Could the laws of physics and fine-tune parameters of the universe change, or are they bound to physical necessity?
https://reasonandscience.catsboard.com/t3152-could-the-laws-of-physics-change-or-are-they-bound-to-physical-necessity
1. If the laws of physics can change, then the fact that they are set to permit a life-permitting universe demands an explanation.
2. If they cannot change, then they are due to physical necessity, and invoking a lawgiver, who did set them up is not necessary.
3. There are infinite possible ways that the values fundamental constants of the standard models could have been chosen.
4. The laws of physics can change, therefore the fact that they are set up to instantiate a life-permitting universe is best explained by a lawgiver. That lawgiver is God.
Leonard Susskind The Cosmic Landscape: String Theory and the Illusion of Intelligent Design 2006, page 100
By varying the Higgs field, we can add diversity to the world; the laws of nuclear and atomic physics will also vary. A physicist from one region would not entirely recognize the Laws of Physics in another. But the variety inherent in the variations of the Higgs field is very modest. What if the number of variable fields were many hundreds instead of just one? This would imply a multidimensional Landscape, so diverse that almost anything could be found. Then we might begin to wonder what is not possible instead of what is. As we will see this is not idle speculation.
https://3lib.net/book/2472017/1d5be1
The Friedmann equations for the evolution of space predicted the big-bang singularity. General relativity theory predicts a boundary at the big bang, in that the laws of physics break down, and space and time shrink to nothing. That means that the laws of physics had to be instantiated at the Big Bang. As the universe cooled after the Big Bang, symmetries were spontaneously broken, ‘phase transitions’ took place, and discontinuous changes occurred in the values of various physical parameters (e.g., in the strength of certain fundamental interactions, or in the masses of certain species of particle).
[The Lord God] is eternal and infinite, omnipotent and omniscient, that is, he endures from eternity to eternity, and he is present from infinity to infinity; he rules all things, and he knows all things that happen or can happen.
—Isaac Newton, General Scholium to the Principia (1726) Robert Boyle uses the clockwork metaphor to argue for both divine transcendence and the radical contingency of creation. 8
Marc Lange: Could the Laws of Nature Change?* (January 2008)
The natural laws are traditionally characterized as ‘eternal’, ‘fixed’, and ‘immutable’. Is the laws’ unchanging character a metaphysical necessity? If so, then in any possible world, there are exactly the same laws at all times (though presumably there are different laws in different possible worlds).2 That there actually are exactly the same laws at all times is then a consequence of what it is for a truth to be a law of nature. On the other hand, if the laws’ unchanging character is not a metaphysical necessity, then even if in fact there have always been and will always be exactly the same laws, this fact is metaphysically contingent.
As the universe cooled after the Big Bang, symmetries were spontaneously broken, ‘phase transitions’ took place, and discontinuous changes occurred in the values of various physical parameters (e.g., in the strength of certain fundamental interactions, or in the masses of certain species of particle).
One usually assumes that the current laws of physics did not apply [in the period immediately following the Big Bang]. They took hold only after the density of the universe dropped below the so-called Planck density, which equals 1094 grams per cubic centimeter. Lewis’s account entails the laws’ immutability only because a certain parameter in the account has been set to ‘the universe’s entire history’. That parameter could be set differently.
https://philosophy.unc.edu/wp-content/uploads/sites/122/2013/10/Laws-of-nature-change.pdf
My comment: This is a very important admission by the author: The parameter could be set differently. That means, there is no physical restriction or necessity that entails that the parameter could only have the one that is actualized. Since that is so, the question arises: What instantiated the life-permitting parameters? There are two possibilities: Luck, or a Lawgiver. I go with the latter.
KATE BECKER: Are the Laws of Physics Really Universal? OCTOBER 21, 2015
As far as physicists can tell, the cosmos has been playing by the same rulebook since the time of the Big Bang. But could the laws have been different in the past, and could they change in the future?
A scalar field, Carroll explains , is any quantity that has a unique value at every point in space-time. The celebrity-du-jour scalar field is the Higgs, but you can also think of less exotic quantities, like temperature, as scalar fields, too. A yet-undiscovered scalar field that changes very slowly could continue to evolve even billions of years after the Big Bang—and with it, the so-called constants of nature could evolve, too.
https://www.philosophytalk.org/shows/could-laws-physics-ever-change
Clara Moskowitz: 2 Accelerators Find Particles That May Break Known Laws of Physics September 9, 2015
The LHC and the Belle experiment have found particle decay patterns that violate the Standard Model of particle physics, confirming earlier observations at the BaBar facility. Two experiments have observed particles misbehaving in ways not predicted by any known laws of physics. The eyebrow-raising results come from the LHCb experiment at the Large Hadron Collider (LHC) in Switzerland and the Belle experiment at the High Energy Accelerator Research Organization (KEK) in Japan.
