https://reasonandscience.catsboard.com/t1339-fine-tuning-of-the-fundamental-forces-of-the-universe
Believing that tossing a coin and having it come up heads 10 septillion times in a row defies common sense. That would be at least the odds to get the four fundamental forces right to have a life-permitting universe. I don't think so. I do have not enough faith to be an atheist.
The fundamental forces of the universe are finely adjusted to permit life
1. If the strong nuclear force, the force that binds protons and neutrons together in an atom, had been stronger or weaker by as little as 5%, life would be impossible.
If gravity had been stronger or weaker by 1 part in 1040, then life-sustaining stars like the sun could not exist. This would most likely make life impossible.
If the neutron were not about 1.001 times the mass of the proton, all protons would have decayed into neutrons or all neutrons would have decayed into protons, and thus life would not be possible.
If the electromagnetic force were slightly stronger or weaker, life would be impossible, for a variety of different reasons.
If the strong nuclear force were changed 0.4%, carbon-based life appears to be impossible, since all the stars then would produce either almost solely carbon or oxygen, but could not produce both elements.”
2. Imaginatively, one could think of each instance of fine-tuning as a radio dial: unless all the dials are set exactly right, life would be impossible. Or, one could think of the initial conditions of the universe and the fundamental parameters of physics as a dart board that fills the whole galaxy, and the conditions necessary for life to exist as a small one-foot wide target: unless the dart hits the target, life would be impossible. The fact that the dials are perfectly set, or the dart has hit the target, strongly suggests that someone set the dials or aimed the dart, for it seems enormously improbable that such a coincidence could have happened by chance.
3. The fine-tuning argument is probably the most powerful current argument for the existence of God.
J. Warner Wallace: FINE-TUNING OF THE FORCE STRENGTHS TO PERMIT LIFE AUGUST 3, 2014
The constants governing the four fundamental forces of physics must be finely tuned to support life. I now focus on the quantitative constraints on the strengths of these forces if intelligent life is to plausibly exist anywhere in the universe. First some background – physicists typically refer to coupling constants for those dimensionless constants which represent the strength of each force. The strength of these forces ranges over about 40 orders of magnitude – that is to say that the strongest force is 10^40 times stronger than the weakest force. Thus, it would be surprising if the strengths of these forces must lie in narrow ranges to permit life – at least if the values were set at random such as would be the case in a universe without God. Let’s look at how sensitive these parameters are with respect to permitting life:
Strong nuclear force
This force is important for the existence of stable atoms beyond hydrogen. If the strong force were 50% weaker, no elements used by life would exist because protons couldn’t be held together in the nucleus. The strong nuclear force must exceed the strength of the electromagnetic force sufficiently to overcome the electromagnetic repulsion of positively charged protons. While learning chemistry would be much easier if only the first few elements existed in the periodic table, there would be no physical creatures around to learn it! If the strong force were about 50% stronger no hydrogen would be left over from nuclear fusion processes occurring in the early universe. Hydrogen plays a critical life-supporting role not only as a constituent of water but hydrogen-burning stars last 30 times longer than alternatives. This particular constraint may not make intelligent life impossible but life would certainly be much harder to originate if the available time were so limited and if neither water nor hydrocarbons existed.
Also, hydrogen-bonding is very important in biology for many reasons: information storage in DNA, antibody-antigen interaction, and for the secondary structure of proteins. Remember that parameters that seem beneficial for life but are more fine-tuned than is strictly necessary counts against a multiverse explanation of the fine-tuning because multiverse scenarios predict only what is minimally necessary for life. An even tighter constraint is that if the strong force were more than about 2% stronger protons wouldn’t form from quarks – in which case no chemical elements would exist! If the strong force were 9% weaker, stars would be unable to synthesize any elements heavier than deuterium (which is heavy hydrogen).
Electromagnetic force
This force is responsible for chemistry and plays a critical role in stellar fusion which powers life. The electromagnetic force needs to be much weaker than the strong nuclear force for atoms to be stable – so that the radius of the electron orbit is much larger than the radius of the nucleus. Unless the electromagnetic coupling constant (which represents its strength) is less than about 0.2, there would be no stable atoms because electrons orbiting the nucleus would have enough kinetic energetic to create electron-positron pairs which would then annihilate each other and produce photons. Additional examples of fine-tuning for this force strength will be described later in this blog.
