessary cells.
Evolution of Adaptive Immunity: The emergence of the adaptive immune system in vertebrates would have supposedly introduced a necessity for precise cell death mechanisms to eliminate unwanted immune cells and prevent autoimmune responses. Apoptosis might have played a central role in maintaining the balance of the immune system.
De Novo Genetic Information to instantiate apoptosis
The emergence of apoptosis, a complex and regulated process of programmed cell death, would have required the addition of specific genetic information to enable its functions.
Apoptosis Initiation Genes: New genetic elements encoding proteins responsible for initiating apoptosis would need to evolve. These proteins, often activated in response to cellular stress or signals, would trigger the apoptotic cascade.
Caspase Genes: Caspases are a family of protease enzymes crucial for executing apoptosis. The evolution of apoptosis would require the emergence of genes encoding various types of caspases, each with distinct roles in apoptosis progression.
Cell Death Regulatory Proteins: Proteins that regulate the balance between pro-apoptotic and anti-apoptotic signals would need to evolve. These proteins would control the decision-making process of whether a cell undergoes apoptosis.
Apoptotic Signaling Pathways: New genetic information would be necessary to establish the intricate signaling pathways that transmit pro-apoptotic and anti-apoptotic signals, leading to the activation of caspases and subsequent cell death.
DNA Fragmentation Genes: During apoptosis, DNA is fragmented into smaller pieces. Genes responsible for this fragmentation process would need to evolve, ensuring the controlled degradation of the cell's genetic material.
Cellular Membrane Alteration Genes: Changes in the cell's membrane structure and properties are common in apoptosis. Genes encoding proteins responsible for altering the membrane would be essential.
Phagocytosis Recognition Genes: In multicellular organisms, phagocytes engulf apoptotic cells. Genes encoding surface molecules on apoptotic cells that signal for their recognition and engulfment by phagocytes would need to emerge.
Regulation of Apoptosis Timing: The evolution of apoptosis would require genetic mechanisms to regulate the timing of cell death, ensuring that it occurs at the right stage of development or in response to appropriate signals.
Apoptosis in Single-Celled Organisms: In prokaryotes or unicellular eukaryotes, apoptosis-like mechanisms could involve genes that trigger self-destruction under certain conditions, such as nutrient depletion or environmental stress.
The emergence of apoptosis would require the simultaneous addition of multiple genes and regulatory elements to form a functional and controlled process of programmed cell death. The complexity and coordination involved in apoptosis suggest that such genetic information would need to be instantiated and integrated into existing genetic systems to achieve its crucial functions.
Manufacturing codes and languages employed to instantiate apoptosis
Exempting genetic codes and languages, the emergence of apoptosis would have required the establishment of intricate manufacturing codes and languages within cells. These codes and languages encompass a series of coordinated biochemical processes that lead to the controlled dismantling and elimination of cells.
Cellular Signaling Network: Cells would need to develop a sophisticated network of signaling pathways that sense both internal and external cues. These pathways would translate specific triggers into appropriate responses that initiate apoptosis.
Protein Activation and Inactivation: Intricate protein interactions would be established, involving activation and inactivation of key enzymes and factors. This would require the development of mechanisms to ensure precise timing and regulation.
Enzyme Cascades: Manufacturing codes would involve creating enzyme cascades, like the caspase cascade in apoptosis. These cascades amplify signals, ensuring a rapid and coordinated response throughout the cell.
Protein Modifications: The emergence of new manufacturing codes would facilitate various protein modifications, including phosphorylation, ubiquitination, and proteolytic cleavage. These modifications would control protein functions within the apoptosis pathway.
Membrane Remodeling: Manufacturing languages would govern the reorganization of cellular membranes during apoptosis, leading to characteristic changes such as blebbing and externalization of phospholipids.
Cellular Morphological Changes: The process of apoptosis involves specific changes in cell shape and structure. Manufacturing codes would be needed to coordinate these changes, such as cell shrinkage and chromatin condensation.
Phagocytosis Coordination: Apoptotic bodies, the remnants of apoptotic cells, must be recognized and engulfed by phagocytes to prevent inflammation. Manufacturing languages would regulate the signals that facilitate this recognition and engulfment.
Removal of Cellular Debris: Efficient removal of cellular debris resulting from apoptosis would necessitate manufacturing codes for the disassembly and recycling of cellular components.
Tissue Repair and Homeostasis: Manufacturing languages would ensure that apoptosis contributes to tissue repair and homeostasis, avoiding excessive cell loss and enabling the replacement of damaged cells.