Massimo Pigliucci, professor of philosophy at the City University of New York, argues that the word “law” (in the context of a “law of physics” or “law of nature”) is problematic. He maintains that the concept of law raises the question of who or what decided the law to be that way.
In order to have a life-permitting universe, that would entail a “cosmic selection” to fine-tune the universe that we have today. Or an ensemble of multiverses would generate all kinds of universes, amongst them, ours, life-permitting.
https://www.scientificamerican.com/article/2-accelerators-find-particles-that-may-break-known-laws-of-physics1/
Andy Boyd CONSTANTS OF NATURE
What really makes the fine structure constant amazing, as Feynman and others realized, is that if it was somehow even a tiny bit different, the universe we experience wouldn't be the same. In particular, human life would not have evolved. For such a number to come out of thin air is unsettling to say the least. While we understand where some of the constants of nature come from, many seem completely arbitrary, and a better explanation of their origin remains one of the Holy Grails of modern physics.
https://www.uh.edu/engines/epi3149.htm
Philip Ball Can the laws of physics change? 28th March 2012
If Star Trek taught us one thing, it is that “ye cannae change the laws of physics”. That is certainly how scientists generally seem to regard their various laws and constants. No sooner has someone discovered a new “law” than it acquires quasi-legal status, with violations implicitly prohibited. No sooner has someone defined a “constant” than it is assumed to be just that, never varying at different times or places.
And yet, who says so? Why should we assume that a particular mass always exerts the same gravity, or that an atom always has the same mass anyway? After all, one of the most revelatory theories of modern physics, special relativity, showed that the length of a centimetre and the duration of a second can change depending on how fast you are moving. And look at the recent excitement about experiments hinting (and then dismissing due to a faulty connection) that particles called neutrinos could travel faster than the speed of light.
There is no principle of physics that says physical laws or constants have to be the same everywhere and always. This could drive you a bit mad. How can you possibly do science if the rules keep changing? But if you are a scientist, you have to face up to that possibility – and you have a responsibility to check out if it is true.
This brings us to the apparent Lewis Carroll absurdity of a new paper setting out to test if a constant central to quantum physics, called Planck’s constant or h, is really as it says. The fact that it is, as far as we can tell – there does not seem many points in manufacturing suspense here – means that this is not likely to grab any headlines. It sounds a bit like proving that Paris has not moved to China.
Precision timing
But this is an experiment that is significant not so much for what has been found as for the fact that it was done at all. After all, testing the constancy of a constant means going to extraordinary lengths in terms of precision measurements.
To test whether Planck’s constant is really constant, Makan Mohageg and graduate student James Kentosh of California State University in Northridge turned to the same GPS systems that help drivers find their way home. GPS relies on the most accurate timing devices we currently possess: atomic clocks. These count the passage of time according to frequency of the radiation that atoms emit when their electrons jump between different energy levels.
Why go to all this bother? The point is that the researchers did not just pick on a random constant. Planck’s constant is in effect the number that launched the field of quantum physics. In 1900 the German scientist Max Planck proposed h as a measure of the size of energy “packets”, or quanta, into which light is divided. Planck said that a light quantum has an amount of energy equal to the frequency of the light multiplied by h. Planck introduced this “quantum” hypothesis of light as a mathematical trick to get his equations to work out. But Albert Einstein argued five years later that the trick must be taken literally: light really is chopped up into these discrete packets of energy.
Disturbance in the universe?
The reason why Kentosh and Mohageg asked whether h is really constant, however, is not just because it is a central number for modern physics, but because h also appears in the expression for another fundamental constant, called the fine-structure constant. This measures the strength of interactions between light and matter, or equivalently, how strong electrical and magnetic forces are. It can be expressed as a combination of three constants: the charge on an electron, the speed of light, and h.
And here is the crux: some scientists have suggested that the fine structure constant might not be constant, but could vary over time and space. In 1999, a team of astronomers using a telescope in Hawaii reported that measurements of light absorbed by very distant galaxy-like objects in space called quasars – which are so far away that we see them today as they looked billions of years ago – suggest that the value of the fine-structure constant was once slightly different from what it is today.
That claim was controversial, and still unproven. But if true, it must mean that at least one of the three fundamental constants that constitute it must vary.
Location, location, location
Kentosh and Mohageg fixed on h, and specifically on whether h depends on where (not when) you measure it. If h changes from place to place, so do the frequencies, and thus the “ticking rate”, of atomic clocks. And any dependence of h on location would translate as a tiny timing discrepancy between different GPS clocks.