Weak nuclear force
The weak force controls proton-proton fusion, a reaction 1,000,000,000,000,000,000 times slower than the nuclear reaction based on the strong nuclear force. Without this, “essentially all the matter in the universe would have been burned to helium before the first galaxies” were formed. Because the weak nuclear force is so much weaker than the strong nuclear force, a star can “burn its hydrogen gently for billions of years instead of blowing up like a bomb.” I’ve previously described the negative ramifications for life if there were no hydrogen in the universe. John Leslie points out several other ways in which the weak nuclear force is finely-tuned. “Had the weak force been appreciably stronger then the Big Bang’s nuclear burning would have proceeded past helium and all the way to iron. Fusion-powered stars would then be impossible.” Neutrinos interact only via the weak force and are just powerful enough to blast off outer layers of exploding stars but and just weak enough to pass through parts of the star to get there. The weak force also plays a role in fusing electrons and protons into neutrons during the core collapse of stars to keep the collapse proceeding until it becomes an exploding star (supernova). UK Astronomer Royal Sir Martin Rees estimated that a change in the strength of the weak nuclear force by about 1 part in at least 10,000 relative to the strength of the strong force would have prevented supernova explosions which allow heavier elements to find their way to planets.[8] Without these supernova explosions key heavy elements would be unavailable for life.
Gravitational force
Many physicists think that we’ll eventually discover a Grand Unified Theory, uniting gravity with the other 3 fundamental forces. For this reason Stanford physicist Leonard Susskind remarks that “the properties of gravity, especially its strength, could easily have been different. In fact, it is an unexplained miracle that gravity is as weak as it is.[9]” This probable underlying relationship leads to a natural expectation that gravity could be as strong as the strongest force. The strength of gravity is about 40 orders of magnitude weaker than the strong nuclear force. Based on this expectation that gravity can vary up to strong nuclear force strength, the level of fine-tuning required for life is pretty remarkable: If gravity is weaker by 1 in 10^36, stars are unstable to degeneracy pressure (for small stars) or unstable to radiative pressure just expelling huge chunks of the star (for larger stars).If gravity is stronger by 1 in 10^40, the universe is dominated by black holes not stars.If gravity is weaker by 1 in 10^30, the largest planet that would avoid crushing effects of gravity on any large-brained creatures would have a radius of about 50 meters – which is not a good candidate for an ecosystem and the development/sustenance of intelligent life.
These are huge numbers that may be hard for most readers to visualize. Thus, consider the following analogy to help understand the improbability of 1 part in 10^36. Suppose one could make a sand pile encompassing all of Europe and Asia and up to 5 times the height of the moon. Suppose one grain of sand is painted red and randomly placed somewhere within this pile. A blind-folded person then randomly selects one grain of sand from the pile. The odds that she would select that one red grain of sand are slightly better than the 1 in 10^36 odds of a life-permitting strength of the gravitational force based on just one of the above criteria.
https://crossexamined.org/fine-tuning-force-strengths-permit-life/
Warner Wallace: Energy-Density is Finely-Tuned JULY 21, 2014
The amount of matter (or more precisely energy density) in our universe at the Big Bang turns out to be finely-tuned to about 1 part in 1055. In other words, to get a life-permitting universe the amount of mass would have to be set to a precision of 55 decimal places. This fine-tuning arises because of the sensitivity to the initial conditions of the universe – the life-permitting density now is certainly much more flexible! If the initial energy density would have been slightly larger, gravity would have quickly slowed the expansion and then caused the universe to collapse too quickly for life to form. Conversely if the density were a tad smaller, the universe would have expanded too quickly for galaxies, stars, or planets to form.
https://crossexamined.org/fine-tuning-initial-conditions-support-life/
G.Gonzalez and Jay W. Richards: THE PRIVILEGED PLANET, page 220:
Imagine a large, complicated device, adorned with scores of enormous dials not unlike the dials on a Master padlock. Every knob is inscribed with numbered lines. And above each knob are titles like
“Gravitational Force Constant,”
“Electromagnetic Force Constant,”
“Strong Nuclear Force Constant,”
“Weak Nuclear Force Constant.”
That is a Universe-Creating Machine. The machine has a viewing screen that allows previewing what different settings will produce before they Press Start. Only if each of the dials is set to the right combination will the machine produce a habitable universe. Most of the settings produce uninhabitable universes.