Integration with Other Processes: The codes for apoptosis would need to integrate with other cellular processes, such as inflammation and cell survival pathways, to maintain balanced responses and prevent excessive tissue damage. The development of these manufacturing codes and languages would require a comprehensive understanding of cell biology, biochemistry, and cellular interactions. The interplay and synchronization of these processes would be vital to ensure proper apoptosis execution while avoiding unintended consequences. The emergence of apoptosis would represent a finely tuned system that contributes to the overall health and functionality of multicellular organisms.
Epigenetic Regulatory Mechanisms necessary to be instantiated for Apoptosis
To instantiate the development of apoptosis from scratch, several epigenetic regulatory mechanisms would need to be created and subsequently employed. These mechanisms involve intricate interactions between different cellular systems to ensure proper regulation and functioning of apoptosis.
DNA Methylation: Epigenetic marks involving DNA methylation could be established to regulate the expression of genes related to apoptosis. Methylation of promoter regions could silence or activate specific apoptotic genes.
Histone Modifications: Various histone modifications, such as acetylation, methylation, and phosphorylation, would need to be established to influence the accessibility of chromatin regions associated with apoptotic genes.
Non-Coding RNAs: Non-coding RNAs, like microRNAs and long non-coding RNAs, could emerge to fine-tune the expression of apoptotic genes by interacting with mRNA transcripts or chromatin-modifying complexes.
Systems Involved in Instantiating and Employing Apoptotic Regulation
Transcriptional Machinery: The core transcriptional machinery, including RNA polymerases and transcription factors, would need to be in place to enable the transcription of apoptotic genes based on the epigenetic marks.
Chromatin Remodeling Complexes: Complexes responsible for chromatin remodeling would play a crucial role in modulating the accessibility of apoptotic gene promoters and enhancers, guided by epigenetic modifications.
RNA Processing Machinery: The emergence of a functional RNA processing machinery would be necessary for the production and regulation of non-coding RNAs that control apoptotic gene expression.
Systems Collaborating to Maintain Apoptotic Regulation
DNA Repair and Replication Systems: These systems would collaborate to ensure the faithful inheritance of epigenetic marks during cell division, maintaining the proper epigenetic regulation of apoptotic genes.
Cell Signaling Pathways: Cellular signaling pathways that respond to various cues, such as stress or developmental signals, would work in conjunction with epigenetic mechanisms to initiate or suppress apoptosis as needed.
Apoptotic Pathways: Once initiated, the apoptotic pathways themselves would engage in a feedback loop to reinforce or inhibit apoptotic signals, further shaping the outcome of the process.
The instantiation and employment of epigenetic regulation for apoptosis would require a coordinated effort between multiple cellular systems. The DNA methylation, histone modification, and non-coding RNA systems would work together to establish the proper epigenetic marks on apoptotic genes. Subsequently, transcriptional machinery, chromatin remodeling complexes, and RNA processing machinery would collaborate to translate these marks into appropriate gene expression patterns. Maintenance of this regulation would involve systems that ensure accurate epigenetic inheritance during cell division and the integration of signals from various cellular pathways to determine whether apoptosis should be initiated or inhibited. Overall, these interdependent systems would contribute to the proper development and functioning of apoptosis.
Signaling Pathways necessary to create, and maintain apoptosis
The emergence of apoptosis from scratch would require the creation and subsequent involvement of specific signaling pathways that coordinate and regulate the process of programmed cell death. These signaling pathways would be interconnected, and interdependent, and would crosstalk with each other and with other biological systems to ensure proper apoptotic regulation.
Intrinsic Apoptotic Pathway
Activation Trigger: Cellular stress, DNA damage, or other internal factors.
Pathway: Mitochondrial outer membrane permeabilization (MOMP) releases cytochrome c, activating caspases.
Crosstalk: Interacts with anti-apoptotic Bcl-2 family proteins that counteract MOMP.
Connection to Other Systems: Responds to DNA damage and stress signals, integrates with cell cycle checkpoints, and engages DNA repair systems.
Extrinsic Apoptotic Pathway
Activation Trigger: External signals, such as binding of death ligands (e.g., Fas ligand) to death receptors (e.g., Fas receptor).
Pathway: Ligand-receptor binding activates caspase-8, initiating downstream caspase cascade.
Crosstalk: Inhibitory proteins like FLIP can block caspase-8 activation.
Connection to Other Systems: Interacts with immune responses and inflammation pathways, and integrates signals from death ligands.
Caspase Activation Pathway
Activation Trigger: Initiators such as caspase-8 (extrinsic) or caspase-9 (intrinsic) are activated.