So, what did they discover? Well, if there is any difference in h it would have to be really tiny. After careful analysis of the data from seven highly stable GPS satellites, Kentosh and Mohageg conclude that h is identical at different locations to an accuracy of seven parts in a thousand. In other words, if h were a one-metre measuring stick, two sticks in different places anywhere in the world do not differ by more than seven millimetres.
Spotting this variation of less than 1% in measuring sticks might be easy, spotting this in an exceedingly tiny number like Planck’s constant, which is 0.000000000000000000000000000000000662606957 joule seconds, demands the type of extreme accuracy of measurement that is most likely beyond the capabilities of our most accurate atomic clocks. At this point, however, we can feel reassured that there is no reason to suspect that this particular aspect of physics shifts between, say, London and Beijing – or indeed, between our galaxy and the next one.
https://www.bbc.com/future/article/20120329-can-the-laws-of-physics-change
New findings suggest laws of nature not as constant as previously thought April 27, 2020
Four new measurements of light emitted from a quasar 13 billion light-years away reaffirm past studies that have measured tiny variations in the fine structure constant.
https://www.sciencedaily.com/releases/2020/04/200427102544.htm
What is the difference between classical physics and quantum physics?
The electron, the proton, and the quark are all entities within the realm of particle hence quantum physics. All three carry an electrical charge. The electric charge of the proton is exactly equal and the opposite of the electric charge on the electron, despite the proton being 1836 times more massive. There’s no set-in-concrete theoretical reason why this should be so. It cannot be determined from first principles, only experimentally measured.
An electron has a negative charge exactly equal to and opposite to that of a proton. Note: the charge is exactly equal, even though the proton has a far greater mass than the electron (some 2000 times heavier in fact, not that there has to be of necessity any relationship between mass and charge).
Now that’s strange since the electron is a fundamental particle but the positively charged proton is a composite particle, made up of a trio of quarks (as is the neutron with no net charge). The proton has two quarks each with a positive 2/3rds charge (up quark) and one quark with a negative 1/3rd charge (down quark) for an overall balance of one positive charge. (The neutron, on the other hand, has one up quark with a positive 2/3rds charge and two down quarks each with a negative 1/3rd charge, for an overall balance of zero charge – neither positive nor negative.)
Now you might suggest that an electron might be a fusion of a trio of down quarks, each with a negative 1/3rd charge, except the electron, again, isn’t a composite particle, and the mass is all wrong for that scenario. If an electron were a composite of a trio of down quarks, each with a minus 1/3rd charge, the electron would be thirty times more massive than it is – not something particle physicists would fail to take notice of.
Further, the force particle that governs the electron is the photon; that which governs the quarks inside the proton and the neutron is the gluon, which further differentiates the two things – quarks and electrons. In any event, if you could have a composite particle of a trio of negative 1/3rd down quarks, if that were the case, and it is the case, and it’s called the Negative Delta, you’d also need a composite particle that’s the fusion of a trio of positive 2/3rds up quarks for an overall charge of plus two. To the best of my knowledge, there is only one such critter in the particle zoo and it’s called the Doubly Positive Delta. I’m sure you’ve never heard of these Delta particles, which goes to show how much bearing or impact they have on life, the Universe, and everything.
http://www.esalq.usp.br/lepse/imgs/conteudo_thumb/What-is-the-difference-between-classical-physics-and-quantum-physics.pdf
Frank Wilczek: Asymptotic freedom: From paradox to paradigm 2005 Jun 8
In the early 1960s, Murray Gell-Mann and George Zweig made a great advance in the theory of the strong interaction by proposing the concept of quarks. If you imagined that hadrons were not fundamental particles, but rather that they were assembled from a few more basic types, the quarks, patterns clicked into place. The dozens of observed hadrons could be understood, at least roughly, as different ways of putting together just three kinds (“flavors”) of quarks. You can have a given set of quarks in different spatial orbits, or with their spins aligned in different ways. The energy of the configuration will depend on these things, and so there will be a number of states with different energies, giving rise to particles with different masses, according to m = E/c2.
My comment: There is no physical constraint that dictates the composition of a proton, or neutron. But it is precisely the composition that is, which permits the existence of atoms, and a life-permitting universe.
The electron, the proton, and the quark are all entities within the realm of particle hence quantum physics. All three carry an electrical charge. All three have mass. The electric charge of the proton is exactly equal and the opposite of the electric charge on the electron, despite the proton being 1836 times more massive. There’s no set-in-concrete theoretical reason why this should be so. It cannot be determined from first principles, only experimentally measured. The proton is a composite particle, made up of a trio of quarks (as is the neutron with no net charge). The proton has two quarks each with a positive 2/3rds charge (up quark) and one quark with a negative 1/3rd charge (down quark) for an overall balance of one positive charge. (The neutron, on the other hand, has one up quark with a positive 2/3rds charge and two down quarks each with a negative 1/3rd charge, for an overall balance of zero charge – neither positive nor negative.)
https://www.ncbi.nlm.nih.gov/pmc/articles/PMC1150826/
My comment: There is no physical principle, or necessity, that a proton carries two up quarks and one down, and the neutron having one up, and two down. Neither is there a physical principle, that says that rather than the charges of the proton and the electron canceling out, that the net charge could be positive or negative.
