ABC transporters
ABC Transporters
Bacterial ABC transporters are essential in cell viability, virulence, and pathogenicity. The substrates that can be transported include ions, amino acids, peptides, sugars, and other molecules that are mostly hydrophilic. The ATP-binding cassette (ABC) family is a group of proteins which bind and hydrolyse ATP in order to transport substances across cellular membranes. ATP-binding cassette transporters (ABC-transporters) are members of a protein superfamily that is one of the largest and most ancient families with representatives in all extant phyla from prokaryotes to humans. ABC transporters are transmembrane proteins that utilize the energy of adenosine triphosphate (ATP) hydrolysis to carry out certain biological processes including translocation of various substrates across membranes and non-transport-related processes such as translation of RNA and DNA repair. They transport a wide variety of substrates across extra- and intracellular membranes, including metabolic products, lipids and sterols, and drugs. Proteins are classified as ABC transporters based on the sequence and organization of their ATP-binding cassette (ABC) domain(s).
ABC Transporters Constitute the Largest Family of Membrane Transport Proteins
The last type of transport ATPase that we discuss is the family of the ABC transporters, so named because each member contains two highly conserved ATPase domains, or ATP-Binding “Cassettes,” on the cytosolic side of the membrane. ATP binding brings together the two ATPase domains, and ATP hydrolysis leads to their dissociation (Figure 11–16).
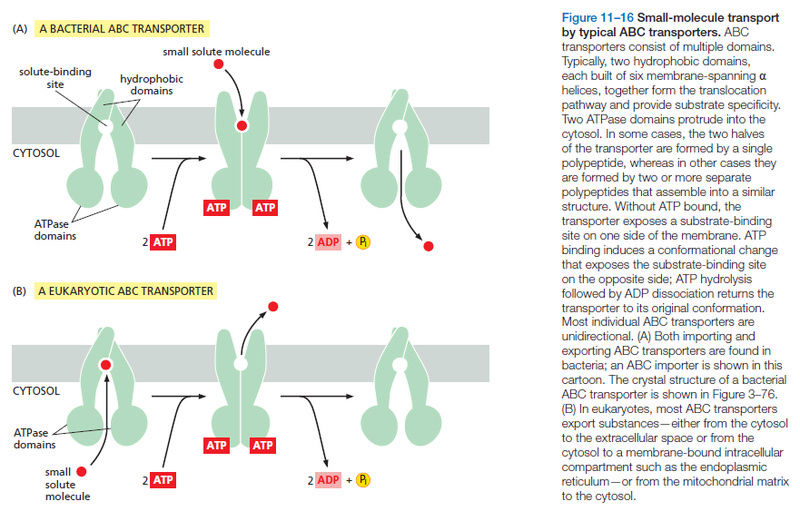
These movements of the cytosolic domains are transmitted to the transmembrane segments, driving cycles of conformational changes that alternately expose solute-binding sites on one side of the membrane and then on the other, as we have seen for other transporters. In this way, ABC transporters harvest the energy released upon ATP binding and hydrolysis to drive transport of solutes across the bilayer. The transport is directional toward
inside or toward outside, depending on the particular conformational change in the solute binding site that is linked to ATP hydrolysis (see Figure 11–16). ABC transporters constitute the largest family of membrane transport proteins and are of great clinical importance. The first of these proteins to be characterized was found in bacteria. We have already mentioned that the plasma membranes of all bacteria contain transporters that use the H+ gradient across the membrane to actively transport a variety of nutrients into the cell. In addition, bacteria use ABC transporters to import certain small molecules. In bacteria such as E. coli that have double membranes (Figure 11–17),
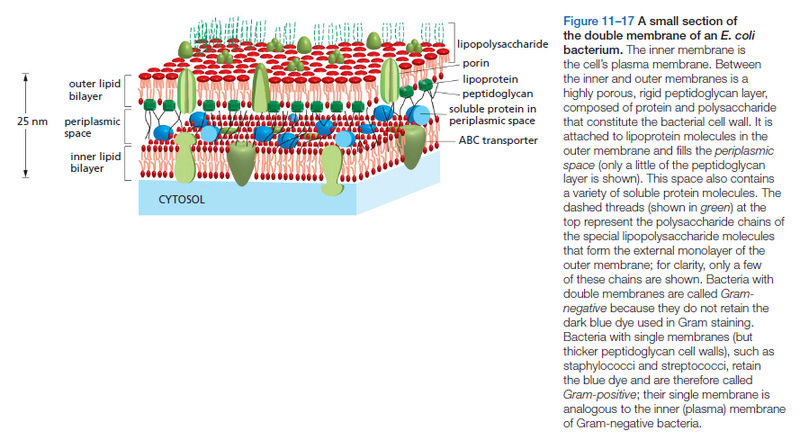
the ABC transporters are located in the inner membrane, and an auxiliary mechanism operates to capture the nutrients and deliver them to the transporters (Figure 11–18).