The dials must all be set very precisely, or the Universe-Creating Machine will spit out a universe that collapses within a few seconds into a single black hole or drifts along indefinitely as a lifeless hydrogenated soup.
How precisely do the knobs have to be set?
There is only ONE combination that actually produces a universe even mildly habitable—namely, our own. the universe, as described by its physical laws and constants, is be fine-tuned for the existence of life. In the nineteenth century, many scientists thought the universe and our existence had little or no fundamental connection, and that our existence was simply one of the events that happen in an infinite and eternal universe. The fine-tuning of the universe obviously contradicts this. When physicists say, for example, that gravity is “fine-tuned” for life, what they usually mean is that if the gravitational force had even a slightly different value, life would not have been possible.
If gravity were slightly weaker, the expansion after the Big Bang would have dispersed matter too rapidly, preventing the formation of galaxies, planets, and astronomers. If it were slightly stronger, the universe would have collapsed in on itself, retreating into oblivion like the groundhog returning to his hole on a wintry day. In either case, the universe would not be compatible with the sort of stable, ordered complexity required by living organisms. Specifically, physicists normally refer to the value of, say, gravity relative to other forces, like electromagnetism or the strong nuclear force. In this case, the ratio of gravity to electromagnetism must be just so if complex life as we know it is to exist. If we were just to pick these values at random, we would almost never find a combination compatible with life or anything like it. Given the prevailing assumptions of nineteenth- and twentieth-century science, discovering that the universe is fine-tuned was a surprise. Underlying the astonishment is the implication that the range of uninhabitable (theoretical) universes vastly exceeds the range of universes, like our own, that are hospitable to life. Thrown to the winds of chance, an uninhabitable universe is an astronomically more likely state of affairs.
CARBON AND OXYGEN, ACT II
But such fine-tuning isn’t limited to chemistry. In 1952–1953 Fred Hoyle discovered one of the most celebrated examples of fine-tuning in physics.8 In contemplating the required pathway for the production of carbon and oxygen in nuclear reactions in the hot interiors of red giant stars, Hoyle correctly predicted that carbon-12 must have a very specific nuclear energy resonance not known at the time.9 A nuclear resonance is a range of energies that greatly increases the chances of interaction between a nucleus and another particle—for example, the capture of a proton or a neutron. An energy resonance in a nucleus will accelerate reactions if the colliding particles have just the right kinetic energy. Resonances tend to be very narrow, so even very slight changes in their location would lead to enormous changes in the reaction rates. This may seem obscure but think of a wineglass shattering when just the right acoustic note is played. That’s a resonance. The relevant nuclear reactions occur in the stage of a star’s life following the hydrogen-burning main sequence, during so-called helium-shell burning. Fortunately for us, many moderate- to high-mass stars have already reached this stage and have seeded our galaxy with a healthy dose of carbon and oxygen. During this advanced, helium-shell burning stage, alpha particles (helium nuclei) abound in a star’s deep interior, creating frequent high-energy collisions. When two helium nuclei collide, they form an unstable beryllium-8 nucleus; even this is only possible because the mass of two helium nuclei is very close to that of the beryllium-8 nucleus. It remains bound long enough (just 10-16 seconds) to collide with another alpha particle to form carbon-12.10 But this result is not quite sufficient. Because it is effectively a three-body reaction, carbon-12 won’t be produced without a resonance.
It was the lack of a known resonance at the energy level required to produce carbon that led Hoyle to make his famous prediction. Since the universe contains plenty of carbon, Hoyle deduced that such a resonance must exist. Had the resonance been slightly lower, the universe would have far less carbon. In fact, the observed abundance of carbon and oxygen depends on a few other coincidences. It turns out that the lack of resonance in oxygen at the typical alpha particle energy in a star prevents all the carbon from being used up to make oxygen (thankfully, the closest resonance is just a little bit too low). But if the fine-tuning stopped there, the universe would have squandered most of its oxygen well before any star system had time even to think about hosting life. You see, certain conservation laws prevent easy capture of alpha particles by oxygen-16 to form neon-20, even though a resonance exists in neon-20 at just the right place. Otherwise, little oxygen would remain. As a result of these four astounding “coincidences,” stars produce carbon and oxygen in comparable amounts. Astrophysicists have recently confirmed the sensitivity of carbon and oxygen production to the carbon-energy resonance; a change in the (strong) nuclear force strength (the force that binds particles in an atomic nucleus) by more than about half a percent, or by 4 percent in the electromagnetic force (the force between charged particles), would yield a universe with either too much carbon compared with oxygen or vice versa, and thus little if any chance for life. Including the other three required fine-tunings further narrows this range.