Luke A. Barnes The Fine-Tuning of the Universe for Intelligent Life 7 Jun 2012
Lisa Randall: [T]he universe seems to have two entirely different mass scales, and we don’t understand why they are so different. There’s what’s called the Planck scale, which is associated with gravitational interactions. It’s a huge mass scale . . . 1019 GeV. Then there’s the electroweak scale, which sets the masses for the W and Z bosons. [∼ 100 GeV] . . . So the hierarchy problem, in its simplest manifestation, is how can you have these particles be so light when the other scale is so big. (Taubes, 2002)
Frank Wilzcek: [W]e have no . . . compelling idea about the origin of the enormous number [mPl/me] = 2.4 × 1022. If you would like to humble someone who talks glibly about the Theory of Everything, just ask about it, and watch ‘em squirm (Wilczek, 2005).
Leonard Susskind: [T]he up- and down-quarks are absurdly light. The fact that they are roughly twenty thousand times lighter than particles like the Z-boson . . . needs an explanation. The Standard Model has not provided one. Thus, we can ask what the world would be like is the up- and down-quarks were much heavier than they are. Once again — disaster! (Susskind, 2005, pg. 176).
https://arxiv.org/abs/1112.4647
Flatness problem
The relative density Ω against cosmic time t (neither axis to scale). Each curve represents a possible universe: note that Ω diverges rapidly from 1.
https://en.wikipedia.org/wiki/Flatness_problem
LUKE A. BARNES: The Cosmic Revolutionary’s Handbook (Or: How to Beat the Big Bang) 2020
There are a couple of ways we can combine the up quark and the down quark, so could we join three ups together, or three downs?’ Yes, it is possible, and these additional combinations exist. Combining three up quarks gives the attractively named Delta-plus-plus particle (symbolically, Δ++) that has a positive charge of twice that of the proton.
Masses of the fundamental zoo
In fact, it is rather easy to arrange for a universe to have no chemistry at all. Grab a hold of the particle mass dials and let’s create a few universes. For simplicity, we will change the mass of only the up and down quarks, the quarks that are the basic constituents of protons and neutrons. You might think that this would simply make heavier protons and neutrons, and hence slightly heavier things in general. However, the picture is a little more complicated than that. Remember why, despite there being so many types of quarks and so many ways of putting them together, we only see matter built from protons (up-up-down) and neutrons (up-down-down). When we use particle accelerators to make heavier particles, such as the Δ++ (Delta++plus-plus, up-up-up), Σ+ (Sigma-plus, up-up-strange) or even the muon, they decay into lighter particles.
https://3lib.net/book/5500293/8167d2
Michael Brooks There's a glitch at the edge of the universe that could remake physics 6 October 2018
IT IS a well-kept secret, but we know the answer to life, the universe and everything. it’s 1/137.
This immutable number determines how stars burn, how chemistry happens, and even whether atoms exist at all. Physicist Richard Feynman, who knew a thing or two about it, called it “one of the greatest damn mysteries of physics: a magic number that comes to us with no understanding”. Now its mystery is deepening. Controversial hints suggest this number might not be the universal constant we had assumed, instead varying subtly over time and space. If confirmed, that would have profound consequences for our understanding of physics, forcing us to reconsider basic assumptions about the structure of reality.
https://www.newscientist.com/article/mg24031982-200-theres-a-glitch-at-the-edge-of-the-universe-that-could-remake-physics/
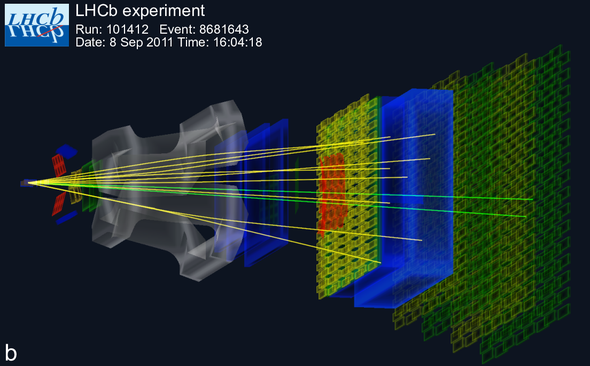
https://reasonandscience.catsboard.com/t3152-could-the-laws-of-physics-change-or-are-they-bound-to-physical-necessity
1. If the laws of physics can change, then the fact that they are set to permit a life-permitting universe demands an explanation.
