Angiogenesis and Vasculogenesis
Angiogenesis and vasculogenesis are essential processes involved in the formation and maintenance of blood vessels within biological systems. Angiogenesis refers to the formation of new blood vessels from preexisting ones. It plays a crucial role in various biological contexts, such as wound healing, tissue regeneration, and development. Angiogenesis produces a network of blood vessels that supply nutrients and oxygen to tissues, remove waste products, and facilitate the exchange of molecules between the bloodstream and surrounding cells. This process is particularly important in embryonic development, tissue repair, and growth, as well as in conditions like cancer where new blood vessels support tumor growth and metastasis. Vasculogenesis is the process by which new blood vessels are formed de novo from endothelial progenitor cells. It is particularly significant during embryonic development when the cardiovascular system is initially established. Vasculogenesis contributes to the formation of the primary vascular plexus, which serves as a scaffold for further vascular remodeling and the eventual development of a mature vascular network. Defects in vasculogenesis can lead to severe developmental abnormalities. Angiogenesis and vasculogenesis are fundamental for the survival and function of complex multicellular organisms. They enable efficient transport of nutrients, oxygen, hormones, and immune cells throughout the body. These processes are vital for tissue growth, repair, and regeneration, as well as for maintaining proper physiological functions. Additionally, angiogenesis plays a role in various diseases, such as cancer, where excessive or aberrant blood vessel formation supports tumor growth. Understanding the molecular mechanisms underlying angiogenesis and vasculogenesis has implications for developing therapeutic strategies for conditions involving abnormal blood vessel formation or inadequate blood supply to tissues.
How do angiogenesis and vasculogenesis contribute to the establishment of blood vessel networks during embryonic development?
Angiogenesis and vasculogenesis are essential processes that contribute to the establishment of blood vessel networks during embryonic development. These processes involve the formation, expansion, and remodeling of blood vessels, which are crucial for supplying nutrients, oxygen, and other essential molecules to developing tissues and organs. Here's how angiogenesis and vasculogenesis work together to create functional vascular systems:
Vasculogenesis
Formation of Blood Islands: Vasculogenesis begins with the formation of blood islands, which are clusters of angioblasts (precursor cells) that differentiate into endothelial cells, the building blocks of blood vessels.
Angioblast Migration and Aggregation: Angioblasts migrate to specific areas in the developing embryo, guided by chemical signals and gradients. They aggregate to form endothelial cords or tubes, which serve as the initial structures of blood vessels.
Vascular Lumen Formation: The endothelial cords undergo lumenization, during which they develop a central channel, or lumen. This lumen becomes the pathway for blood flow.
Hemangioblast Differentiation: Some angioblasts differentiate into hemangioblasts, which give rise to both endothelial cells and blood cells. This connection between blood vessel formation and blood cell production is crucial for the functional development of the circulatory system.
Angiogenesis
Sprouting Angiogenesis: In regions where tissues require increased blood supply, endothelial cells from existing vessels start to sprout out in response to pro-angiogenic signals, such as growth factors like VEGF (vascular endothelial growth factor).
Migration and Proliferation: Sprouting endothelial cells migrate towards the source of pro-angiogenic signals and proliferate to form new endothelial sprouts.
Lumen Formation: Similar to vasculogenesis, the endothelial sprouts undergo lumenization to form functional tubular structures.
Anastomosis: The newly formed sprouts elongate and connect with adjacent sprouts, leading to the establishment of interconnected networks of blood vessels. This anastomosis process creates a functional circulation system.
Stabilization: Pericytes and smooth muscle cells are recruited to the developing vessels to provide structural support and stability to the new blood vessels.
Appearance of angiogenesis and vasculogenesis in the evolutionary timeline
The appearance of angiogenesis and vasculogenesis in the evolutionary timeline is a complex topic that involves speculation and ongoing research. While the exact timing is not definitively known, scientists have proposed hypotheses about when these processes might have emerged based on comparative studies and the fossil record. Angiogenesis and vasculogenesis are fundamental processes for the development and maintenance of blood vessels in organisms. Angiogenesis involves the formation of new blood vessels from preexisting ones, while vasculogenesis refers to the de novo formation of blood vessels from endothelial precursor cells.
Early Life Forms (Prokaryotes): These simple organisms did not possess complex vascular systems or mechanisms like angiogenesis and vasculogenesis. Their nutrient exchange and waste removal were likely facilitated by direct diffusion through cell membranes due to their small size and relatively simple structure.
Simple Eukaryotes: As organisms supposedly evolved into more complex eukaryotic forms, some multicellular organisms would have started to develop basic mechanisms to transport nutrients and waste products. However, true vascular systems had not yet emerged, and mechanisms like diffusion and simple tissue organization served these early organisms.
Invertebrates: The appearance of more complex invertebrates would have marked the transition toward more developed circulatory systems. Many invertebrates have open circulatory systems where blood is pumped into body cavities, allowing for nutrient exchange. These systems would have involved rudimentary precursor processes that could be considered primitive forms of angiogenesis.
Vertebrates: The emergence of vertebrates would have brought about more sophisticated circulatory systems, including closed systems with dedicated blood vessels. While the exact point at which angiogenesis and vasculogenesis evolved in vertebrate history is unclear, they would have played essential roles in the development of more advanced vascular systems.
Early Vertebrates: Over time, vertebrates would have evolved into increasingly complex cardiovascular systems. The emergence of angiogenesis and vasculogenesis would have accompanied the need for more efficient nutrient delivery, waste removal, and tissue repair. These processes would have allowed vertebrates to sustain larger and more metabolically active bodies.
De Novo Genetic Information, necessary to instantiate angiogenesis and vasculogenesis
The hypothetical emergence of angiogenesis, the process of forming new blood vessels from scratch in the development of organisms that didn't previously possess a vascular system, would have required the addition of specific genetic information to enable this complex process. While the exact genetic changes are speculative, here are some potential additions or modifications to genetic information that could have been involved in the evolution of angiogenesis:
Angiogenic Factors: The development of angiogenesis from scratch would have necessitated the evolution of genes encoding angiogenic factors, such as rudimentary versions of vascular endothelial growth factors (VEGFs). These factors would act as signaling molecules to initiate vessel formation.
Receptor Proteins: The emergence of angiogenesis would have required the development of new receptor proteins on hypothetical endothelial-like precursor cells. These receptors would allow cells to detect and respond to angiogenic factors, initiating the signaling cascades necessary for vessel formation.
Cell Adhesion Molecules: As cells begin to organize into blood vessels, new genetic information could have been necessary to generate primitive cell adhesion molecules. These molecules would facilitate cell-cell interactions and the formation of vessel-like structures.
Matrix Remodeling Enzymes: The initial formation of blood vessels from scratch would involve breaking down and restructuring extracellular matrix. Genes encoding matrix remodeling enzymes could have evolved to allow cells to create pathways for vessel growth.
Transcription Factors: The evolution of angiogenesis would require new transcription factors that activate gene expression programs specific to vessel formation. These factors would regulate the expression of genes involved in cell migration, proliferation, and differentiation.
Guidance Proteins: To direct the movement of primitive endothelial-like cells toward the formation of blood vessels, hypothetical guidance proteins could have evolved to provide directional cues.
Signaling Molecules and Pathways: New genetic information would be needed to establish the initial signaling pathways that trigger cellular responses, such as migration, proliferation, and differentiation, during angiogenesis.
Cytoskeletal Regulators: The development of angiogenesis from scratch would require genes that control cytoskeletal dynamics, enabling cell movement, migration, and organization into vessel-like structures.
Apoptosis and Survival Regulators: As cells form blood vessels, mechanisms for balancing cell survival and cell death would be crucial. Genes involved in apoptosis and survival pathways might have been required.
Differentiation and Specification Genes: The evolution of angiogenesis would involve the development of genes that specify and differentiate precursor cells into endothelial-like cells with distinct roles in vessel formation.
Cell-Cell Communication Molecules: New genetic information could have been necessary to allow cells to communicate and coordinate their behaviors during the formation of blood vessels.
Cell Signaling Pathways for Coordination: To ensure proper coordination among endothelial-like cells, genetic information might have evolved to create rudimentary cell signaling pathways for communication and synchronization.
Epigenetic Regulatory Mechanisms necessary to be instantiated
The hypothetical emergence of angiogenesis "from scratch," meaning the evolution of blood vessel formation in organisms that previously lacked such a system, would have required the establishment of new epigenetic regulations to coordinate the complex cellular changes necessary for vessel development.
Epigenetic Priming for Vascular Precursors: The initial step might have involved the establishment of epigenetic marks that prime certain precursor cells for vascular differentiation. These marks could create a permissive chromatin environment for angiogenesis-related genes.
Histone Modifications for Vessel Formation: The evolution of angiogenesis would have necessitated the emergence of specific histone modifications that activate genes involved in vessel development. Histone acetylation, for example, could enhance the expression of genes related to endothelial differentiation and tube formation.
DNA Methylation Dynamics: The formation of blood vessels would require the establishment of specific DNA methylation patterns. New patterns could regulate the expression of genes essential for angiogenesis, fine-tuning their activation during different stages of vessel formation.
Non-Coding RNA-Mediated Regulation: The evolution of angiogenesis might have led to the emergence of non-coding RNAs, such as microRNAs or long non-coding RNAs, which could interact with chromatin-modifying complexes to regulate the expression of genes associated with vessel development.
Imprinting and Allelic Regulation: To ensure proper vessel assembly, the evolution of angiogenesis could involve the establishment of allele-specific epigenetic marks that guide cells to adopt specific roles during vessel formation.
Epigenetic Inheritance of Vascular Patterns: The ability to pass down epigenetic information related to vessel formation could have evolved, allowing subsequent generations to inherit the regulatory marks necessary for angiogenesis.
Temporal Epigenetic Regulations: The coordinated development of angiogenesis would require the evolution of epigenetic mechanisms that act as molecular "clocks," ensuring the precise timing of vessel-related processes.
Suppression of Anti-Angiogenic Factors: New epigenetic regulations could have evolved to suppress the expression of genes encoding anti-angiogenic factors, ensuring that the vessel development process is not hindered.
Chromatin Remodeling for Vessel Assembly: As endothelial-like cells come together to form vessels, new epigenetic mechanisms might have been required to regulate chromatin remodeling, facilitating proper cell-cell interactions and tube formation.