Pathway: Initiators cleave and activate effector caspases (caspase-3, -6, -7) leading to cell dismantling.
Crosstalk: Inhibitors like XIAP can directly block effector caspases.
Connection to Other Systems: Links to DNA repair systems and cellular stress responses.
PI3K/AKT Survival Pathway
Activation Trigger: Growth factors and survival signals.
Pathway: Activation of PI3K and AKT promotes cell survival and inhibits apoptosis by phosphorylating pro-apoptotic factors.
Crosstalk: Counteracted by PTEN, which opposes PI3K.
Connection to Other Systems: Integrates with cell growth, proliferation, and nutrient sensing pathways.
p53 Signaling Pathway
Activation Trigger: DNA damage or stress signals.
Pathway: Activation of p53 leads to transcription of pro-apoptotic genes (e.g., PUMA, Bax).
Crosstalk: Interaction with MDM2 regulates p53 stability.
Connection to Other Systems: Coordinates DNA repair mechanisms, cell cycle arrest, and apoptosis in response to genotoxic stress.
NF-κB Pathway
Activation Trigger: Inflammation, immune responses, and stress signals.
Pathway: Activation of NF-κB promotes cell survival and inhibits apoptosis by regulating the expression of anti-apoptotic genes.
Crosstalk: IKK complex phosphorylation controls NF-κB activation.
Connection to Other Systems: Links apoptosis to inflammation and immune responses.
These signaling pathways are interconnected, often converging and diverging to fine-tune the apoptotic response based on cellular conditions. They are interdependent, with some pathways directly regulating others to maintain proper cell fate decisions. Crosstalk between pathways allows cells to integrate various signals, ensuring a balanced and appropriate apoptotic response. Additionally, these pathways interact with broader biological systems, including immune responses, DNA repair, and cell cycle control, to create a sophisticated network that orchestrates the emergence and regulation of apoptosis.
Regulatory codes necessary for maintenance and operation of apoptosis
The emergence, maintenance, and operation of apoptosis would require the instantiation of regulatory codes and languages that ensure proper coordination, execution, and control of the process. These regulatory codes and languages would involve intricate interactions between various molecules and cellular components to regulate apoptosis.
Transcriptional Regulatory Code: The activation of specific genes associated with apoptosis, including pro-apoptotic and anti-apoptotic factors, would require a transcriptional regulatory code. Transcription factors such as p53, NF-κB, and STATs could bind to specific DNA sequences, promoting or inhibiting the expression of apoptotic genes.
Post-Translational Modification Code: Phosphorylation, acetylation, ubiquitination, and other post-translational modifications would be involved in modulating the activity of apoptotic regulators. For instance, phosphorylation of Bcl-2 family proteins can affect their pro-apoptotic or anti-apoptotic functions.
Protein Interaction Networks: Regulatory codes would govern the interactions between proteins involved in apoptosis. These interactions would dictate the formation of protein complexes, such as apoptosome assembly, which activate caspase cascades.
Inhibitory and Activating Signals: The language of signaling molecules, including growth factors, cytokines, and ligands, would determine whether apoptosis is initiated or inhibited. These signals would engage receptors and trigger downstream signaling events.
Feedback Loops: Regulatory codes could involve feedback loops that sense cellular stress, DNA damage, or other triggers and modulate the apoptotic response accordingly. These loops could fine-tune the process to ensure an appropriate response.
Epigenetic Regulation: Epigenetic marks, such as DNA methylation and histone modifications, might be established to regulate the accessibility of apoptotic genes. Epigenetic changes could be involved in maintaining proper balance between pro-apoptotic and anti-apoptotic factors.
Protein Stability Control: Regulatory codes would control the stability of key apoptosis regulators. For instance, proteins like p53 are tightly regulated through degradation mechanisms.
Feedback Inhibition: Inhibitory factors, such as caspase inhibitors (cIAPs) and anti-apoptotic Bcl-2 proteins, would be part of the regulatory language to prevent excessive or premature apoptosis.
Cell-Cell Communication: Apoptosis can be influenced by signals from neighboring cells. Communication between cells through death ligands and their receptors (e.g., Fas/FasL) would be an important aspect of the regulatory code.
Feedback to Other Cellular Processes: The apoptotic regulatory code would interact with other cellular processes like DNA repair, cell cycle control, and immune responses to ensure proper integration of signals and responses.
These regulatory codes and languages would be intertwined, forming a complex network that governs the initiation, execution, and modulation of apoptosis. The balance and coordination of these codes would be crucial to ensure the appropriate elimination of cells and maintenance of tissue homeostasis.