2. If they cannot change, then they are due to physical necessity, and invoking a lawgiver, who did set them up is not necessary.
3. There are infinite possible ways that the values fundamental constants of the standard models could have been chosen.
4. The laws of physics can change, therefore the fact that they are set up to instantiate a life-permitting universe is best explained by a lawgiver. That lawgiver is God.
Leonard Susskind The Cosmic Landscape: String Theory and the Illusion of Intelligent Design 2006, page 100
By varying the Higgs field, we can add diversity to the world; the laws of nuclear and atomic physics will also vary. A physicist from one region would not entirely recognize the Laws of Physics in another. But the variety inherent in the variations of the Higgs field is very modest. What if the number of variable fields were many hundreds instead of just one? This would imply a multidimensional Landscape, so diverse that almost anything could be found. Then we might begin to wonder what is not possible instead of what is. As we will see this is not idle speculation.
https://3lib.net/book/2472017/1d5be1
The Friedmann equations for the evolution of space predicted the big-bang singularity. General relativity theory predicts a boundary at the big bang, in that the laws of physics break down, and space and time shrink to nothing. That means that the laws of physics had to be instantiated at the Big Bang. As the universe cooled after the Big Bang, symmetries were spontaneously broken, ‘phase transitions’ took place, and discontinuous changes occurred in the values of various physical parameters (e.g., in the strength of certain fundamental interactions, or in the masses of certain species of particle).
[The Lord God] is eternal and infinite, omnipotent and omniscient, that is, he endures from eternity to eternity, and he is present from infinity to infinity; he rules all things, and he knows all things that happen or can happen.
—Isaac Newton, General Scholium to the Principia (1726) Robert Boyle uses the clockwork metaphor to argue for both divine transcendence and the radical contingency of creation. 8
Marc Lange: Could the Laws of Nature Change?* (January 2008)
The natural laws are traditionally characterized as ‘eternal’, ‘fixed’, and ‘immutable’. Is the laws’ unchanging character a metaphysical necessity? If so, then in any possible world, there are exactly the same laws at all times (though presumably there are different laws in different possible worlds).2 That there actually are exactly the same laws at all times is then a consequence of what it is for a truth to be a law of nature. On the other hand, if the laws’ unchanging character is not a metaphysical necessity, then even if in fact there have always been and will always be exactly the same laws, this fact is metaphysically contingent.
As the universe cooled after the Big Bang, symmetries were spontaneously broken, ‘phase transitions’ took place, and discontinuous changes occurred in the values of various physical parameters (e.g., in the strength of certain fundamental interactions, or in the masses of certain species of particle).
One usually assumes that the current laws of physics did not apply [in the period immediately following the Big Bang]. They took hold only after the density of the universe dropped below the so-called Planck density, which equals 1094 grams per cubic centimeter. Lewis’s account entails the laws’ immutability only because a certain parameter in the account has been set to ‘the universe’s entire history’. That parameter could be set differently.
https://philosophy.unc.edu/wp-content/uploads/sites/122/2013/10/Laws-of-nature-change.pdf
My comment: This is a very important admission by the author: The parameter could be set differently. That means, there is no physical restriction or necessity that entails that the parameter could only have the one that is actualized. Since that is so, the question arises: What instantiated the life-permitting parameters? There are two possibilities: Luck, or a Lawgiver. I go with the latter.
KATE BECKER: Are the Laws of Physics Really Universal? OCTOBER 21, 2015
As far as physicists can tell, the cosmos has been playing by the same rulebook since the time of the Big Bang. But could the laws have been different in the past, and could they change in the future?
A scalar field, Carroll explains , is any quantity that has a unique value at every point in space-time. The celebrity-du-jour scalar field is the Higgs, but you can also think of less exotic quantities, like temperature, as scalar fields, too. A yet-undiscovered scalar field that changes very slowly could continue to evolve even billions of years after the Big Bang—and with it, the so-called constants of nature could evolve, too.
https://www.philosophytalk.org/shows/could-laws-physics-ever-change
Clara Moskowitz: 2 Accelerators Find Particles That May Break Known Laws of Physics September 9, 2015
The LHC and the Belle experiment have found particle decay patterns that violate the Standard Model of particle physics, confirming earlier observations at the BaBar facility. Two experiments have observed particles misbehaving in ways not predicted by any known laws of physics. The eyebrow-raising results come from the LHCb experiment at the Large Hadron Collider (LHC) in Switzerland and the Belle experiment at the High Energy Accelerator Research Organization (KEK) in Japan.