Epigenetic Regulation of Vascular Maturation: Epigenetic marks might have been necessary to guide the maturation and stabilization of newly formed vessels, including the recruitment of support cells like pericytes and smooth muscle cells.
Epigenetic Sensing of Environmental Cues: The evolution of angiogenesis would likely require epigenetic mechanisms that enable cells to sense and respond to environmental signals, allowing for adaptive vessel formation in different contexts.
Signaling Pathways necessary to create, and maintain angiogenesis and vasculogenesis
If we're considering the emergence of angiogenesis "from scratch," i.e., the initial development of the process in organisms that didn't previously have any vascular system, potential signaling pathways that would have had to be involved in the hypothetical evolution of angiogenesis: are:
Basic Growth Factor and Receptor Pathways: The emergence of angiogenesis would have required the evolution of basic growth factor pathways and receptor systems that stimulate cell proliferation, migration, and differentiation. Simple signaling cascades involving ligands and receptors might have formed the foundation for more complex angiogenic signaling.
Chemotaxis Pathways: Hypothetically, rudimentary chemotactic signaling pathways could have evolved to guide primitive endothelial-like cells toward specific gradients of factors that promote cell movement. These could have been primitive versions of later-developing pathways.
Adhesion Pathways: Early angiogenesis would have necessitated cell adhesion and migration. Signaling pathways related to cell adhesion molecules could have emerged to facilitate endothelial cell movement and organization into nascent blood vessels.
Cytoskeletal Remodeling Pathways: The evolution of angiogenesis would likely have involved mechanisms for altering cell shape and movement. Signaling pathways related to the cytoskeleton, such as actin and microtubule dynamics, could have been essential.
Cell-Cell Communication Pathways: Basic cell-cell communication pathways might have developed to allow primitive endothelial cells to coordinate their behaviors during the initial stages of vessel formation.
Apoptosis and Survival Pathways: As vessel formation requires the precise balance between cell death (apoptosis) and cell survival, hypothetical pathways regulating these processes might have been crucial.
Initial Extracellular Matrix Signaling: Signaling pathways involved in responding to the extracellular environment could have emerged to guide cells to form structures resembling the rudimentary precursor of blood vessels.
Evolution of Ligand-Receptor Pairs: New ligand-receptor pairs might have evolved, allowing cells to sense and respond to cues that promote vessel formation.
The emergence of angiogenesis, the process of forming new blood vessels from preexisting ones, would have involved the establishment and modification of specific signaling pathways to coordinate the complex cellular changes required for vessel formation. While the exact signaling pathways are speculative, here are some potential pathways that could have been involved in the emergence of angiogenesis:
VEGF Signaling Pathway: Vascular endothelial growth factor (VEGF) is a central regulator of angiogenesis. The VEGF signaling pathway involves the binding of VEGF to its receptors (VEGFRs) on endothelial cells. This activates downstream signaling cascades that promote endothelial cell proliferation, migration, and tube formation.
FGF Signaling Pathway: Fibroblast growth factors (FGFs) also play a role in angiogenesis. The FGF signaling pathway, similar to the VEGF pathway, involves FGF ligands binding to their receptors, which triggers signaling events that contribute to endothelial cell proliferation and migration.
Notch Signaling Pathway: The Notch pathway is involved in cell-cell communication and could have played a role in coordinating endothelial cell differentiation and tip cell selection during vessel sprouting.
Wnt Signaling Pathway: The Wnt pathway has diverse roles in development, and its components could have been involved in angiogenesis by influencing endothelial cell behavior and vessel branching patterns.
TGF-β Signaling Pathway: Transforming growth factor-beta (TGF-β) family members could have been implicated in angiogenesis by regulating endothelial cell differentiation and extracellular matrix remodeling.
PDGF Signaling Pathway: Platelet-derived growth factor (PDGF) signaling might have been involved in recruiting pericytes and smooth muscle cells to stabilize and mature newly formed vessels.
ECM Signaling Pathways: Extracellular matrix (ECM) components, including integrins and focal adhesion kinase (FAK), could have participated in transmitting signals that guide endothelial cell migration and vessel assembly.
MAPK Signaling Pathway: Mitogen-activated protein kinase (MAPK) pathways could have been essential for transmitting signals that regulate cell proliferation, survival, and migration during angiogenesis.
PI3K/AKT Signaling Pathway: The phosphatidylinositol 3-kinase (PI3K)/AKT pathway could have been involved in promoting endothelial cell survival, migration, and angiogenesis-related cellular responses.
Rho GTPase Signaling Pathway: Rho GTPases, such as Rho, Rac, and Cdc42, could have participated in regulating cytoskeletal dynamics and cell migration during angiogenesis.
Chemokine Signaling: Chemokines and their receptors might have guided endothelial cell migration and positioning during vessel sprouting.
Hedgehog Signaling Pathway: Hedgehog signaling could have been implicated in regulating vascular patterning and endothelial cell differentiation.
Endothelial-Specific Signaling Pathways: Signaling pathways specifically active in endothelial cells could have evolved to control angiogenesis-related processes like proliferation, migration, and tube formation.
Regulatory codes necessary for maintenance and operation
The hypothetical emergence of blood vessels and angiogenesis would have likely involved the establishment of regulatory codes and languages to coordinate the development, maintenance, and operation of the vascular system. While the exact details are speculative, here are potential regulatory codes and languages that could have been instantiated:
Transcriptional Regulatory Code: The evolution of blood vessels would require a transcriptional regulatory code involving specific DNA sequences, transcription factors, and regulatory elements that control the expression of genes involved in vessel development, maintenance, and function.
Cis-Regulatory Elements: Enhancers, promoters, and other cis-regulatory elements would need to evolve to ensure proper spatiotemporal expression of angiogenesis-related genes.
Epigenetic Regulatory Language: Epigenetic modifications such as DNA methylation and histone modifications could form an epigenetic regulatory language that guides the activation and repression of genes essential for vascular development and maintenance.
Signaling Pathway Crosstalk: Complex signaling pathways involved in angiogenesis and vascular function would need to communicate and coordinate their activities through a regulatory language that ensures proper cellular responses.
Cell-Cell Communication Codes: As blood vessels involve multiple cell types, a communication code involving cell surface receptors, ligands, and their interactions would be necessary to coordinate cellular behaviors and functions.
Extracellular Matrix (ECM) Signaling: A code involving interactions between cells and the extracellular matrix would regulate processes such as cell adhesion, migration, and signaling.
Vascular Patterning Code: The establishment of hierarchical vessel networks would require a code that guides the formation and branching patterns of blood vessels to ensure efficient distribution of nutrients and oxygen.
Stability and Maturation Code: Blood vessel stabilization and maturation would require a regulatory code involving communication between endothelial cells and support cells (pericytes and smooth muscle cells) to ensure structural integrity.
Oxygen and Nutrient Sensing Code: Blood vessels need to adapt to changing oxygen and nutrient levels. A regulatory code might govern the response of vessels to these fluctuations, ensuring appropriate vessel dilation and constriction.
Immune-Endothelial Communication: Blood vessels interact with the immune system. A code would be needed to regulate the communication between endothelial cells and immune cells, enabling immune surveillance and inflammation regulation.
Inflammatory Response Code: Inflammatory responses and repair processes would require a regulatory code to activate and control specific genes involved in tissue repair and vessel remodeling.
Vascular Tone and Homeostasis Code: Blood pressure and vessel tone need to be tightly regulated. A code would be necessary to balance vasoconstriction and vasodilation to maintain blood flow and homeostasis.
Angiogenic Switch Code: The transition from quiescent vessels to angiogenesis activation would require a code that senses environmental cues and triggers angiogenic responses.
Vascular Regression Code: Vessels need to regress when not needed. A regulatory code would be necessary to initiate vessel regression and tissue remodeling.
Wound Healing and Regeneration Code: Blood vessels play a role in tissue repair. A code would be involved in coordinating vessel-related processes during wound healing and tissue regeneration.
These regulatory codes and languages would have had to emerge to ensure the development, maintenance, and operation of blood vessels. The precise details would depend on the specific context and the genetic and molecular mechanisms that contributed to the emergence of angiogenesis and vascular systems.
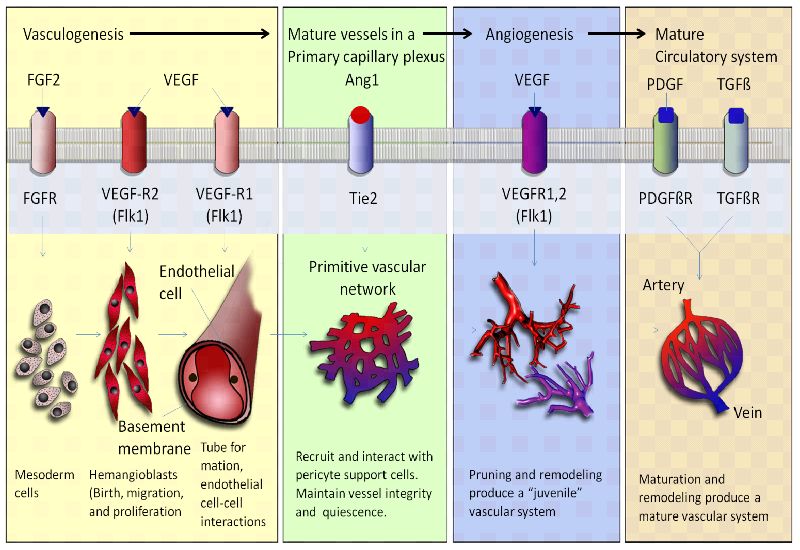
What molecular cues and signaling pathways regulate the growth and branching of blood vessels?
The growth and branching of blood vessels, known as angiogenesis, is regulated by a complex interplay of molecular cues and signaling pathways that guide the formation of new blood vessels in response to various physiological and pathological stimuli. Several key molecular cues and signaling pathways are involved in this process:
VEGF (Vascular Endothelial Growth Factor) Family: VEGF is a central regulator of angiogenesis. It binds to its receptors (VEGFRs) on endothelial cells and promotes their proliferation, migration, and survival. The VEGF family includes various isoforms, such as VEGF-A, VEGF-B, VEGF-C, and VEGF-D, each with specific roles in angiogenesis and lymphangiogenesis.
FGF (Fibroblast Growth Factor) Family: FGFs, particularly FGF-2 and FGF-9, stimulate endothelial cell proliferation and migration. They also induce the release of proteases that help remodel the extracellular matrix to create paths for new vessel growth.