The strengths of the “fundamental forces”—the gravitational, strong nuclear, weak nuclear, and electromagnetic (these last two now often combined into one force called electroweak)—are perhaps the most popular examples of fine-tuning. These forces affect virtually everything in the cosmos. And like those individual dials on the Universe-Creating Machine, each one must take a narrow value to render a life-friendly universe.
The Interactions and Their Strengths
In nuclear physics and particle physics, the strong interaction is one of the four known fundamental interactions, with the others being electromagnetism, the weak interaction, and gravitation. At the range of 10−15 m (1 femtometer), the strong force is approximately 137 times as strong as electromagnetism, 106 times as strong as the weak interaction, and 1038 times as strong as gravitation.
https://en.wikipedia.org/wiki/Strong_interaction
The strengths or coupling constants of the strong, weak, and electromagnetic interactions are fundamental parameters of the Standard Model of particle physics.
In physics, a coupling constant or gauge coupling parameter (or, more simply, a coupling), is a number that determines the strength of the force exerted in an interaction. Originally, the coupling constant related the force acting between two static bodies to the "charges" of the bodies (i.e. the electric charge for electrostatic and the mass for Newton's gravity) divided by the distance squared, {\displaystyle r^{2}}
They depend on energy and in Grand Unified Theories converge to a common value. Feynman once commented on the famous value of the fine structure constant α = e2/4π = 1/137 of quantum electrodynamics: “There is a most profound and beautiful question associated with the observed coupling constant, e—the amplitude for a real electron to emit or absorb a real photon . . . It’s one of the greatest damn mysteries of physics: a magic number that comes to us with no understanding by man. You might say the ‘hand of God’ wrote that number, and ‘we don’t know how He pushed his pencil’. We know what kind of a dance to do experimentally to measure this number very accurately, but we don’t know what kind of dance to do on the computer to make this number come out, without putting it in secretly!”
Gravity:
How finely tuned is gravity? -- Well, the strength of gravity could be at any one of 14 billion billion billion settings, but there is only one setting which is adequate (and optimal) for a universe with intelligent life to exist.
-- To illustrate: This is as if you had a measuring tape with one-inch sections stretched across the known universe, it would be 14 billion billion billion inches long, and only one or two of those inches in the middle is the optimal strength setting for gravity. If you moved the strength setting to the right or left just a couple of inches, then intelligent life could not exist (though bacterial life might survive with gravity stronger or weaker by one setting up or down).
THE PROBABILITY: Although the force of gravity could obviously have attained a large number of wrong magnitude-ranges, the chance of it being correct for intelligent life to exist, is one chance out of 14 billion billion billion. --Thus, we can conservatively say that it was about one chance out of 1,000,000,000,000,000,000,000 (or 1 out of 10^21, or 1 out of a billion trillion) that the force of gravity might have randomly attained such an advantageous strength for the making of life-necessary elements in the stars.
Imagine stretching a measuring tape across the entire known universe. Now imagine one particular mark on the tape represents the correct degree of gravitational force required to create the universe we have. If this mark were moved more than an inch from its location (on a measuring tape spanning the entire universe), the altered gravitational force would prevent our universe from coming into existence
The Strong Nuclear Force
If the strong nuclear force were very slightly weaker by just one part in 10,000 billion billion billion billion, then protons and neutrons would not stick together, and the only element possible in the universe, would be hydrogen only. There would be no stars and no planets or life in the universe. (Ref., Dr. Robin Collins of Messiah College).
However, if the strong nuclear force were slightly too strong by the same fraction amount, the protons and neutrons would tend to stick together so much that there would basically only be heavy elements, but no hydrogen at all --If this were the case, then life would also not be possible, because hydrogen is a key element in water and in all life-chemistry.
THE PROBABILITY: If the strong nuclear force were slightly weaker or stronger than it in fact is, then life would be impossible. Therefore, we can very conservatively say that it was about one chance out of 1,000,000,000,000 (1 out of a trillion) that the strong nuclear force might have randomly possessed the correct strength to make life possible in our cosmos.