Massimo Pigliucci, professor of philosophy at the City University of New York, argues that the word “law” (in the context of a “law of physics” or “law of nature”) is problematic. He maintains that the concept of law raises the question of who or what decided the law to be that way.
In order to have a life-permitting universe, that would entail a “cosmic selection” to fine-tune the universe that we have today. Or an ensemble of multiverses would generate all kinds of universes, amongst them, ours, life-permitting.
https://www.scientificamerican.com/article/2-accelerators-find-particles-that-may-break-known-laws-of-physics1/
Andy Boyd CONSTANTS OF NATURE
What really makes the fine structure constant amazing, as Feynman and others realized, is that if it was somehow even a tiny bit different, the universe we experience wouldn't be the same. In particular, human life would not have evolved. For such a number to come out of thin air is unsettling to say the least. While we understand where some of the constants of nature come from, many seem completely arbitrary, and a better explanation of their origin remains one of the Holy Grails of modern physics.
https://www.uh.edu/engines/epi3149.htm
Philip Ball Can the laws of physics change? 28th March 2012
If Star Trek taught us one thing, it is that “ye cannae change the laws of physics”. That is certainly how scientists generally seem to regard their various laws and constants. No sooner has someone discovered a new “law” than it acquires quasi-legal status, with violations implicitly prohibited. No sooner has someone defined a “constant” than it is assumed to be just that, never varying at different times or places.
And yet, who says so? Why should we assume that a particular mass always exerts the same gravity, or that an atom always has the same mass anyway? After all, one of the most revelatory theories of modern physics, special relativity, showed that the length of a centimetre and the duration of a second can change depending on how fast you are moving. And look at the recent excitement about experiments hinting (and then dismissing due to a faulty connection) that particles called neutrinos could travel faster than the speed of light.
There is no principle of physics that says physical laws or constants have to be the same everywhere and always. This could drive you a bit mad. How can you possibly do science if the rules keep changing? But if you are a scientist, you have to face up to that possibility – and you have a responsibility to check out if it is true.
This brings us to the apparent Lewis Carroll absurdity of a new paper setting out to test if a constant central to quantum physics, called Planck’s constant or h, is really as it says. The fact that it is, as far as we can tell – there does not seem many points in manufacturing suspense here – means that this is not likely to grab any headlines. It sounds a bit like proving that Paris has not moved to China.
Precision timing
But this is an experiment that is significant not so much for what has been found as for the fact that it was done at all. After all, testing the constancy of a constant means going to extraordinary lengths in terms of precision measurements.
To test whether Planck’s constant is really constant, Makan Mohageg and graduate student James Kentosh of California State University in Northridge turned to the same GPS systems that help drivers find their way home. GPS relies on the most accurate timing devices we currently possess: atomic clocks. These count the passage of time according to frequency of the radiation that atoms emit when their electrons jump between different energy levels.
Why go to all this bother? The point is that the researchers did not just pick on a random constant. Planck’s constant is in effect the number that launched the field of quantum physics. In 1900 the German scientist Max Planck proposed h as a measure of the size of energy “packets”, or quanta, into which light is divided. Planck said that a light quantum has an amount of energy equal to the frequency of the light multiplied by h. Planck introduced this “quantum” hypothesis of light as a mathematical trick to get his equations to work out. But Albert Einstein argued five years later that the trick must be taken literally: light really is chopped up into these discrete packets of energy.
Disturbance in the universe?
The reason why Kentosh and Mohageg asked whether h is really constant, however, is not just because it is a central number for modern physics, but because h also appears in the expression for another fundamental constant, called the fine-structure constant. This measures the strength of interactions between light and matter, or equivalently, how strong electrical and magnetic forces are. It can be expressed as a combination of three constants: the charge on an electron, the speed of light, and h.
And here is the crux: some scientists have suggested that the fine structure constant might not be constant, but could vary over time and space. In 1999, a team of astronomers using a telescope in Hawaii reported that measurements of light absorbed by very distant galaxy-like objects in space called quasars – which are so far away that we see them today as they looked billions of years ago – suggest that the value of the fine-structure constant was once slightly different from what it is today.
That claim was controversial, and still unproven. But if true, it must mean that at least one of the three fundamental constants that constitute it must vary.
Location, location, location
Kentosh and Mohageg fixed on h, and specifically on whether h depends on where (not when) you measure it. If h changes from place to place, so do the frequencies, and thus the “ticking rate”, of atomic clocks. And any dependence of h on location would translate as a tiny timing discrepancy between different GPS clocks.