Notch Signaling: Notch signaling is crucial for maintaining proper vascular sprouting and branching. Delta-like ligands (DLLs) on the tip cells of growing vessels interact with Notch receptors on neighboring stalk cells, promoting stalk cell fate and inhibiting tip cell behaviors.
Ephrin-Eph Signaling: Ephrins and their Eph receptors play a role in guiding angiogenic sprouting and vessel branching. The interactions between Ephs on endothelial cells and ephrins on adjacent cells help establish vessel boundaries and prevent excessive vessel growth.
Angiopoietins and Tie Receptors: Angiopoietin-1 (Ang1) and Angiopoietin-2 (Ang2) interact with Tie receptors on endothelial cells to regulate vessel stabilization and remodeling. Ang1 promotes vessel maturation, while Ang2 destabilizes vessels, preparing them for sprouting.
PDGF (Platelet-Derived Growth Factor): PDGF recruits pericytes and smooth muscle cells to stabilize newly formed blood vessels. Pericytes help to reinforce vessel walls and prevent leakage.
Semaphorins and Plexins: Semaphorins guide vessel growth by repelling or attracting endothelial cells. They interact with Plexin receptors to influence angiogenic processes.
Hedgehog Signaling: Hedgehog signaling plays a role in angiogenesis by influencing endothelial cell behavior and vessel branching during development and tissue repair.
Integrins and Cell-ECM Interactions: Integrin-mediated interactions between endothelial cells and the extracellular matrix guide vessel migration, alignment, and branching.
Hypoxia and HIF (Hypoxia-Inducible Factor) Pathway: Low oxygen levels induce the expression of HIF, which upregulates the production of angiogenic factors like VEGF in response to tissue hypoxia.
Plasminogen Activation System: Plasminogen activators and plasmin play a role in angiogenesis by degrading the extracellular matrix and facilitating endothelial cell migration during vessel sprouting.
These signaling pathways and molecular cues interact in a highly coordinated manner to regulate various aspects of blood vessel growth and branching. They respond to tissue-specific needs, developmental cues, and pathological conditions, ensuring the proper formation of functional blood vessel networks that support tissue growth, repair, and homeostasis.
What mechanisms for angiogenesis and vasculogenesis had to be instantiated to ensure proper oxygen and nutrient supply to developing tissues?
Hypoxia-Induced Signaling: Oxygen deficiency triggers the upregulation of hypoxia-inducible factors (HIFs), which stimulate the production of angiogenic factors like VEGF-A. This response initiates angiogenesis by promoting endothelial cell proliferation, migration, and vessel sprouting to supply oxygen and nutrients to hypoxic tissues.
Extracellular Matrix Remodeling: During angiogenesis, proteases are activated to degrade the extracellular matrix, creating paths for endothelial cell migration and vessel sprouting. This remodeling allows vessels to extend into tissues where oxygen and nutrients are needed.
Chemoattractant Gradients: Pro-angiogenic factors, such as VEGF, form gradients that guide endothelial cell migration towards areas of higher concentration. This directional migration ensures that new vessels form in regions with the greatest need for oxygen and nutrients.
Tip Cell and Stalk Cell Differentiation: Developing blood vessels exhibit tip cells at the leading edge and stalk cells behind them. Tip cells guide vessel growth, while stalk cells proliferate and form the vessel trunk. Proper balance between tip and stalk cell behaviors ensures efficient vessel sprouting and branching.
Pericyte and Smooth Muscle Cell Recruitment: During vessel maturation, pericytes and smooth muscle cells are recruited to stabilize vessel walls and regulate blood flow. This prevents vessel collapse and optimizes oxygen and nutrient delivery to tissues.
Branching and Anastomosis: Angiogenic vessels branch and form anastomoses (connections) to create an interconnected network. This network ensures redundancy and efficient distribution of oxygen and nutrients throughout tissues.
Remodeling and Maturation: Angiogenic vessels undergo maturation and remodeling processes, including endothelial cell-cell junction formation and basement membrane deposition. These processes enhance vessel stability and functionality, improving oxygen and nutrient delivery.
Angiopoietin-Tie Signaling: Angiopoietins and their Tie receptors influence vessel stabilization and maturation. Ang1 promotes pericyte recruitment and vessel maturation, optimizing nutrient and oxygen transport.
Functional Adaptation: Developing tissues release angiogenic factors in response to local metabolic demands. This adaptive response ensures that new vessels form where they are most needed for adequate oxygen and nutrient supply.
Vessel Diameter Regulation: Vessel diameter and permeability are regulated to match tissue demands. Small-diameter vessels provide higher oxygen exchange rates, while larger vessels accommodate greater blood flow and nutrient delivery.
The instantiation of these mechanisms ensures that developing tissues receive sufficient oxygen and nutrients by facilitating the establishment of an intricate vascular network. Properly regulated angiogenesis and vasculogenesis enable tissues to grow and develop while maintaining physiological homeostasis.
Differences between Angiogenesis and Vasculogenesis
Vasculogenesis and angiogenesis are two distinct but interconnected processes involved in the formation and development of blood vessels within an organism. While they share similarities, they differ in their timing, mechanisms, and contexts.
Vasculogenesis
Vasculogenesis is the process by which new blood vessels are formed de novo (from scratch) during embryonic development. It primarily occurs during the early stages of embryogenesis when the embryo is a cluster of undifferentiated cells. Here are the key differences:
Timing: Vasculogenesis occurs very early in embryonic development, often before the formation of major organs. It involves the initial assembly of the primary vascular network.
Cell Origin: During vasculogenesis, endothelial precursor cells, called angioblasts, differentiate from mesodermal cells. These angioblasts aggregate and coalesce to form the primitive blood vessels.
Organogenesis: Vasculogenesis is closely associated with organogenesis. As organs begin to develop, the primary vascular network formed by vasculogenesis provides the foundation for the subsequent growth and development of organs.
Vessel Formation: In vasculogenesis, angioblasts aggregate to form blood vessel-like structures, which then coalesce to create the primary capillary plexus. This process involves both endothelial cell differentiation and organization into vessel-like structures.
Angiogenesis
Angiogenesis is the process of forming new blood vessels from preexisting ones. It is a more refined and specialized process that occurs during various developmental stages and in response to specific needs in the body. Here are the key differences:
Timing: Angiogenesis can occur during both embryonic development and postnatal stages. It plays a role in tissue growth, wound healing, and other processes throughout an organism's life.
Cell Origin: During angiogenesis, existing endothelial cells in preexisting blood vessels are activated to proliferate, migrate, and remodel, forming new blood vessels. It involves the expansion and remodeling of existing vascular networks.
Tissue Growth and Repair: Angiogenesis is essential for tissue growth, repair, and regeneration. It allows for the expansion of the vascular network to supply nutrients and oxygen to growing tissues or to aid in healing wounded tissues.
Initiation: Angiogenesis is initiated in response to specific signals, such as hypoxia (low oxygen levels) or growth factors released during tissue injury. These signals activate endothelial cells in existing vessels to sprout, migrate, and form new vessels.
Is there scientific evidence supporting the idea that the intricate processes of angiogenesis and vasculogenesis evolved?
Functional Interdependence: Angiogenesis and vasculogenesis involve multiple interdependent steps, including cell migration, proliferation, signaling, and ECM remodeling. It's challenging to envision how each step could evolve independently, as many components are needed for the process to be functional.
Complex Signaling Networks: The successful formation of blood vessels requires precise coordination of multiple signaling pathways. These pathways involve intricate interactions between growth factors, cell adhesion molecules, and transcription factors. For angiogenesis and vasculogenesis to work, these pathways need to be in place and functional simultaneously.
Required Structures: Blood vessels require a certain structural complexity to function properly. The vessels need to be able to transport blood efficiently, withstand mechanical forces, and maintain barrier properties. These structural requirements make it unlikely that partially developed vessels or intermediate stages would provide any selective advantage.
Energy and Resource Costs: Developing and maintaining blood vessels is metabolically costly. Evolution requires that intermediate stages offer some selective advantage to the organism. If the intermediate stages lack functionality and only incur energy and resource costs, they are less likely to be favored by natural selection.
Regulation and Control: The processes of angiogenesis and vasculogenesis need to be tightly regulated. Failure to regulate these processes properly can lead to diseases such as cancer, where blood vessel growth is uncontrolled. The precise regulation required for these processes to function accurately raises questions about how this regulation could evolve incrementally.
Cell-Cell Interactions: The formation of blood vessels involves intricate cell-cell interactions and communication. Cells need to respond to signals from neighboring cells and the microenvironment. Evolutionary intermediates that lack the ability to properly communicate and interact might not provide any adaptive advantage.
Emergence of Blood Cells: Blood vessels require specialized blood cells (endothelial cells and blood-forming cells) to function. The evolution of these cell types alongside the vessels raises questions about their origin and development in a stepwise manner.
Irreducibility and Interdependence of the systems to instantiate and operate Angiogenesis and Vasculogenesis
The processes involved in the emergence of angiogenesis are highly interdependent:
Angiogenic Factors and Receptor Proteins: Both are needed to initiate signaling for vessel formation.
Cell Adhesion Molecules and Matrix Remodeling Enzymes: They work together to organize cells and create pathways for growth.
Transcription Factors and Differentiation Genes: Transcription factors activate differentiation genes, guiding cell specialization.
Signaling Pathways and Guidance Proteins: Signaling cues guide cells via guidance proteins, requiring functional pathways.
Cytoskeletal Regulators and Cell Signaling: Proper cell migration relies on regulated signaling and coordinated cytoskeletal changes.
Apoptosis and Survival Regulators: Balancing cell numbers during vessel formation involves coordinated apoptosis and survival.
Cell-Cell Communication and Signaling Pathways: Communication depends on functional signaling, enabling coordinated cell behaviors.
Cell Signaling Pathways and Cytoskeletal Regulation: Effective cell migration requires synchronized signaling and cytoskeletal changes.
Epigenetic Priming and Histone Modifications: Epigenetic priming sets the stage for histone modifications to activate vessel-related genes. Both are essential for creating a conducive chromatin environment.
DNA Methylation Dynamics and Non-Coding RNA Regulation: DNA methylation patterns and non-coding RNAs work together to regulate gene expression. Non-coding RNAs could influence DNA methylation patterns, fine-tuning gene activation during vessel formation.