In E. coli, 78 genes (an amazing 5% of the bacterium’s genes) encode ABC transporters, and animal genomes encode an even larger number. Although each transporter is thought to be specific for a particular molecule or class of molecules, the variety of substrates transported by this superfamily is great and includes inorganic ions, amino acids, mono- and polysaccharides, peptides, lipids, drugs, and, in some cases, even proteins that can be larger than the transporter itself. The first eukaryotic ABC transporters identified were discovered because of their ability to pump hydrophobic drugs out of the cytosol. One of these transporters is the multidrug resistance (MDR) protein, also called P-glycoprotein. It is present at elevated levels in many human cancer cells and makes the cells simultaneously resistant to a variety of chemically unrelated cytotoxic drugs that arewidely used in cancer chemotherapy. Treatment with any one of these drugs can result in the selective survival and overgrowth of those cancer cells that express an especially large amount of the MDR transporter. These cells pump drugs out of the cell very efficiently and are therefore relatively resistant to the drugs’ toxic effects. Selection for cancer cells with resistance to one drug can thereby lead to resistance to a wide variety of anticancer drugs. Some studies indicate that up to 40% of human cancers develop multidrug resistance, making it a major hurdle in the battle against cancer.
A related and equally sinister phenomenon occurs in the protist Plasmodium falciparum, which causes malaria. More than 200 million people are infected worldwide with this parasite, which remains a major cause of human death, killing almost a million people every year. The development of resistance to the antimalarial drug chloroquine has hampered the control of malaria. The resistant P. falciparum have amplified a gene encoding an ABC transporter that pumps out the chloroquine.