Is there scientific evidence supporting the idea that apoptosis is brought about by the process of evolution?
Apoptosis, or programmed cell death, is a complex and highly regulated process that plays a crucial role in maintaining tissue homeostasis, development, and immune responses.
Functional Complexity: Apoptosis involves a series of intricate steps, including cellular signaling, organelle fragmentation, DNA degradation, and cell engulfment by neighboring cells or phagocytes. These steps require multiple interacting components to function properly.
Regulation and Signaling: Apoptosis is tightly regulated to ensure that it occurs when necessary and doesn't harm the organism. It requires a sophisticated signaling network involving pro-apoptotic and anti-apoptotic factors. This network must be fully operational to prevent accidental cell death or survival.
Cellular Communication: Apoptosis often involves communication between cells to signal the need for cell death. The evolution of the ability to send and receive such signals, and the corresponding cellular responses, is complex.
Selective Advantage: For an evolutionary process to proceed, intermediate stages must offer some selective advantage to the organism. The early stages of apoptosis, without the complete set of regulatory mechanisms, could potentially lead to harmful outcomes, such as uncontrolled cell death or immune system dysfunction.
Interdependence of Components: Apoptosis requires the coordinated activity of various proteins, enzymes, and regulatory factors. The evolution of these components in a stepwise manner might not offer any functional advantage until the entire system is in place.
Conservation and Complexity: Apoptosis is a highly conserved process found across diverse organisms. This suggests that the components and mechanisms involved are fundamental to life. The complex nature of apoptosis raises questions about how these components could have evolved incrementally.
Cellular Consequences: Incomplete or partially functional apoptotic pathways could have detrimental effects on an organism. If intermediate stages led to excessive cell death or impaired cell survival, they might be disadvantageous and not favored by natural selection.
Integration with Other Systems: Apoptosis is interconnected with various cellular processes, including cell proliferation, immune responses, and tissue development. Evolution would need to consider how apoptosis fits into these existing systems.
Irreducibility and Interdependence of the systems to instantiate and operate apoptosis
The emergence, development, and operation of apoptosis involve a highly intricate and interdependent web of manufacturing, signaling, and regulatory codes and languages. These codes are irreducible and cannot function independently; they rely on each other to achieve a coherent and functional apoptotic process. Communication between these codes is crucial for normal cell operation, ensuring that apoptosis is triggered, executed, and controlled accurately. It becomes apparent that these interdependencies point to a simultaneous and purposeful instantiation of these codes.
Manufacturing Codes and Languages: The manufacturing codes are responsible for producing the intricate molecular machinery required for apoptosis, including the components of the apoptosome, caspases, and their regulators. These codes are interdependent, as without the manufacturing of these specific components, apoptosis cannot be executed effectively. The coordination between the manufacturing codes ensures that the required proteins and structures are produced accurately and in the right quantities.
Signaling Pathways: Signaling pathways, like those involving death receptors and their ligands, guide the initiation and propagation of apoptotic signals. These pathways communicate with each other through crosstalk, amplifying or dampening the apoptotic response based on cellular context. For example, interactions between the extrinsic and intrinsic pathways ensure a balanced response. Without these interconnected signaling pathways, the decision to initiate apoptosis or not would lack proper integration and coordination.
Regulatory Codes and Languages: Regulatory codes control the activation, inhibition, and modulation of apoptosis. These codes are interconnected, forming a delicate balance between pro-apoptotic and anti-apoptotic factors. The communication between these codes is crucial to fine-tune the apoptotic response and prevent unintended cell death or survival. The regulatory codes communicate with the manufacturing and signaling codes to ensure that the execution of apoptosis is well-timed and controlled.
Communication among these codes is essential to ensure proper cell operation. For instance, the manufacturing of apoptotic components must be closely regulated to prevent premature apoptosis or cell survival. Signaling pathways communicate the cellular status to initiate apoptosis only when appropriate. Regulatory codes ensure that apoptosis proceeds correctly, preventing aberrant outcomes. This intricate interplay is unlikely to have evolved in a stepwise manner, as individual components would lack functionality without the presence of the others. An incomplete manufacturing code would lead to missing apoptotic components, rendering signaling and regulatory codes meaningless. Similarly, signaling pathways without regulatory controls would lead to uncontrolled cell death or survival. These codes were likely instantiated all at once, fully operational, to ensure the coordinated and purposeful execution of apoptosis. The precise interdependencies, communication, and crosstalk among these codes point to a carefully orchestrated system that couldn't have emerged gradually through evolution. The interdependence of manufacturing, signaling, and regulatory codes strongly suggests that they were designed to work together harmoniously from the outset.