The Weak Nuclear Force
Our hydrogen-burning Sun consumes its nuclear fuel more than one hundred times more slowly than a pure helium star of comparable mass. A helium star of an appropriate mass wouldn’t last nearly long enough for life to develop. Not that life would ever develop around such a star anyway: it would contain no water or organic compounds, making the formation of life on any timescale impossible. It’s not even clear that stars could form from contracting clouds of gas in a universe of pure helium. Unlike hydrogen, helium does not form molecules, which are the primary means by which dense interstellar clouds cool, and thereby contract to form stars. 2
The weak nuclear force is what controls the rates at which radioactive elements decay. If this force were slightly stronger, the matter would decay into the heavy elements in a relatively short time. However, if it were significantly weaker, all matter would almost totally exist in the form of the lightest elements, especially hydrogen and helium ---there would be (for example) virtually no oxygen, carbon or nitrogen, which are essential for life.
In addition, although heavier elements necessary for life are formed inside giant stars, those elements can only escape the cores of those stars when they explode in supernova explosions, however, such supernova explosions can only occur because the weak nuclear force is exactly the right value. As Professor of astronomy, Paul Davies, describes this situation: "If the weak interaction were slightly weaker, the neutrinos would not be able to exert enough pressure on the outer envelope of the star to cause the supernova explosion. On the other hand, if it were slightly stronger, the neutrinos would be trapped inside the core, and rendered impotent" (My emphasis.) (ref. P.C.W. Davies, The Accidental Universe, London, 1982, p.68.)
THE PROBABILITY: Considering the fine-tuning of the weak nuclear force for both the rate of radioactive decay as well as the precise value required to allow supernova explosions, it is probably conservative to say that it was one chance out of 1000 that the weak nuclear force was at the right strength to permit these processes so that life would be possible.
The Electromagnetic Force
Electrons are bound by the electromagnetic force to atomic nuclei, and their orbital shapes and their influence on nearby atoms with their electrons is described by quantum mechanics. The electromagnetic force governs the processes involved in chemistry, which arise from interactions between the electrons of neighboring atoms. 1
If the electromagnetic force (exerted by electrons) were somewhat stronger, electrons would adhere to atoms so tightly that atoms would not share their electrons with each other ---and the sharing of electrons between atoms is what makes chemical bonding possible so that atoms can combine into molecules (e.g., water) so that life can exist. However, if the electromagnetic force were somewhat weaker, then atoms would not hang onto electrons enough to cause any bonding between atoms, and thus, compounds would never hold together. In addition, this fine-tuning of the electromagnetic force must be even more stringent if more and more elements are to be able to bond together into many different types of molecules.
THE PROBABILITY: Considering the range of electromagnetic force that might have occurred, it is reasonable to say that the probability of the electromagnetic force being balanced at the right level for many thousands of compounds to function for the making of chemical compounds necessary for life, is one chance out of 1000.
So, the atom symbol would be well appropriated to be used by proponents of intelligent design - and not atheists.
1. https://crossexamined.org/fine-tuning-force-strengths-permit-life/
http://www.pbs.org/wgbh/nova/physics/blog/2012/03/is-the-universe-fine-tuned-for-life/
Hyperphysics, fundamental forces
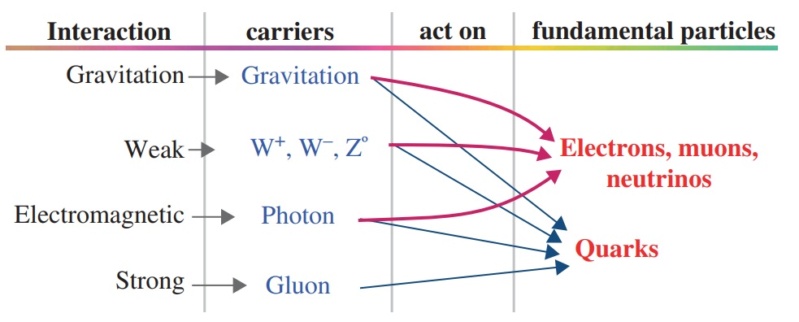

Last edited by Otangelo on Fri Oct 29, 2021 8:09 am; edited 30 times in total