So, what did they discover? Well, if there is any difference in h it would have to be really tiny. After careful analysis of the data from seven highly stable GPS satellites, Kentosh and Mohageg conclude that h is identical at different locations to an accuracy of seven parts in a thousand. In other words, if h were a one-metre measuring stick, two sticks in different places anywhere in the world do not differ by more than seven millimetres.
Spotting this variation of less than 1% in measuring sticks might be easy, spotting this in an exceedingly tiny number like Planck’s constant, which is 0.000000000000000000000000000000000662606957 joule seconds, demands the type of extreme accuracy of measurement that is most likely beyond the capabilities of our most accurate atomic clocks. At this point, however, we can feel reassured that there is no reason to suspect that this particular aspect of physics shifts between, say, London and Beijing – or indeed, between our galaxy and the next one.
https://www.bbc.com/future/article/20120329-can-the-laws-of-physics-change
New findings suggest laws of nature not as constant as previously thought April 27, 2020
Four new measurements of light emitted from a quasar 13 billion light-years away reaffirm past studies that have measured tiny variations in the fine structure constant.
https://www.sciencedaily.com/releases/2020/04/200427102544.htm
What is the difference between classical physics and quantum physics?
The electron, the proton, and the quark are all entities within the realm of particle hence quantum physics. All three carry an electrical charge. The electric charge of the proton is exactly equal and the opposite of the electric charge on the electron, despite the proton being 1836 times more massive. There’s no set-in-concrete theoretical reason why this should be so. It cannot be determined from first principles, only experimentally measured.
An electron has a negative charge exactly equal to and opposite to that of a proton. Note: the charge is exactly equal, even though the proton has a far greater mass than the electron (some 2000 times heavier in fact, not that there has to be of necessity any relationship between mass and charge).
Now that’s strange since the electron is a fundamental particle but the positively charged proton is a composite particle, made up of a trio of quarks (as is the neutron with no net charge). The proton has two quarks each with a positive 2/3rds charge (up quark) and one quark with a negative 1/3rd charge (down quark) for an overall balance of one positive charge. (The neutron, on the other hand, has one up quark with a positive 2/3rds charge and two down quarks each with a negative 1/3rd charge, for an overall balance of zero charge – neither positive nor negative.)
Now you might suggest that an electron might be a fusion of a trio of down quarks, each with a negative 1/3rd charge, except the electron, again, isn’t a composite particle, and the mass is all wrong for that scenario. If an electron were a composite of a trio of down quarks, each with a minus 1/3rd charge, the electron would be thirty times more massive than it is – not something particle physicists would fail to take notice of.
Further, the force particle that governs the electron is the photon; that which governs the quarks inside the proton and the neutron is the gluon, which further differentiates the two things – quarks and electrons. In any event, if you could have a composite particle of a trio of negative 1/3rd down quarks, if that were the case, and it is the case, and it’s called the Negative Delta, you’d also need a composite particle that’s the fusion of a trio of positive 2/3rds up quarks for an overall charge of plus two. To the best of my knowledge, there is only one such critter in the particle zoo and it’s called the Doubly Positive Delta. I’m sure you’ve never heard of these Delta particles, which goes to show how much bearing or impact they have on life, the Universe, and everything.
http://www.esalq.usp.br/lepse/imgs/conteudo_thumb/What-is-the-difference-between-classical-physics-and-quantum-physics.pdf
Frank Wilczek: Asymptotic freedom: From paradox to paradigm 2005 Jun 8
In the early 1960s, Murray Gell-Mann and George Zweig made a great advance in the theory of the strong interaction by proposing the concept of quarks. If you imagined that hadrons were not fundamental particles, but rather that they were assembled from a few more basic types, the quarks, patterns clicked into place. The dozens of observed hadrons could be understood, at least roughly, as different ways of putting together just three kinds (“flavors”) of quarks. You can have a given set of quarks in different spatial orbits, or with their spins aligned in different ways. The energy of the configuration will depend on these things, and so there will be a number of states with different energies, giving rise to particles with different masses, according to m = E/c2.
My comment: There is no physical constraint that dictates the composition of a proton, or neutron. But it is precisely the composition that is, which permits the existence of atoms, and a life-permitting universe.
The electron, the proton, and the quark are all entities within the realm of particle hence quantum physics. All three carry an electrical charge. All three have mass. The electric charge of the proton is exactly equal and the opposite of the electric charge on the electron, despite the proton being 1836 times more massive. There’s no set-in-concrete theoretical reason why this should be so. It cannot be determined from first principles, only experimentally measured. The proton is a composite particle, made up of a trio of quarks (as is the neutron with no net charge). The proton has two quarks each with a positive 2/3rds charge (up quark) and one quark with a negative 1/3rd charge (down quark) for an overall balance of one positive charge. (The neutron, on the other hand, has one up quark with a positive 2/3rds charge and two down quarks each with a negative 1/3rd charge, for an overall balance of zero charge – neither positive nor negative.)
https://www.ncbi.nlm.nih.gov/pmc/articles/PMC1150826/
My comment: There is no physical principle, or necessity, that a proton carries two up quarks and one down, and the neutron having one up, and two down. Neither is there a physical principle, that says that rather than the charges of the proton and the electron canceling out, that the net charge could be positive or negative.