Imprinting and Allelic Regulation: Imprinting relies on specific epigenetic marks that guide cell roles. Allelic regulation ensures proper vessel assembly by assigning distinct roles based on epigenetic marks.
Epigenetic Inheritance and Temporal Regulation: Epigenetic inheritance and temporal regulation are linked. The ability to inherit epigenetic information aids the precise timing of vessel-related processes.
Suppression of Anti-Angiogenic Factors and Chromatin Remodeling: Suppressing anti-angiogenic genes and proper chromatin remodeling go hand in hand. Both are necessary for unobstructed vessel formation.
Epigenetic Regulation of Maturation and Sensing of Cues: The regulation of vascular maturation and sensing cues is interconnected. Epigenetic marks guide vessel maturation while sensing cues allow adaptive vessel formation.
Basic Growth Factor and Receptor Pathways: These pathways rely on each other to stimulate cell behaviors, such as proliferation and migration, essential for vessel formation.
Chemotaxis and Adhesion Pathways: Chemotaxis guides cell movement, while adhesion pathways facilitate cell organization. Both are necessary for coordinated vessel formation.
Cytoskeletal Remodeling and Cell-Cell Communication: Cytoskeletal changes are directed by signaling pathways and influence cell movement. Effective cell-cell communication ensures coordinated behavior during angiogenesis.
Apoptosis and Survival Pathways: Balancing apoptosis and survival is crucial for proper vessel formation. Both pathways work together to determine cell numbers and roles.
Extracellular Matrix Signaling and Ligand-Receptor Pairs: Cells respond to extracellular cues via signaling pathways, which also involve ligand-receptor interactions. These processes work in tandem to guide cell behavior during vessel formation.
VEGF and FGF Signaling: Both pathways promote endothelial cell proliferation and migration. Their combined action is essential for vessel formation.
Notch and Wnt Signaling: These pathways likely cooperated to coordinate endothelial cell differentiation and vessel sprouting, ensuring proper branching patterns.
TGF-β and PDGF Signaling: TGF-β could have influenced endothelial cell differentiation and extracellular matrix remodeling, while PDGF might have recruited supporting cells. Both pathways contribute to vessel stabilization.
ECM, MAPK, and Rho GTPase Signaling: ECM signaling, MAPK pathways, and Rho GTPases could have worked together to guide cell migration, cytoskeletal dynamics, and vessel assembly.
PI3K/AKT and Chemokine Signaling: PI3K/AKT pathway likely collaborated with chemokine signaling to regulate endothelial cell survival, migration, and positioning during vessel sprouting.
Hedgehog and Endothelial-Specific Signaling: Hedgehog signaling and endothelial-specific pathways could have coordinated to regulate vascular patterning, differentiation, proliferation, and tube formation.
Transcriptional Regulatory Code and Epigenetic Regulatory Language: Transcriptional regulation relies on epigenetic modifications for proper gene expression control.
Cis-Regulatory Elements and Signaling Pathway Crosstalk: Cis-regulatory elements ensure spatiotemporal gene expression, influenced by signaling pathway crosstalk.
Cell-Cell Communication Codes and Immune-Endothelial Communication: Effective immune-endothelial communication requires coordinated cell-cell communication codes.
Extracellular Matrix (ECM) Signaling and Vascular Patterning Code: ECM interactions influence vascular patterning, which involves signaling pathways guiding vessel formation.
Stability and Maturation Code and Inflammatory Response Code: Blood vessel maturation is related to inflammation regulation. Proper maturation relies on coordinated immune responses.
Oxygen and Nutrient Sensing Code and Vascular Tone and Homeostasis Code: Both codes contribute to maintaining vessel tone and blood flow in response to changing environmental cues.
Angiogenic Switch Code and Vascular Regression Code: Angiogenesis activation and regression are interconnected processes that require coordinated switches in response to cues.
Wound Healing and Regeneration Code and Vascular Tone and Homeostasis Code: Blood vessels play a role in wound healing and tissue repair, which impacts vessel tone and homeostasis.
The intricacies of creating, developing, and operating complex biological processes like angiogenesis and vasculogenesis highlight a deep interdependence among the manufacturing, signaling, and regulatory codes and languages involved. This complexity raises questions about the feasibility of a stepwise, gradual evolution and instead suggests a purposeful design. The behaviors of cells, including migration, proliferation, and differentiation, are essential for the formation of blood vessels. Without the manufacturing code, cellular actions would lack direction and purpose, rendering vessel formation impossible. Signaling pathways guide cellular behaviors by conveying information between cells. These cues instruct cells on where to migrate, when to proliferate, and how to differentiate. Without the signaling codes, cells would lack the guidance needed to perform their specific tasks. The regulatory codes orchestrate gene expression, determining which genes are activated or suppressed. This precise control ensures that the appropriate genes are turned on to support angiogenesis and vasculogenesis. Without the regulatory codes, genes required for these processes would lack the necessary regulation. Signaling pathways provide cues for cellular behaviors (manufacturing), ensuring that cells migrate, proliferate, and differentiate in a coordinated manner. Without proper signaling, cellular actions would lack direction and purpose. The regulatory codes interpret signaling cues and guide gene expression. Signaling pathways activate transcription factors that influence gene expression levels. Without functional signaling cues, regulatory mechanisms would have no meaningful signals to interpret. Cellular behaviors rely on gene expression controlled by the regulatory code. The manufacturing code's instructions are carried out through the expression of specific genes. Without proper gene regulation, cells wouldn't perform the behaviors required for vessel formation.
From this perspective, the intricate interdependence of these codes suggests a coordinated design, rather than a gradual evolution. The simultaneous emergence of these interconnected systems seems implausible through incremental changes. Instead, it points toward a purposeful design where these components were created all at once, fully operational, to achieve the complex task of forming functional blood vessels. The interconnected nature of the manufacturing, signaling, and regulatory aspects implies that these systems were instantiated together, working in harmony to achieve the intricate process of angiogenesis and vasculogenesis. This kind of interdependence and complexity aligns with the concept of intelligent design, where the coordinated emergence of these mechanisms suggests a guiding intelligence that foresaw the necessary components and their interactions from the outset.
What are veins and arteries interdependent with?
Once veins and arteries are operational, they are interconnected with various intracellular and extracellular systems to ensure proper blood circulation, nutrient delivery, waste removal, and overall physiological function.
Intracellular Systems
Cardiac Muscle Contraction: The heartbeat generated by the cardiac muscle drives blood through the arteries and veins. The function of the circulatory system is closely tied to the heart's pumping action.
Blood Cell Production: The circulatory system relies on the bone marrow to produce red blood cells, white blood cells, and platelets. Arteries and veins transport these cells to various parts of the body.
Hemostasis and Coagulation: The circulatory system interacts with the clotting cascade to prevent excessive bleeding from damaged blood vessels. Platelets, clotting factors, and endothelial cells play roles in this process.
Oxygen and Carbon Dioxide Exchange: Oxygen from inhaled air diffuses into the bloodstream in the lungs and binds to hemoglobin. Carbon dioxide produced by cells is carried by the blood back to the lungs for exhalation.
Extracellular Systems
Respiratory System: The circulatory system collaborates with the respiratory system to ensure oxygen uptake and carbon dioxide removal. Oxygenated blood is transported to cells, and deoxygenated blood is returned to the lungs for oxygen exchange.
Lymphatic System: The lymphatic system helps maintain fluid balance by collecting excess fluid and proteins leaked from blood vessels. Lymphatic vessels return this fluid to the bloodstream, preventing swelling.
Endocrine System: Hormones released by endocrine glands regulate blood pressure, heart rate, and electrolyte balance, all of which impact the function of veins and arteries.
Digestive System: The circulatory system transports nutrients absorbed from the digestive tract to cells throughout the body. Veins and arteries play a role in nutrient distribution.
Nervous System: Autonomic nerves control the diameter of blood vessels, affecting blood pressure and blood flow regulation. Sympathetic and parasympathetic signals influence vessel constriction and dilation.
Immune System: Immune cells are transported via the bloodstream to sites of infection or injury. Blood vessels also play a role in immune cell trafficking and inflammation.
Excretory System: Blood vessels transport waste products to the kidneys for filtration and elimination from the body.
Temperature Regulation: Blood flow to the skin's surface helps regulate body temperature. Arterioles in the skin can dilate to release excess heat or constrict to conserve heat.
The interdependence of veins and arteries with these systems underscores the role of the circulatory system in maintaining overall homeostasis and the proper functioning of various bodily processes. The integration of these systems ensures the coordination of functions necessary for survival and optimal physiological performance.
Premise 1: The interdependence of the circulatory system (veins and arteries) with various intracellular and extracellular systems is crucial for maintaining overall homeostasis and optimal physiological function.
These interconnected systems, including cardiac muscle contraction, blood cell production, hemostasis and coagulation, oxygen and carbon dioxide exchange, respiratory, lymphatic, endocrine, digestive, nervous, immune, excretory systems, and temperature regulation, all rely on each other to perform their functions.
Premise 2: Such intricate interdependence and collaboration among these diverse systems indicate a high level of complexity, precision, and mutual adaptation that appears designed for a holistic and well-coordinated physiological setup.
Conclusion: The profound interdependence and mutual adaptation observed among the circulatory system and the various interconnected systems strongly suggest a carefully designed and integrated setup. The simultaneous emergence of these systems, each playing a crucial role in the function and maintenance of the organism, implies a coordinated plan rather than a gradual, step-by-step process. The functional harmony and coherence across these interdependent systems hint at a deliberate design, ensuring the survival and optimal performance of the organism.
Premise 1: The emergence of blood vessels and angiogenesis involves a multitude of interconnected processes, including angiogenic factors, cell adhesion molecules, transcription factors, signaling pathways, cytoskeletal regulators, and more.
Premise 2: These processes are interdependent, requiring simultaneous and coordinated activation to ensure proper vessel formation, maintenance, and operation.
Conclusion: The intricate interdependence among these processes strongly suggests that they cannot have evolved step by step, as isolated mechanisms or codes, but must have been designed and instantiated together from scratch to function harmoniously and achieve the complex goal of creating, developing, and operating a functional vascular system.