Once apoptosis is operational, what other intra and extracellular systems is it interdependent with?
Apoptosis, as a highly regulated process of programmed cell death, is interconnected with various intracellular and extracellular systems to ensure proper functioning, tissue homeostasis, and the overall health of the organism.
Intracellular Systems
Cell Cycle Control: Apoptosis interacts with the cell cycle machinery. In cases of irreparable DNA damage or cell stress, apoptosis prevents the replication and division of damaged cells.
DNA Repair Pathways: If DNA damage can be repaired, apoptosis might be averted. However, if repair mechanisms fail, apoptosis eliminates cells with potentially harmful mutations.
Cell Signaling Pathways: Apoptosis interacts with various intracellular signaling pathways, such as growth factor pathways, stress response pathways (e.g., p53), and immune signaling pathways, to integrate signals that determine whether a cell should undergo apoptosis.
Mitochondrial Function: Apoptosis involves the mitochondrial pathway, where mitochondria release pro-apoptotic factors. The health and integrity of mitochondria impact the sensitivity of cells to apoptosis.
Endoplasmic Reticulum Stress: Disruption of protein folding in the endoplasmic reticulum can trigger apoptosis, ensuring that misfolded proteins don't accumulate.
Cell Adhesion and ECM: Cells undergoing apoptosis often detach from neighboring cells and the extracellular matrix to facilitate their removal by phagocytic cells.
Extracellular Systems
Immune Response: Apoptosis plays a role in immune regulation. Dead or dying cells release signals that attract immune cells to remove cellular debris and prevent inflammation.
Phagocytosis: Apoptotic cells release "eat me" signals that attract phagocytic cells (macrophages and dendritic cells), which engulf and clear the dying cells.
Inflammation: Failure to properly clear apoptotic cells can lead to secondary necrosis, where cellular contents spill out and trigger inflammation. Timely apoptosis prevents this.
Tissue Development and Homeostasis: Apoptosis is essential for sculpting tissues during development, eliminating excess or unwanted cells. It also maintains tissue homeostasis by removing damaged or aged cells.
Cancer and Tumor Suppression: Dysregulated apoptosis can contribute to cancer development. Apoptosis acts as a fail-safe mechanism to eliminate cells with potential oncogenic mutations.
Vascular System: Apoptosis can play a role in vascular regression during development and disease. In angiogenesis, for example, excess blood vessels are pruned through apoptosis.
Neurodevelopment: Apoptosis is involved in sculpting the developing nervous system by eliminating excess neurons and establishing proper connections.
The interdependence of apoptosis with these systems highlights its role in maintaining tissue integrity, preventing disease, and contributing to the overall function and health of multicellular organisms.
1. The intricate interdependence of apoptosis with various intracellular and extracellular systems, including cell cycle control, DNA repair, signaling pathways, mitochondrial function, immune response, phagocytosis, tissue development, and others, is crucial for maintaining tissue integrity, proper immune regulation, and overall health in multicellular organisms.
2. These interdependent systems must function harmoniously and collaboratively from the outset to ensure that apoptosis serves its vital roles, including eliminating damaged cells, preventing inflammation, sculpting tissues, and maintaining homeostasis.
The seamless integration of apoptosis with these interconnected systems suggests a coherent and intentional design that facilitates the coordinated functioning of diverse cellular and physiological processes.
Conclusion: The interdependence of apoptosis with various intracellular and extracellular systems, each contributing to the health and function of the organism, strongly implies a purposeful and intricately designed setup. The simultaneous and interlocked emergence of these systems underscores a level of complexity and coordination that appears to go beyond gradual step-by-step evolution, pointing to an orchestrated design that ensures the holistic functioning and well-being of multicellular organisms.
Premise 1: The emergence, development, and operation of apoptosis involve a highly intricate and interdependent web of manufacturing, signaling, and regulatory codes and languages. These codes are irreducible and cannot function independently; they rely on each other to achieve a coherent and functional apoptotic process.
Premise 2: Communication between these codes is crucial for normal cell operation, ensuring accurate triggering, execution, and control of apoptosis.
Conclusion: Therefore, the intricate interdependencies, irreducibility, and seamless communication among manufacturing, signaling, and regulatory codes indicate a purposeful and simultaneous instantiation of these codes to orchestrate apoptosis, pointing towards intelligent design rather than a stepwise evolutionary process.