Luke A. Barnes The Fine-Tuning of the Universe for Intelligent Life 7 Jun 2012
Lisa Randall: [T]he universe seems to have two entirely different mass scales, and we don’t understand why they are so different. There’s what’s called the Planck scale, which is associated with gravitational interactions. It’s a huge mass scale . . . 1019 GeV. Then there’s the electroweak scale, which sets the masses for the W and Z bosons. [∼ 100 GeV] . . . So the hierarchy problem, in its simplest manifestation, is how can you have these particles be so light when the other scale is so big. (Taubes, 2002)
Frank Wilzcek: [W]e have no . . . compelling idea about the origin of the enormous number [mPl/me] = 2.4 × 1022. If you would like to humble someone who talks glibly about the Theory of Everything, just ask about it, and watch ‘em squirm (Wilczek, 2005).
Leonard Susskind: [T]he up- and down-quarks are absurdly light. The fact that they are roughly twenty thousand times lighter than particles like the Z-boson . . . needs an explanation. The Standard Model has not provided one. Thus, we can ask what the world would be like is the up- and down-quarks were much heavier than they are. Once again — disaster! (Susskind, 2005, pg. 176).
https://arxiv.org/abs/1112.4647
Flatness problem
The relative density Ω against cosmic time t (neither axis to scale). Each curve represents a possible universe: note that Ω diverges rapidly from 1.
https://en.wikipedia.org/wiki/Flatness_problem
LUKE A. BARNES: The Cosmic Revolutionary’s Handbook (Or: How to Beat the Big Bang) 2020
There are a couple of ways we can combine the up quark and the down quark, so could we join three ups together, or three downs?’ Yes, it is possible, and these additional combinations exist. Combining three up quarks gives the attractively named Delta-plus-plus particle (symbolically, Δ++) that has a positive charge of twice that of the proton.
Masses of the fundamental zoo
In fact, it is rather easy to arrange for a universe to have no chemistry at all. Grab a hold of the particle mass dials and let’s create a few universes. For simplicity, we will change the mass of only the up and down quarks, the quarks that are the basic constituents of protons and neutrons. You might think that this would simply make heavier protons and neutrons, and hence slightly heavier things in general. However, the picture is a little more complicated than that. Remember why, despite there being so many types of quarks and so many ways of putting them together, we only see matter built from protons (up-up-down) and neutrons (up-down-down). When we use particle accelerators to make heavier particles, such as the Δ++ (Delta++plus-plus, up-up-up), Σ+ (Sigma-plus, up-up-strange) or even the muon, they decay into lighter particles.
https://3lib.net/book/5500293/8167d2
Michael Brooks There's a glitch at the edge of the universe that could remake physics 6 October 2018
IT IS a well-kept secret, but we know the answer to life, the universe and everything. it’s 1/137.
This immutable number determines how stars burn, how chemistry happens, and even whether atoms exist at all. Physicist Richard Feynman, who knew a thing or two about it, called it “one of the greatest damn mysteries of physics: a magic number that comes to us with no understanding”. Now its mystery is deepening. Controversial hints suggest this number might not be the universal constant we had assumed, instead varying subtly over time and space. If confirmed, that would have profound consequences for our understanding of physics, forcing us to reconsider basic assumptions about the structure of reality.
https://www.newscientist.com/article/mg24031982-200-theres-a-glitch-at-the-edge-of-the-universe-that-could-remake-physics/
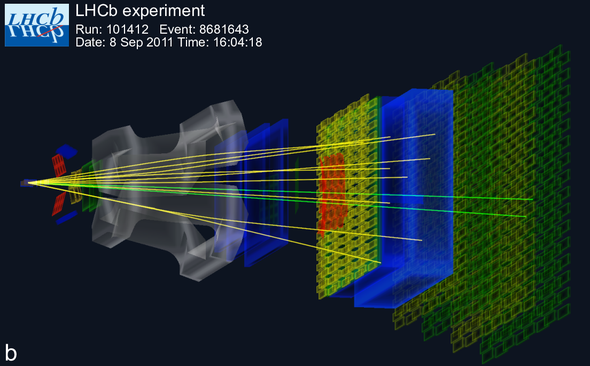
Last edited by Otangelo on Mon Jul 26, 2021 1:42 pm; edited 5 times in total