1. Prinz, R. (2023). biological codes: a field guide for code hunters. biological Theory. https://doi.org/10.1007/s13752-023-00444-2
Angiogenesis and vasculogenesis are essential processes involved in the formation and maintenance of blood vessels within biological systems. Angiogenesis refers to the formation of new blood vessels from preexisting ones. It plays a crucial role in various biological contexts, such as wound healing, tissue regeneration, and development. Angiogenesis produces a network of blood vessels that supply nutrients and oxygen to tissues, remove waste products, and facilitate the exchange of molecules between the bloodstream and surrounding cells. This process is particularly important in embryonic development, tissue repair, and growth, as well as in conditions like cancer where new blood vessels support tumor growth and metastasis. Vasculogenesis is the process by which new blood vessels are formed de novo from endothelial progenitor cells. It is particularly significant during embryonic development when the cardiovascular system is initially established. Vasculogenesis contributes to the formation of the primary vascular plexus, which serves as a scaffold for further vascular remodeling and the eventual development of a mature vascular network. Defects in vasculogenesis can lead to severe developmental abnormalities. Angiogenesis and vasculogenesis are fundamental for the survival and function of complex multicellular organisms. They enable efficient transport of nutrients, oxygen, hormones, and immune cells throughout the body. These processes are vital for tissue growth, repair, and regeneration, as well as for maintaining proper physiological functions. Additionally, angiogenesis plays a role in various diseases, such as cancer, where excessive or aberrant blood vessel formation supports tumor growth. Understanding the molecular mechanisms underlying angiogenesis and vasculogenesis has implications for developing therapeutic strategies for conditions involving abnormal blood vessel formation or inadequate blood supply to tissues.
How do angiogenesis and vasculogenesis contribute to the establishment of blood vessel networks during embryonic development?
Angiogenesis and vasculogenesis are essential processes that contribute to the establishment of blood vessel networks during embryonic development. These processes involve the formation, expansion, and remodeling of blood vessels, which are crucial for supplying nutrients, oxygen, and other essential molecules to developing tissues and organs. Here's how angiogenesis and vasculogenesis work together to create functional vascular systems:
Vasculogenesis
Formation of Blood Islands: Vasculogenesis begins with the formation of blood islands, which are clusters of angioblasts (precursor cells) that differentiate into endothelial cells, the building blocks of blood vessels.
Angioblast Migration and Aggregation: Angioblasts migrate to specific areas in the developing embryo, guided by chemical signals and gradients. They aggregate to form endothelial cords or tubes, which serve as the initial structures of blood vessels.
Vascular Lumen Formation: The endothelial cords undergo lumenization, during which they develop a central channel, or lumen. This lumen becomes the pathway for blood flow.
Hemangioblast Differentiation: Some angioblasts differentiate into hemangioblasts, which give rise to both endothelial cells and blood cells. This connection between blood vessel formation and blood cell production is crucial for the functional development of the circulatory system.
Angiogenesis
Sprouting Angiogenesis: In regions where tissues require increased blood supply, endothelial cells from existing vessels start to sprout out in response to pro-angiogenic signals, such as growth factors like VEGF (vascular endothelial growth factor).
Migration and Proliferation: Sprouting endothelial cells migrate towards the source of pro-angiogenic signals and proliferate to form new endothelial sprouts.
Lumen Formation: Similar to vasculogenesis, the endothelial sprouts undergo lumenization to form functional tubular structures.
Anastomosis: The newly formed sprouts elongate and connect with adjacent sprouts, leading to the establishment of interconnected networks of blood vessels. This anastomosis process creates a functional circulation system.
Stabilization: Pericytes and smooth muscle cells are recruited to the developing vessels to provide structural support and stability to the new blood vessels.
Appearance of angiogenesis and vasculogenesis in the evolutionary timeline
The appearance of angiogenesis and vasculogenesis in the evolutionary timeline is a complex topic that involves speculation and ongoing research. While the exact timing is not definitively known, scientists have proposed hypotheses about when these processes might have emerged based on comparative studies and the fossil record. Angiogenesis and vasculogenesis are fundamental processes for the development and maintenance of blood vessels in organisms. Angiogenesis involves the formation of new blood vessels from preexisting ones, while vasculogenesis refers to the de novo formation of blood vessels from endothelial precursor cells.
Early Life Forms (Prokaryotes): These simple organisms did not possess complex vascular systems or mechanisms like angiogenesis and vasculogenesis. Their nutrient exchange and waste removal were likely facilitated by direct diffusion through cell membranes due to their small size and relatively simple structure.
Simple Eukaryotes: As organisms supposedly evolved into more complex eukaryotic forms, some multicellular organisms would have started to develop basic mechanisms to transport nutrients and waste products. However, true vascular systems had not yet emerged, and mechanisms like diffusion and simple tissue organization served these early organisms.
Invertebrates: The appearance of more complex invertebrates would have marked the transition toward more developed circulatory systems. Many invertebrates have open circulatory systems where blood is pumped into body cavities, allowing for nutrient exchange. These systems would have involved rudimentary precursor processes that could be considered primitive forms of angiogenesis.
Vertebrates: The emergence of vertebrates would have brought about more sophisticated circulatory systems, including closed systems with dedicated blood vessels. While the exact point at which angiogenesis and vasculogenesis evolved in vertebrate history is unclear, they would have played essential roles in the development of more advanced vascular systems.
Early Vertebrates: Over time, vertebrates would have evolved into increasingly complex cardiovascular systems. The emergence of angiogenesis and vasculogenesis would have accompanied the need for more efficient nutrient delivery, waste removal, and tissue repair. These processes would have allowed vertebrates to sustain larger and more metabolically active bodies.
De Novo Genetic Information, necessary to instantiate angiogenesis and vasculogenesis
The hypothetical emergence of angiogenesis, the process of forming new blood vessels from scratch in the development of organisms that didn't previously possess a vascular system, would have required the addition of specific genetic information to enable this complex process. While the exact genetic changes are speculative, here are some potential additions or modifications to genetic information that could have been involved in the evolution of angiogenesis:
Angiogenic Factors: The development of angiogenesis from scratch would have necessitated the evolution of genes encoding angiogenic factors, such as rudimentary versions of vascular endothelial growth factors (VEGFs). These factors would act as signaling molecules to initiate vessel formation.
Receptor Proteins: The emergence of angiogenesis would have required the development of new receptor proteins on hypothetical endothelial-like precursor cells. These receptors would allow cells to detect and respond to angiogenic factors, initiating the signaling cascades necessary for vessel formation.
Cell Adhesion Molecules: As cells begin to organize into blood vessels, new genetic information could have been necessary to generate primitive cell adhesion molecules. These molecules would facilitate cell-cell interactions and the formation of vessel-like structures.
Matrix Remodeling Enzymes: The initial formation of blood vessels from scratch would involve breaking down and restructuring extracellular matrix. Genes encoding matrix remodeling enzymes could have evolved to allow cells to create pathways for vessel growth.
Transcription Factors: The evolution of angiogenesis would require new transcription factors that activate gene expression programs specific to vessel formation. These factors would regulate the expression of genes involved in cell migration, proliferation, and differentiation.
Guidance Proteins: To direct the movement of primitive endothelial-like cells toward the formation of blood vessels, hypothetical guidance proteins could have evolved to provide directional cues.
Signaling Molecules and Pathways: New genetic information would be needed to establish the initial signaling pathways that trigger cellular responses, such as migration, proliferation, and differentiation, during angiogenesis.
Cytoskeletal Regulators: The development of angiogenesis from scratch would require genes that control cytoskeletal dynamics, enabling cell movement, migration, and organization into vessel-like structures.
Apoptosis and Survival Regulators: As cells form blood vessels, mechanisms for balancing cell survival and cell death would be crucial. Genes involved in apoptosis and survival pathways might have been required.
Differentiation and Specification Genes: The evolution of angiogenesis would involve the development of genes that specify and differentiate precursor cells into endothelial-like cells with distinct roles in vessel formation.
Cell-Cell Communication Molecules: New genetic information could have been necessary to allow cells to communicate and coordinate their behaviors during the formation of blood vessels.
Cell Signaling Pathways for Coordination: To ensure proper coordination among endothelial-like cells, genetic information might have evolved to create rudimentary cell signaling pathways for communication and synchronization.
Epigenetic Regulatory Mechanisms necessary to be instantiated
The hypothetical emergence of angiogenesis "from scratch," meaning the evolution of blood vessel formation in organisms that previously lacked such a system, would have required the establishment of new epigenetic regulations to coordinate the complex cellular changes necessary for vessel development.
Epigenetic Priming for Vascular Precursors: The initial step might have involved the establishment of epigenetic marks that prime certain precursor cells for vascular differentiation. These marks could create a permissive chromatin environment for angiogenesis-related genes.
Histone Modifications for Vessel Formation: The evolution of angiogenesis would have necessitated the emergence of specific histone modifications that activate genes involved in vessel development. Histone acetylation, for example, could enhance the expression of genes related to endothelial differentiation and tube formation.
DNA Methylation Dynamics: The formation of blood vessels would require the establishment of specific DNA methylation patterns. New patterns could regulate the expression of genes essential for angiogenesis, fine-tuning their activation during different stages of vessel formation.
Non-Coding RNA-Mediated Regulation: The evolution of angiogenesis might have led to the emergence of non-coding RNAs, such as microRNAs or long non-coding RNAs, which could interact with chromatin-modifying complexes to regulate the expression of genes associated with vessel development.
Imprinting and Allelic Regulation: To ensure proper vessel assembly, the evolution of angiogenesis could involve the establishment of allele-specific epigenetic marks that guide cells to adopt specific roles during vessel formation.
Epigenetic Inheritance of Vascular Patterns: The ability to pass down epigenetic information related to vessel formation could have evolved, allowing subsequent generations to inherit the regulatory marks necessary for angiogenesis.
Temporal Epigenetic Regulations: The coordinated development of angiogenesis would require the evolution of epigenetic mechanisms that act as molecular "clocks," ensuring the precise timing of vessel-related processes.
Suppression of Anti-Angiogenic Factors: New epigenetic regulations could have evolved to suppress the expression of genes encoding anti-angiogenic factors, ensuring that the vessel development process is not hindered.
Chromatin Remodeling for Vessel Assembly: As endothelial-like cells come together to form vessels, new epigenetic mechanisms might have been required to regulate chromatin remodeling, facilitating proper cell-cell interactions and tube formation.