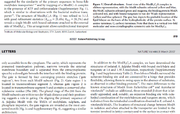



ABC Transporters
Bacterial ABC transporters are essential in cell viability, virulence, and pathogenicity. The substrates that can be transported include ions, amino acids, peptides, sugars, and other molecules that are mostly hydrophilic. The ATP-binding cassette (ABC) family is a group of proteins which bind and hydrolyse ATP in order to transport substances across cellular membranes. ATP-binding cassette transporters (ABC-transporters) are members of a protein superfamily that is one of the largest and most ancient families with representatives in all extant phyla from prokaryotes to humans. ABC transporters are transmembrane proteins that utilize the energy of adenosine triphosphate (ATP) hydrolysis to carry out certain biological processes including translocation of various substrates across membranes and non-transport-related processes such as translation of RNA and DNA repair. They transport a wide variety of substrates across extra- and intracellular membranes, including metabolic products, lipids and sterols, and drugs. Proteins are classified as ABC transporters based on the sequence and organization of their ATP-binding cassette (ABC) domain(s).
ABC Transporters Constitute the Largest Family of Membrane Transport Proteins
The last type of transport ATPase that we discuss is the family of the ABC transporters, so named because each member contains two highly conserved ATPase domains, or ATP-Binding “Cassettes,” on the cytosolic side of the membrane. ATP binding brings together the two ATPase domains, and ATP hydrolysis leads to their dissociation (Figure 11–16).
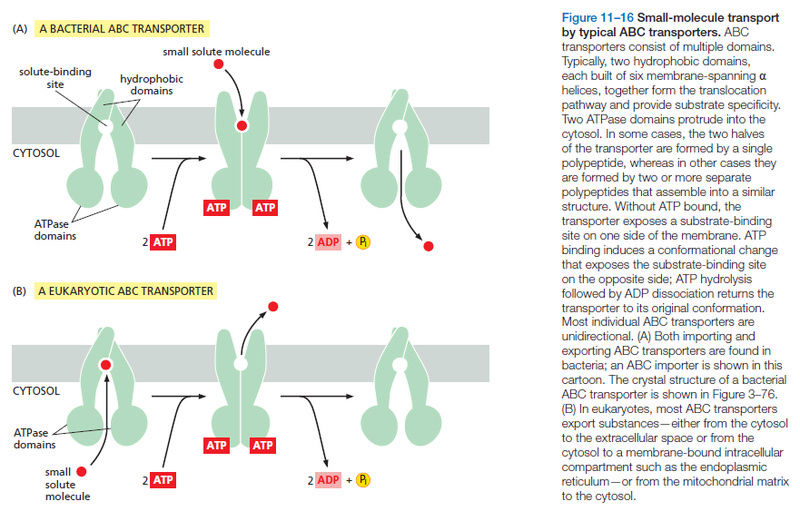
These movements of the cytosolic domains are transmitted to the transmembrane segments, driving cycles of conformational changes that alternately expose solute-binding sites on one side of the membrane and then on the other, as we have seen for other transporters. In this way, ABC transporters harvest the energy released upon ATP binding and hydrolysis to drive transport of solutes across the bilayer. The transport is directional toward
inside or toward outside, depending on the particular conformational change in the solute binding site that is linked to ATP hydrolysis (see Figure 11–16). ABC transporters constitute the largest family of membrane transport proteins and are of great clinical importance. The first of these proteins to be characterized was found in bacteria. We have already mentioned that the plasma membranes of all bacteria contain transporters that use the H+ gradient across the membrane to actively transport a variety of nutrients into the cell. In addition, bacteria use ABC transporters to import certain small molecules. In bacteria such as E. coli that have double membranes (Figure 11–17),
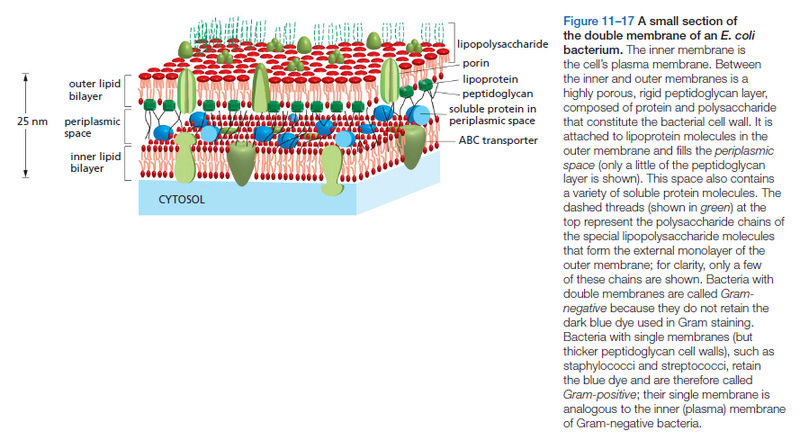
the ABC transporters are located in the inner membrane, and an auxiliary mechanism operates to capture the nutrients and deliver them to the transporters (Figure 11–18).

In E. coli, 78 genes (an amazing 5% of the bacterium’s genes) encode ABC transporters, and animal genomes encode an even larger number. Although each transporter is thought to be specific for a particular molecule or class of molecules, the variety of substrates transported by this superfamily is great and includes inorganic ions, amino acids, mono- and polysaccharides, peptides, lipids, drugs, and, in some cases, even proteins that can be larger than the transporter itself. The first eukaryotic ABC transporters identified were discovered because of their ability to pump hydrophobic drugs out of the cytosol. One of these transporters is the multidrug resistance (MDR) protein, also called P-glycoprotein. It is present at elevated levels in many human cancer cells and makes the cells simultaneously resistant to a variety of chemically unrelated cytotoxic drugs that arewidely used in cancer chemotherapy. Treatment with any one of these drugs can result in the selective survival and overgrowth of those cancer cells that express an especially large amount of the MDR transporter. These cells pump drugs out of the cell very efficiently and are therefore relatively resistant to the drugs’ toxic effects. Selection for cancer cells with resistance to one drug can thereby lead to resistance to a wide variety of anticancer drugs. Some studies indicate that up to 40% of human cancers develop multidrug resistance, making it a major hurdle in the battle against cancer.
A related and equally sinister phenomenon occurs in the protist Plasmodium falciparum, which causes malaria. More than 200 million people are infected worldwide with this parasite, which remains a major cause of human death, killing almost a million people every year. The development of resistance to the antimalarial drug chloroquine has hampered the control of malaria. The resistant P. falciparum have amplified a gene encoding an ABC transporter that pumps out the chloroquine.

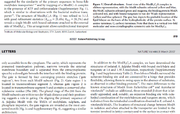



Last edited by Admin on Sat Feb 18, 2017 11:48 am; edited 2 times in total