Epigenetic Regulation of Vascular Maturation: Epigenetic marks might have been necessary to guide the maturation and stabilization of newly formed vessels, including the recruitment of support cells like pericytes and smooth muscle cells.
Epigenetic Sensing of Environmental Cues: The evolution of angiogenesis would likely require epigenetic mechanisms that enable cells to sense and respond to environmental signals, allowing for adaptive vessel formation in different contexts.
Signaling Pathways necessary to create, and maintain angiogenesis and vasculogenesis
If we're considering the emergence of angiogenesis "from scratch," i.e., the initial development of the process in organisms that didn't previously have any vascular system, potential signaling pathways that would have had to be involved in the hypothetical evolution of angiogenesis: are:
Basic Growth Factor and Receptor Pathways: The emergence of angiogenesis would have required the evolution of basic growth factor pathways and receptor systems that stimulate cell proliferation, migration, and differentiation. Simple signaling cascades involving ligands and receptors might have formed the foundation for more complex angiogenic signaling.
Chemotaxis Pathways: Hypothetically, rudimentary chemotactic signaling pathways could have evolved to guide primitive endothelial-like cells toward specific gradients of factors that promote cell movement. These could have been primitive versions of later-developing pathways.
Adhesion Pathways: Early angiogenesis would have necessitated cell adhesion and migration. Signaling pathways related to cell adhesion molecules could have emerged to facilitate endothelial cell movement and organization into nascent blood vessels.
Cytoskeletal Remodeling Pathways: The evolution of angiogenesis would likely have involved mechanisms for altering cell shape and movement. Signaling pathways related to the cytoskeleton, such as actin and microtubule dynamics, could have been essential.
Cell-Cell Communication Pathways: Basic cell-cell communication pathways might have developed to allow primitive endothelial cells to coordinate their behaviors during the initial stages of vessel formation.
Apoptosis and Survival Pathways: As vessel formation requires the precise balance between cell death (apoptosis) and cell survival, hypothetical pathways regulating these processes might have been crucial.
Initial Extracellular Matrix Signaling: Signaling pathways involved in responding to the extracellular environment could have emerged to guide cells to form structures resembling the rudimentary precursor of blood vessels.
Evolution of Ligand-Receptor Pairs: New ligand-receptor pairs might have evolved, allowing cells to sense and respond to cues that promote vessel formation.
The emergence of angiogenesis, the process of forming new blood vessels from preexisting ones, would have involved the establishment and modification of specific signaling pathways to coordinate the complex cellular changes required for vessel formation. While the exact signaling pathways are speculative, here are some potential pathways that could have been involved in the emergence of angiogenesis:
VEGF Signaling Pathway: Vascular endothelial growth factor (VEGF) is a central regulator of angiogenesis. The VEGF signaling pathway involves the binding of VEGF to its receptors (VEGFRs) on endothelial cells. This activates downstream signaling cascades that promote endothelial cell proliferation, migration, and tube formation.
FGF Signaling Pathway: Fibroblast growth factors (FGFs) also play a role in angiogenesis. The FGF signaling pathway, similar to the VEGF pathway, involves FGF ligands binding to their receptors, which triggers signaling events that contribute to endothelial cell proliferation and migration.
Notch Signaling Pathway: The Notch pathway is involved in cell-cell communication and could have played a role in coordinating endothelial cell differentiation and tip cell selection during vessel sprouting.
Wnt Signaling Pathway: The Wnt pathway has diverse roles in development, and its components could have been involved in angiogenesis by influencing endothelial cell behavior and vessel branching patterns.
TGF-β Signaling Pathway: Transforming growth factor-beta (TGF-β) family members could have been implicated in angiogenesis by regulating endothelial cell differentiation and extracellular matrix remodeling.
PDGF Signaling Pathway: Platelet-derived growth factor (PDGF) signaling might have been involved in recruiting pericytes and smooth muscle cells to stabilize and mature newly formed vessels.
ECM Signaling Pathways: Extracellular matrix (ECM) components, including integrins and focal adhesion kinase (FAK), could have participated in transmitting signals that guide endothelial cell migration and vessel assembly.
MAPK Signaling Pathway: Mitogen-activated protein kinase (MAPK) pathways could have been essential for transmitting signals that regulate cell proliferation, survival, and migration during angiogenesis.
PI3K/AKT Signaling Pathway: The phosphatidylinositol 3-kinase (PI3K)/AKT pathway could have been involved in promoting endothelial cell survival, migration, and angiogenesis-related cellular responses.
Rho GTPase Signaling Pathway: Rho GTPases, such as Rho, Rac, and Cdc42, could have participated in regulating cytoskeletal dynamics and cell migration during angiogenesis.
Chemokine Signaling: Chemokines and their receptors might have guided endothelial cell migration and positioning during vessel sprouting.
Hedgehog Signaling Pathway: Hedgehog signaling could have been implicated in regulating vascular patterning and endothelial cell differentiation.
Endothelial-Specific Signaling Pathways: Signaling pathways specifically active in endothelial cells could have evolved to control angiogenesis-related processes like proliferation, migration, and tube formation.
Regulatory codes necessary for maintenance and operation
The hypothetical emergence of blood vessels and angiogenesis would have likely involved the establishment of regulatory codes and languages to coordinate the development, maintenance, and operation of the vascular system. While the exact details are speculative, here are potential regulatory codes and languages that could have been instantiated:
Transcriptional Regulatory Code: The evolution of blood vessels would require a transcriptional regulatory code involving specific DNA sequences, transcription factors, and regulatory elements that control the expression of genes involved in vessel development, maintenance, and function.
Cis-Regulatory Elements: Enhancers, promoters, and other cis-regulatory elements would need to evolve to ensure proper spatiotemporal expression of angiogenesis-related genes.
Epigenetic Regulatory Language: Epigenetic modifications such as DNA methylation and histone modifications could form an epigenetic regulatory language that guides the activation and repression of genes essential for vascular development and maintenance.
Signaling Pathway Crosstalk: Complex signaling pathways involved in angiogenesis and vascular function would need to communicate and coordinate their activities through a regulatory language that ensures proper cellular responses.
Cell-Cell Communication Codes: As blood vessels involve multiple cell types, a communication code involving cell surface receptors, ligands, and their interactions would be necessary to coordinate cellular behaviors and functions.
Extracellular Matrix (ECM) Signaling: A code involving interactions between cells and the extracellular matrix would regulate processes such as cell adhesion, migration, and signaling.
Vascular Patterning Code: The establishment of hierarchical vessel networks would require a code that guides the formation and branching patterns of blood vessels to ensure efficient distribution of nutrients and oxygen.
Stability and Maturation Code: Blood vessel stabilization and maturation would require a regulatory code involving communication between endothelial cells and support cells (pericytes and smooth muscle cells) to ensure structural integrity.
Oxygen and Nutrient Sensing Code: Blood vessels need to adapt to changing oxygen and nutrient levels. A regulatory code might govern the response of vessels to these fluctuations, ensuring appropriate vessel dilation and constriction.
Immune-Endothelial Communication: Blood vessels interact with the immune system. A code would be needed to regulate the communication between endothelial cells and immune cells, enabling immune surveillance and inflammation regulation.
Inflammatory Response Code: Inflammatory responses and repair processes would require a regulatory code to activate and control specific genes involved in tissue repair and vessel remodeling.
Vascular Tone and Homeostasis Code: Blood pressure and vessel tone need to be tightly regulated. A code would be necessary to balance vasoconstriction and vasodilation to maintain blood flow and homeostasis.
Angiogenic Switch Code: The transition from quiescent vessels to angiogenesis activation would require a code that senses environmental cues and triggers angiogenic responses.
Vascular Regression Code: Vessels need to regress when not needed. A regulatory code would be necessary to initiate vessel regression and tissue remodeling.
Wound Healing and Regeneration Code: Blood vessels play a role in tissue repair. A code would be involved in coordinating vessel-related processes during wound healing and tissue regeneration.
These regulatory codes and languages would have had to emerge to ensure the development, maintenance, and operation of blood vessels. The precise details would depend on the specific context and the genetic and molecular mechanisms that contributed to the emergence of angiogenesis and vascular systems.
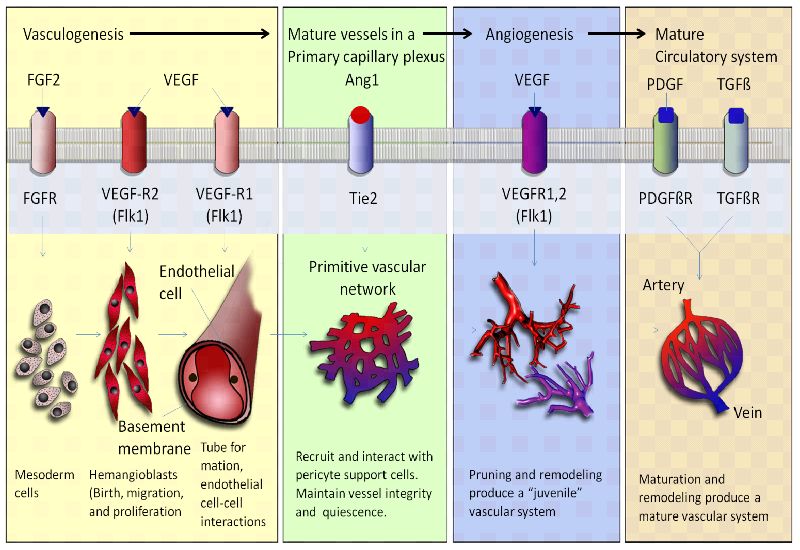
What molecular cues and signaling pathways regulate the growth and branching of blood vessels?
The growth and branching of blood vessels, known as angiogenesis, is regulated by a complex interplay of molecular cues and signaling pathways that guide the formation of new blood vessels in response to various physiological and pathological stimuli. Several key molecular cues and signaling pathways are involved in this process:
VEGF (Vascular Endothelial Growth Factor) Family: VEGF is a central regulator of angiogenesis. It binds to its receptors (VEGFRs) on endothelial cells and promotes their proliferation, migration, and survival. The VEGF family includes various isoforms, such as VEGF-A, VEGF-B, VEGF-C, and VEGF-D, each with specific roles in angiogenesis and lymphangiogenesis.
FGF (Fibroblast Growth Factor) Family: FGFs, particularly FGF-2 and FGF-9, stimulate endothelial cell proliferation and migration. They also induce the release of proteases that help remodel the extracellular matrix to create paths for new vessel growth.
Notch Signaling: Notch signaling is crucial for maintaining proper vascular sprouting and branching. Delta-like ligands (DLLs) on the tip cells of growing vessels interact with Notch receptors on neighboring stalk cells, promoting stalk cell fate and inhibiting tip cell behaviors.
Ephrin-Eph Signaling: Ephrins and their Eph receptors play a role in guiding angiogenic sprouting and vessel branching. The interactions between Ephs on endothelial cells and ephrins on adjacent cells help establish vessel boundaries and prevent excessive vessel growth.
Angiopoietins and Tie Receptors: Angiopoietin-1 (Ang1) and Angiopoietin-2 (Ang2) interact with Tie receptors on endothelial cells to regulate vessel stabilization and remodeling. Ang1 promotes vessel maturation, while Ang2 destabilizes vessels, preparing them for sprouting.
PDGF (Platelet-Derived Growth Factor): PDGF recruits pericytes and smooth muscle cells to stabilize newly formed blood vessels. Pericytes help to reinforce vessel walls and prevent leakage.
Semaphorins and Plexins: Semaphorins guide vessel growth by repelling or attracting endothelial cells. They interact with Plexin receptors to influence angiogenic processes.
Hedgehog Signaling: Hedgehog signaling plays a role in angiogenesis by influencing endothelial cell behavior and vessel branching during development and tissue repair.
Integrins and Cell-ECM Interactions: Integrin-mediated interactions between endothelial cells and the extracellular matrix guide vessel migration, alignment, and branching.
Hypoxia and HIF (Hypoxia-Inducible Factor) Pathway: Low oxygen levels induce the expression of HIF, which upregulates the production of angiogenic factors like VEGF in response to tissue hypoxia.
Plasminogen Activation System: Plasminogen activators and plasmin play a role in angiogenesis by degrading the extracellular matrix and facilitating endothelial cell migration during vessel sprouting.
These signaling pathways and molecular cues interact in a highly coordinated manner to regulate various aspects of blood vessel growth and branching. They respond to tissue-specific needs, developmental cues, and pathological conditions, ensuring the proper formation of functional blood vessel networks that support tissue growth, repair, and homeostasis.
What mechanisms for angiogenesis and vasculogenesis had to be instantiated to ensure proper oxygen and nutrient supply to developing tissues?
Hypoxia-Induced Signaling: Oxygen deficiency triggers the upregulation of hypoxia-inducible factors (HIFs), which stimulate the production of angiogenic factors like VEGF-A. This response initiates angiogenesis by promoting endothelial cell proliferation, migration, and vessel sprouting to supply oxygen and nutrients to hypoxic tissues.
Extracellular Matrix Remodeling: During angiogenesis, proteases are activated to degrade the extracellular matrix, creating paths for endothelial cell migration and vessel sprouting. This remodeling allows vessels to extend into tissues where oxygen and nutrients are needed.
Chemoattractant Gradients: Pro-angiogenic factors, such as VEGF, form gradients that guide endothelial cell migration towards areas of higher concentration. This directional migration ensures that new vessels form in regions with the greatest need for oxygen and nutrients.
Tip Cell and Stalk Cell Differentiation: Developing blood vessels exhibit tip cells at the leading edge and stalk cells behind them. Tip cells guide vessel growth, while stalk cells proliferate and form the vessel trunk. Proper balance between tip and stalk cell behaviors ensures efficient vessel sprouting and branching.
Pericyte and Smooth Muscle Cell Recruitment: During vessel maturation, pericytes and smooth muscle cells are recruited to stabilize vessel walls and regulate blood flow. This prevents vessel collapse and optimizes oxygen and nutrient delivery to tissues.
Branching and Anastomosis: Angiogenic vessels branch and form anastomoses (connections) to create an interconnected network. This network ensures redundancy and efficient distribution of oxygen and nutrients throughout tissues.
Remodeling and Maturation: Angiogenic vessels undergo maturation and remodeling processes, including endothelial cell-cell junction formation and basement membrane deposition. These processes enhance vessel stability and functionality, improving oxygen and nutrient delivery.
Angiopoietin-Tie Signaling: Angiopoietins and their Tie receptors influence vessel stabilization and maturation. Ang1 promotes pericyte recruitment and vessel maturation, optimizing nutrient and oxygen transport.
Functional Adaptation: Developing tissues release angiogenic factors in response to local metabolic demands. This adaptive response ensures that new vessels form where they are most needed for adequate oxygen and nutrient supply.
Vessel Diameter Regulation: Vessel diameter and permeability are regulated to match tissue demands. Small-diameter vessels provide higher oxygen exchange rates, while larger vessels accommodate greater blood flow and nutrient delivery.
The instantiation of these mechanisms ensures that developing tissues receive sufficient oxygen and nutrients by facilitating the establishment of an intricate vascular network. Properly regulated angiogenesis and vasculogenesis enable tissues to grow and develop while maintaining physiological homeostasis.
Differences between Angiogenesis and Vasculogenesis
Vasculogenesis and angiogenesis are two distinct but interconnected processes involved in the formation and development of blood vessels within an organism. While they share similarities, they differ in their timing, mechanisms, and contexts.
Vasculogenesis
Vasculogenesis is the process by which new blood vessels are formed de novo (from scratch) during embryonic development. It primarily occurs during the early stages of embryogenesis when the embryo is a cluster of undifferentiated cells. Here are the key differences:
Timing: Vasculogenesis occurs very early in embryonic development, often before the formation of major organs. It involves the initial assembly of the primary vascular network.
Cell Origin: During vasculogenesis, endothelial precursor cells, called angioblasts, differentiate from mesodermal cells. These angioblasts aggregate and coalesce to form the primitive blood vessels.
Organogenesis: Vasculogenesis is closely associated with organogenesis. As organs begin to develop, the primary vascular network formed by vasculogenesis provides the foundation for the subsequent growth and development of organs.
Vessel Formation: In vasculogenesis, angioblasts aggregate to form blood vessel-like structures, which then coalesce to create the primary capillary plexus. This process involves both endothelial cell differentiation and organization into vessel-like structures.
Angiogenesis
Angiogenesis is the process of forming new blood vessels from preexisting ones. It is a more refined and specialized process that occurs during various developmental stages and in response to specific needs in the body. Here are the key differences:
Timing: Angiogenesis can occur during both embryonic development and postnatal stages. It plays a role in tissue growth, wound healing, and other processes throughout an organism's life.
Cell Origin: During angiogenesis, existing endothelial cells in preexisting blood vessels are activated to proliferate, migrate, and remodel, forming new blood vessels. It involves the expansion and remodeling of existing vascular networks.
Tissue Growth and Repair: Angiogenesis is essential for tissue growth, repair, and regeneration. It allows for the expansion of the vascular network to supply nutrients and oxygen to growing tissues or to aid in healing wounded tissues.
Initiation: Angiogenesis is initiated in response to specific signals, such as hypoxia (low oxygen levels) or growth factors released during tissue injury. These signals activate endothelial cells in existing vessels to sprout, migrate, and form new vessels.
Is there scientific evidence supporting the idea that the intricate processes of angiogenesis and vasculogenesis evolved?
Functional Interdependence: Angiogenesis and vasculogenesis involve multiple interdependent steps, including cell migration, proliferation, signaling, and ECM remodeling. It's challenging to envision how each step could evolve independently, as many components are needed for the process to be functional.
Complex Signaling Networks: The successful formation of blood vessels requires precise coordination of multiple signaling pathways. These pathways involve intricate interactions between growth factors, cell adhesion molecules, and transcription factors. For angiogenesis and vasculogenesis to work, these pathways need to be in place and functional simultaneously.
Required Structures: Blood vessels require a certain structural complexity to function properly. The vessels need to be able to transport blood efficiently, withstand mechanical forces, and maintain barrier properties. These structural requirements make it unlikely that partially developed vessels or intermediate stages would provide any selective advantage.
Energy and Resource Costs: Developing and maintaining blood vessels is metabolically costly. Evolution requires that intermediate stages offer some selective advantage to the organism. If the intermediate stages lack functionality and only incur energy and resource costs, they are less likely to be favored by natural selection.
Regulation and Control: The processes of angiogenesis and vasculogenesis need to be tightly regulated. Failure to regulate these processes properly can lead to diseases such as cancer, where blood vessel growth is uncontrolled. The precise regulation required for these processes to function accurately raises questions about how this regulation could evolve incrementally.
Cell-Cell Interactions: The formation of blood vessels involves intricate cell-cell interactions and communication. Cells need to respond to signals from neighboring cells and the microenvironment. Evolutionary intermediates that lack the ability to properly communicate and interact might not provide any adaptive advantage.
Emergence of Blood Cells: Blood vessels require specialized blood cells (endothelial cells and blood-forming cells) to function. The evolution of these cell types alongside the vessels raises questions about their origin and development in a stepwise manner.
Irreducibility and Interdependence of the systems to instantiate and operate Angiogenesis and Vasculogenesis
The processes involved in the emergence of angiogenesis are highly interdependent:
Angiogenic Factors and Receptor Proteins: Both are needed to initiate signaling for vessel formation.
Cell Adhesion Molecules and Matrix Remodeling Enzymes: They work together to organize cells and create pathways for growth.
Transcription Factors and Differentiation Genes: Transcription factors activate differentiation genes, guiding cell specialization.
Signaling Pathways and Guidance Proteins: Signaling cues guide cells via guidance proteins, requiring functional pathways.
Cytoskeletal Regulators and Cell Signaling: Proper cell migration relies on regulated signaling and coordinated cytoskeletal changes.
Apoptosis and Survival Regulators: Balancing cell numbers during vessel formation involves coordinated apoptosis and survival.
Cell-Cell Communication and Signaling Pathways: Communication depends on functional signaling, enabling coordinated cell behaviors.
Cell Signaling Pathways and Cytoskeletal Regulation: Effective cell migration requires synchronized signaling and cytoskeletal changes.
Epigenetic Priming and Histone Modifications: Epigenetic priming sets the stage for histone modifications to activate vessel-related genes. Both are essential for creating a conducive chromatin environment.
DNA Methylation Dynamics and Non-Coding RNA Regulation: DNA methylation patterns and non-coding RNAs work together to regulate gene expression. Non-coding RNAs could influence DNA methylation patterns, fine-tuning gene activation during vessel formation.
Imprinting and Allelic Regulation: Imprinting relies on specific epigenetic marks that guide cell roles. Allelic regulation ensures proper vessel assembly by assigning distinct roles based on epigenetic marks.
Epigenetic Inheritance and Temporal Regulation: Epigenetic inheritance and temporal regulation are linked. The ability to inherit epigenetic information aids the precise timing of vessel-related processes.
Suppression of Anti-Angiogenic Factors and Chromatin Remodeling: Suppressing anti-angiogenic genes and proper chromatin remodeling go hand in hand. Both are necessary for unobstructed vessel formation.
Epigenetic Regulation of Maturation and Sensing of Cues: The regulation of vascular maturation and sensing cues is interconnected. Epigenetic marks guide vessel maturation while sensing cues allow adaptive vessel formation.
Basic Growth Factor and Receptor Pathways: These pathways rely on each other to stimulate cell behaviors, such as proliferation and migration, essential for vessel formation.
Chemotaxis and Adhesion Pathways: Chemotaxis guides cell movement, while adhesion pathways facilitate cell organization. Both are necessary for coordinated vessel formation.
Cytoskeletal Remodeling and Cell-Cell Communication: Cytoskeletal changes are directed by signaling pathways and influence cell movement. Effective cell-cell communication ensures coordinated behavior during angiogenesis.
Apoptosis and Survival Pathways: Balancing apoptosis and survival is crucial for proper vessel formation. Both pathways work together to determine cell numbers and roles.
Extracellular Matrix Signaling and Ligand-Receptor Pairs: Cells respond to extracellular cues via signaling pathways, which also involve ligand-receptor interactions. These processes work in tandem to guide cell behavior during vessel formation.
VEGF and FGF Signaling: Both pathways promote endothelial cell proliferation and migration. Their combined action is essential for vessel formation.
Notch and Wnt Signaling: These pathways likely cooperated to coordinate endothelial cell differentiation and vessel sprouting, ensuring proper branching patterns.
TGF-β and PDGF Signaling: TGF-β could have influenced endothelial cell differentiation and extracellular matrix remodeling, while PDGF might have recruited supporting cells. Both pathways contribute to vessel stabilization.
ECM, MAPK, and Rho GTPase Signaling: ECM signaling, MAPK pathways, and Rho GTPases could have worked together to guide cell migration, cytoskeletal dynamics, and vessel assembly.
PI3K/AKT and Chemokine Signaling: PI3K/AKT pathway likely collaborated with chemokine signaling to regulate endothelial cell survival, migration, and positioning during vessel sprouting.
Hedgehog and Endothelial-Specific Signaling: Hedgehog signaling and endothelial-specific pathways could have coordinated to regulate vascular patterning, differentiation, proliferation, and tube formation.
Transcriptional Regulatory Code and Epigenetic Regulatory Language: Transcriptional regulation relies on epigenetic modifications for proper gene expression control.
Cis-Regulatory Elements and Signaling Pathway Crosstalk: Cis-regulatory elements ensure spatiotemporal gene expression, influenced by signaling pathway crosstalk.
Cell-Cell Communication Codes and Immune-Endothelial Communication: Effective immune-endothelial communication requires coordinated cell-cell communication codes.
Extracellular Matrix (ECM) Signaling and Vascular Patterning Code: ECM interactions influence vascular patterning, which involves signaling pathways guiding vessel formation.
Stability and Maturation Code and Inflammatory Response Code: Blood vessel maturation is related to inflammation regulation. Proper maturation relies on coordinated immune responses.
Oxygen and Nutrient Sensing Code and Vascular Tone and Homeostasis Code: Both codes contribute to maintaining vessel tone and blood flow in response to changing environmental cues.
Angiogenic Switch Code and Vascular Regression Code: Angiogenesis activation and regression are interconnected processes that require coordinated switches in response to cues.
Wound Healing and Regeneration Code and Vascular Tone and Homeostasis Code: Blood vessels play a role in wound healing and tissue repair, which impacts vessel tone and homeostasis.
The intricacies of creating, developing, and operating complex biological processes like angiogenesis and vasculogenesis highlight a deep interdependence among the manufacturing, signaling, and regulatory codes and languages involved. This complexity raises questions about the feasibility of a stepwise, gradual evolution and instead suggests a purposeful design. The behaviors of cells, including migration, proliferation, and differentiation, are essential for the formation of blood vessels. Without the manufacturing code, cellular actions would lack direction and purpose, rendering vessel formation impossible. Signaling pathways guide cellular behaviors by conveying information between cells. These cues instruct cells on where to migrate, when to proliferate, and how to differentiate. Without the signaling codes, cells would lack the guidance needed to perform their specific tasks. The regulatory codes orchestrate gene expression, determining which genes are activated or suppressed. This precise control ensures that the appropriate genes are turned on to support angiogenesis and vasculogenesis. Without the regulatory codes, genes required for these processes would lack the necessary regulation. Signaling pathways provide cues for cellular behaviors (manufacturing), ensuring that cells migrate, proliferate, and differentiate in a coordinated manner. Without proper signaling, cellular actions would lack direction and purpose. The regulatory codes interpret signaling cues and guide gene expression. Signaling pathways activate transcription factors that influence gene expression levels. Without functional signaling cues, regulatory mechanisms would have no meaningful signals to interpret. Cellular behaviors rely on gene expression controlled by the regulatory code. The manufacturing code's instructions are carried out through the expression of specific genes. Without proper gene regulation, cells wouldn't perform the behaviors required for vessel formation.
From this perspective, the intricate interdependence of these codes suggests a coordinated design, rather than a gradual evolution. The simultaneous emergence of these interconnected systems seems implausible through incremental changes. Instead, it points toward a purposeful design where these components were created all at once, fully operational, to achieve the complex task of forming functional blood vessels. The interconnected nature of the manufacturing, signaling, and regulatory aspects implies that these systems were instantiated together, working in harmony to achieve the intricate process of angiogenesis and vasculogenesis. This kind of interdependence and complexity aligns with the concept of intelligent design, where the coordinated emergence of these mechanisms suggests a guiding intelligence that foresaw the necessary components and their interactions from the outset.
What are veins and arteries interdependent with?
Once veins and arteries are operational, they are interconnected with various intracellular and extracellular systems to ensure proper blood circulation, nutrient delivery, waste removal, and overall physiological function.
Intracellular Systems
Cardiac Muscle Contraction: The heartbeat generated by the cardiac muscle drives blood through the arteries and veins. The function of the circulatory system is closely tied to the heart's pumping action.
Blood Cell Production: The circulatory system relies on the bone marrow to produce red blood cells, white blood cells, and platelets. Arteries and veins transport these cells to various parts of the body.
Hemostasis and Coagulation: The circulatory system interacts with the clotting cascade to prevent excessive bleeding from damaged blood vessels. Platelets, clotting factors, and endothelial cells play roles in this process.
Oxygen and Carbon Dioxide Exchange: Oxygen from inhaled air diffuses into the bloodstream in the lungs and binds to hemoglobin. Carbon dioxide produced by cells is carried by the blood back to the lungs for exhalation.
Extracellular Systems
Respiratory System: The circulatory system collaborates with the respiratory system to ensure oxygen uptake and carbon dioxide removal. Oxygenated blood is transported to cells, and deoxygenated blood is returned to the lungs for oxygen exchange.
Lymphatic System: The lymphatic system helps maintain fluid balance by collecting excess fluid and proteins leaked from blood vessels. Lymphatic vessels return this fluid to the bloodstream, preventing swelling.
Endocrine System: Hormones released by endocrine glands regulate blood pressure, heart rate, and electrolyte balance, all of which impact the function of veins and arteries.
Digestive System: The circulatory system transports nutrients absorbed from the digestive tract to cells throughout the body. Veins and arteries play a role in nutrient distribution.
Nervous System: Autonomic nerves control the diameter of blood vessels, affecting blood pressure and blood flow regulation. Sympathetic and parasympathetic signals influence vessel constriction and dilation.
Immune System: Immune cells are transported via the bloodstream to sites of infection or injury. Blood vessels also play a role in immune cell trafficking and inflammation.
Excretory System: Blood vessels transport waste products to the kidneys for filtration and elimination from the body.
Temperature Regulation: Blood flow to the skin's surface helps regulate body temperature. Arterioles in the skin can dilate to release excess heat or constrict to conserve heat.
The interdependence of veins and arteries with these systems underscores the role of the circulatory system in maintaining overall homeostasis and the proper functioning of various bodily processes. The integration of these systems ensures the coordination of functions necessary for survival and optimal physiological performance.
Premise 1: The interdependence of the circulatory system (veins and arteries) with various intracellular and extracellular systems is crucial for maintaining overall homeostasis and optimal physiological function.
These interconnected systems, including cardiac muscle contraction, blood cell production, hemostasis and coagulation, oxygen and carbon dioxide exchange, respiratory, lymphatic, endocrine, digestive, nervous, immune, excretory systems, and temperature regulation, all rely on each other to perform their functions.
Premise 2: Such intricate interdependence and collaboration among these diverse systems indicate a high level of complexity, precision, and mutual adaptation that appears designed for a holistic and well-coordinated physiological setup.
Conclusion: The profound interdependence and mutual adaptation observed among the circulatory system and the various interconnected systems strongly suggest a carefully designed and integrated setup. The simultaneous emergence of these systems, each playing a crucial role in the function and maintenance of the organism, implies a coordinated plan rather than a gradual, step-by-step process. The functional harmony and coherence across these interdependent systems hint at a deliberate design, ensuring the survival and optimal performance of the organism.
Premise 1: The emergence of blood vessels and angiogenesis involves a multitude of interconnected processes, including angiogenic factors, cell adhesion molecules, transcription factors, signaling pathways, cytoskeletal regulators, and more.
Premise 2: These processes are interdependent, requiring simultaneous and coordinated activation to ensure proper vessel formation, maintenance, and operation.
Conclusion: The intricate interdependence among these processes strongly suggests that they cannot have evolved step by step, as isolated mechanisms or codes, but must have been designed and instantiated together from scratch to function harmoniously and achieve the complex goal of creating, developing, and operating a functional vascular system.
1. Prinz, R. (2023). biological codes: a field guide for code hunters. biological Theory. https://doi.org/10.1007/s13752-023-00444-2
Last edited by Otangelo on Sun Sep 03, 2023 11:08 am; edited 3 times in total